Evaluation of the impact of reference tooth morphology and alignment on model measurement accuracy
Introduction
Accurate measurement and model analysis are critical steps to achieving good outcomes in clinical dental treatment especially for orthodontics, prosthodontics, orthognathic surgery, and oral implantology. The plaster model has commonly been preferred, and comprised the long-standing research standard (1-3). However, compared with the later digital models, the characteristics of long-term storage reliability, space occupation, and fragility of the plaster model have become obvious disadvantages. Currently, dental clinics prefer to use digital models as an alternative (4-6).
Digital models can be obtained in three ways: by instrumental scanning of the plaster model and the patient’s intraoral space or by taking a cone beam computed tomography (CBCT) scan. However, non-radiographic scans are the most commonly used in clinical practice (7). Previous studies have demonstrated that the digital model obtained via non-radiographic scanning is an acceptable clinical substitute for either a cast or an intraoral scan. Nevertheless, digital models also have disadvantages such as extra hardware costs for clinics, and the increased likelihood of activating patient vomiting reflexes during intraoral scans. Further, for the results of the scans, there is also a risk of arch curvature distortion for a full arch or more than half of an arch length (6,8-10).
The use of digital models is relatively widespread for existing personalized dental treatments in the context of precision medicine, such as personalized orthodontic appliances, dental prostheses, and occlusal collocation involving orthognathic surgery (11,12). Tooth width and arch length, as the basic elements of model measurement and analysis, can be obtained with the help of various digital tools for a more detailed and comprehensive observation, but the update of tools for clinical diagnosis and treatment has not been accompanied by a change in measurement methods. The measurement of digital models only transfers the contact measurement method on the original plaster model to the screen and presents it in a non-contact form (13). Although existing studies have explored the substitutability of digital models from different data sources, and the validity of model measurements, few studies have focused on the effect of a comprehensive view of the three-dimensional (3D) tooth structure on measurement accuracy (7,14,15). No studies have analyzed and compared the effect of tooth axis direction and tooth alignment on measurement results. This study sheds new light on the effect of tooth axis direction and tooth alignment on tooth width and arch length measurement results with the aim of improving the accuracy of dental clinical model analysis. We present the following article in accordance with the MDAR reporting checklist (available at https://atm.amegroups.com/article/view/10.21037/atm-22-2497/rc).
Methods
Inclusion criteria and grouping
The study population consisted of 80 patients who received orthodontic diagnosis and treatment at the Hospital of Stomatology, Jilin University from January 2019 to January 2020. The study was conducted in accordance with the Declaration of Helsinki (as revised in 2013). The study was approved by the Medical Ethics Committee of Hospital of Stomatology, Jilin University (No. 2020041), and all participants provided their informed consent. The number of specimens required for this study was estimated using a significance level of α=0.05, 95% power and an effect size of 0.80 at G power (version 3.1, Franx Faul. Universitat Kiel, Germany). The sample consisted of 27 males and 53 females, and the mean age of males and females was 23.6 and 22.8 years, respectively. The inclusion criteria were permanent dentition; patients underwent orthodontic treatment and completed alignment; clear adjacent relationships between teeth; no maxillofacial deformities; no malocclusion; and no interproximal reduction (IPR) operation or other alterations of tooth morphology during orthodontic treatment.
The study population was divided into two groups to investigate the influence of the calibrated tooth axis and tooth arrangement on tooth width measurement, respectively.
Group 1 included 50 patients who had completed tooth alignment, and at the time there was a clear contact between adjacent teeth. A total of 50 sets of plaster casts of aligned dentition were prepared by alginate impressions. To obtain accurate tooth axis information, radioactive CBCT scans were used to provide aligned root morphology.
Group 2 included 30 patients who received orthodontic treatment, for whom plaster casts were prepared by alginate impressions at first visit and after tooth alignment, respectively, and CBCT at first visit was conducted to provide exact root morphology information. A total of 60 dentition models were obtained.
All records collected were part of the standard records of orthodontic treatment, involving radiological examinations to evaluate whether there was an abnormality of the temporomandibular joint or routine preoperative examination of orthognathic surgery. To control radiation intake, no more than two CBCT scans were performed on each individual patient.
Scanning and data processing
The operator was sufficiently experienced in scanning to perform a non-destructive optical scan of the collected plaster casts under the same conditions using the 3Shape® E-1™ model scanner (3Shape, Copenhagen, Denmark) according to the manufacturer’s operating instructions. The obtained digital images are stored in standard tessellation language (STL) format through the supporting software.
To obtain more thorough root morphology and axis information of the tooth as a whole, CBCT scanning was performed. The CBCT images were imported into mimics 17.0 software (Materialise NV, Leuven, Belgium) in digital imaging and communications in medicine (DICOM) format. The CBCT images were preliminarily processed, and the anatomical morphology of the teeth, including the root, was extracted from the whole head image by threshold segmentation and region growth. The dentition data from the same patient was registered with the CBCT extraction data in the Geomagic Studio software (3D Systems, Rock Hill, SC, USA), and Boolean-synthesized into a digital tooth model with roots. The obtained synthetic model was guaranteed to be the closest to the real anatomy by manually annotated and most recent iterations of two registrations. During data processing, regional restoration and smoothing operations without affecting the proximal-distal and axis calibration of the teeth were necessary to obtain a good appearance. All processed models were stored in STL format (Figure 1).
Measurement
Measurements were performed in 3Shape® Ortho Analyzer™ software (3Shape, Copenhagen, Denmark) by an orthodontic specialist fully trained in the method following the reference procedure provided by the manufacturer. Measurements consisted of a portion of the aligned dentition and a portion of the pre- and post-treatment dentition from the same patients. At the same time, to examine the influence of the calibrated tooth axis on the measurement results, different measurement methods were used for the conventional dentition model and the synthetic model. On the conventional model, in addition to the most common direct calibration of the proximal and distal teeth (Method A), the expert also calibrated the axis of the clinical crown to provide a reference for the calibration of the proximal and distal positions (Method B). For the synthetic model, the anatomical shape of the root was coherently combined with the crown, and the overall axis of the tooth could be calibrated by the expert to provide a relatively more comprehensive reference for the proximal and distal calibration of the crown (Method C) (Figure 2).
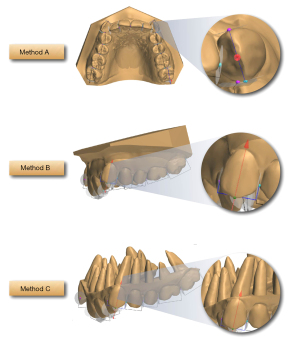
Here, Method A is actually the method of measuring plaster models. Clinical dentists usually use vernier calipers to mark the proximal and distal ends of the teeth. It is a contact measurement method. It is also used in many studies involving digital model measurement. Although the operation object becomes a digital model, it is still limited to marking the proximal and distal positions. Tooth width is usually measured as the longest proximal and distal width parallel to the occlusal plane, so the conventional field of view is also dominated by the occlusal plane, and information about the tooth axis or other 3D features of the tooth is not taken seriously. However, the contact position between adjacent teeth is often limited by the arrangement. Therefore, Methods B and C assist the surveyor in the observation and calibration of the proximal and distal positions by referring to the axis direction of the clinical crown and the entire tooth.
The core line length of each arch was also measured on the diagnostic model of Group 2 to analyze the arch length discrepancy (ALD), which is the difference between the expected arch length and the actual arch length. The core line is an artificial line that passes through most of the tooth contact area and conforms to the shape of the dental arch (16,17). It is used as a baseline to measure the degree of mismatch between the size of the teeth and the size of the dental arch. Except for the obvious buccolingual dislocation of the crown, the core line of the upper dental arch is generally the line connecting the central fossa of the posterior teeth, and the core line of the lower dental arch is generally the line connecting the buccal cusps of the posterior teeth. The length of the core line can be regarded as the existing length of the dental arch, and the sum of the widths of all teeth in the dental arch is regarded as the expected arch length of the dental arch. To minimize the influence of the arch deformation caused by the full arch scan, the existing segmental measurement method of the arch length was not selected.
Statistics
The collected data were processed with the software SPSS 25.0 (IBM SPSS Inc., Armonk, NY, USA). The analysis of variance (ANOVA)-test and paired t-test were used to evaluate the difference in measurement results between the two groups of samples by different measurement methods and different tooth arrangements. Measurements of tooth width, arch length, and subregional arch length were also evaluated. For Group 2, the ALD of each newly diagnosed model was calculated according to the length of the core line, and its correlation with the measurement results was evaluated by the Pearson correlation coefficient (CC). When P<0.05, the absolute value of CC was 0.1–0.3, 0.3–0.5, and >0.5, and it was considered that there was a weak correlation, moderate correlation, and strong correlation between variables. For ALD, when it was positive, it indicated that there was crowding in the dentition, and vice versa, it indicated that there was additional space in the dentition.
Results
Group 1 result
Results using the three different measurements on the model in Group 1 did not show a difference, either for full arch length or independent tooth width. The ANOVA-test yielded P>0.05 in all comparisons of measurements (Table 1). This indicated that on the aligned model, whether the tooth axis (clinical crown axis or tooth 3D axis) was marked when tooth width measurement or full dental arch measurement was performed, the measurement results obtained would not be statistically significantly different.
Table 1
Width and length | F | P value |
---|---|---|
#17 | 0.011 | 0.989 |
#16 | 0.063 | 0.939 |
#15 | 0.001 | 0.999 |
#14 | 0.015 | 0.985 |
#13 | 0.001 | 0.999 |
#12 | 0.003 | 0.997 |
#11 | 0.002 | 0.998 |
#21 | 0.008 | 0.992 |
#22 | 0.007 | 0.993 |
#23 | 0.056 | 0.946 |
#24 | 0.004 | 0.996 |
#25 | 0.008 | 0.992 |
#26 | 0.009 | 0.991 |
#27 | 0.034 | 0.967 |
UAL | 0.000 | 1.000 |
#37 | 0.019 | 0.982 |
#36 | 0.002 | 0.998 |
#35 | 0.003 | 0.997 |
#34 | 0.02 | 0.98 |
#33 | 0.072 | 0.931 |
#32 | 0.011 | 0.989 |
#31 | 0.02 | 0.98 |
#41 | 1.004 | 0.369 |
#42 | 0.003 | 0.997 |
#43 | 0.03 | 0.97 |
#44 | 0.022 | 0.978 |
#45 | 0.000 | 1.000 |
#46 | 0.003 | 0.997 |
#47 | 0.005 | 0.995 |
LAL | 0.556 | 0.575 |
ANOVA-test, unit = mm. UAL, upper (maxilla) full arch length; LAL, lower (mandible) full arch length; F, F value of analysis of variance; ANOVA, analysis of variance.
Group 2 result
For Method A, except for the left lower first molar and the central incisor, the measurement results of tooth width and proper arch length all showed differences, and the average errors were all shown as negative values (Table 2). The largest error occurred in the upper left second molar and the lower right second molar, both exceeding 0.4 mm. The average error of the arch length also exceeded 2 mm, the maxillary error range was −3.911 to −0.965 mm, and the mandibular error range was −4.069 to −0.149 mm. For Method B, only the maxillary incisor, left upper cusp, right second molar, and maxillary arch length showed differences in measurement results, while the other teeth and mandibular arch length showed similar results. The average error range of tooth width measurement was −0.005 to 0.053 mm, and only the lower left first molar showed a positive value. The error ranges of arch length were −2.492 to 0.488 and −2.744 to −0.464 mm, respectively. For Method C, although there were differences in the measurement results of the maxillary incisor, the right upper canine, and the left upper first molar, there were no differences in the measurement results of the maxillary residual tooth width and the width of all the mandibular teeth, as well as the maxillary and maxillary arch length. The average error of tooth width ranged from −0.002 to 0.076 mm, with positive values appearing in molars, premolars, and incisors. The error range of the expected arch length was reduced to −1.740 to 0.986 and −2.213 to 1.619 mm. With the use of different methods for the calibration of the tooth axis, the measurement results of tooth width and dental arch length gradually transitioned from difference to no difference, especially the measurement of the mandible. At the same time, the range of measurement error was gradually decreasing, and there was a change from negative value to positive value, which can be clearly seen from the measurement of the length of the dental arch, whether it is the maxilla or mandible.
Table 2
Width and length | Method A | Method B | Method C | ||||||||
---|---|---|---|---|---|---|---|---|---|---|---|
Mean | SD | P value | Mean | SD | P value | Mean | SD | P value | |||
Maxilla | |||||||||||
#17 | −0.375 | 0.491 | 0.013* | −0.095 | 0.402 | 0.393 | 0.028 | 0.407 | 0.8 | ||
#16 | −0.227 | 0.307 | 0.000*** | −0.211 | 0.635 | 0.084 | −0.060 | 0.306 | 0.302 | ||
#15 | −0.207 | 0.263 | 0.001** | −0.037 | 0.229 | 0.434 | −0.028 | 0.258 | 0.593 | ||
#14 | −0.195 | 0.171 | 0.000*** | −0.082 | 0.174 | 0.069 | −0.078 | 0.172 | 0.078 | ||
#13 | −0.200 | 0.223 | 0.000*** | −0.104 | 0.219 | 0.017* | −0.099 | 0.236 | 0.033* | ||
#12 | −0.104 | 0.187 | 0.005** | −0.005 | 0.178 | 0.876 | 0.03 | 0.152 | 0.286 | ||
#11 | −0.217 | 0.139 | 0.000*** | −0.106 | 0.152 | 0.001** | −0.080 | 0.145 | 0.005** | ||
#21 | −0.228 | 0.196 | 0.000*** | −0.137 | 0.179 | 0.000*** | −0.085 | 0.138 | 0.002** | ||
#22 | −0.133 | 0.154 | 0.000*** | −0.058 | 0.164 | 0.064 | 0.005 | 0.162 | 0.873 | ||
#23 | −0.178 | 0.16 | 0.000*** | −0.049 | 0.158 | 0.104 | −0.047 | 0.145 | 0.093 | ||
#24 | −0.242 | 0.13 | 0.000*** | −0.084 | 0.163 | 0.05 | −0.097 | 0.145 | 0.014* | ||
#25 | −0.174 | 0.178 | 0.000*** | −0.036 | 0.213 | 0.407 | 0.01 | 0.276 | 0.863 | ||
#26 | −0.203 | 0.245 | 0.000*** | −0.089 | 0.288 | 0.109 | −0.039 | 0.275 | 0.45 | ||
#27 | −0.438 | 0.611 | 0.012* | −0.111 | 0.565 | 0.444 | −0.002 | 0.496 | 0.989 | ||
UAL | −2.438 | 1.473 | 0.000*** | −1.002 | 1.49 | 0.001** | −0.377 | 1.363 | 0.141 | ||
Mandible | |||||||||||
#37 | −0.368 | 0.599 | 0.015* | −0.183 | 0.587 | 0.191 | −0.081 | 0.594 | 0.56 | ||
#36 | −0.124 | 0.402 | 0.114 | 0.053 | 0.399 | 0.488 | 0.074 | 0.364 | 0.293 | ||
#35 | −0.224 | 0.22 | 0.000*** | −0.080 | 0.227 | 0.073 | −0.062 | 0.217 | 0.143 | ||
#34 | −0.166 | 0.25 | 0.022* | −0.026 | 0.248 | 0.691 | −0.034 | 0.265 | 0.632 | ||
#33 | −0.138 | 0.217 | 0.002** | −0.036 | 0.225 | 0.388 | −0.046 | 0.229 | 0.285 | ||
#32 | −0.070 | 0.164 | 0.03* | −0.021 | 0.161 | 0.494 | 0.003 | 0.15 | 0.925 | ||
#31 | −0.063 | 0.184 | 0.07 | −0.014 | 0.183 | 0.68 | −0.018 | 0.18 | 0.579 | ||
#41 | −0.106 | 0.193 | 0.005** | −0.071 | 0.202 | 0.063 | −0.055 | 0.213 | 0.171 | ||
#42 | −0.116 | 0.174 | 0.001** | −0.065 | 0.189 | 0.078 | −0.040 | 0.192 | 0.277 | ||
#43 | −0.105 | 0.23 | 0.018* | −0.015 | 0.225 | 0.714 | −0.008 | 0.229 | 0.843 | ||
#44 | −0.155 | 0.25 | 0.022* | −0.015 | 0.265 | 0.815 | −0.005 | 0.269 | 0.939 | ||
#45 | −0.217 | 0.273 | 0.000*** | −0.036 | 0.282 | 0.52 | −0.026 | 0.26 | 0.61 | ||
#46 | −0.244 | 0.435 | 0.007** | 0.02 | 0.491 | 0.833 | 0.076 | 0.437 | 0.373 | ||
#47 | −0.609 | 0.599 | 0.000*** | −0.326 | 0.532 | 0.016* | −0.191 | 0.522 | 0.128 | ||
LAL | −2.109 | 1.96 | 0.000*** | −0.600 | 2.144 | 0.136 | −0.297 | 1.916 | 0.403 |
Paired t-test (*, P<0.05; **, P<0.01; ***, P<0.001, unit = mm). UAL, upper (maxilla) full arch length; LAL, lower (mandible) full arch length; SD, standard deviation.
In the comparison of measurement results in different regions, Method A still showed differences in the measurement results of various regions of the dental arch (Table 3). The difference in the results of Method B appeared in the upper dentition, including the anterior, middle, and posterior segments. The difference in the results of Method C only appeared in the maxillary anterior region. As for the average error, only the measurement of the middle part of the maxillary dental arch group B showed a positive value, and the average error of the mandibular measurement was generally larger than that of the maxilla. The measurement error range between the different methods exhibited an insignificant trend of decreasing gradually. The areas with the largest error range were from the maxillary premolar area of the Method C group, which was −1.444 to 1.764 mm, and the mandibular molar area of the Method B group, which was −1.371 to 0.861 mm.
Table 3
Length | Method A | Method B | Method C | ||||||||
---|---|---|---|---|---|---|---|---|---|---|---|
Mean | SD | P value | Mean | SD | P value | Mean | SD | P value | |||
Maxilla | |||||||||||
AA | −1.048 | 0.742 | 0.000*** | −0.323 | 0.648 | 0.011* | −0.27 | 0.536 | 0.01* | ||
PA | −0.566 | 0.345 | 0.000*** | −0.154 | 0.367 | 0.029* | 0.16 | 1.604 | 0.588 | ||
MA | −0.824 | 0.927 | 0.000*** | −0.393 | 0.898 | 0.023* | −0.083 | 0.727 | 0.536 | ||
Mandible | |||||||||||
AA | −0.588 | 0.86 | 0.001** | −0.217 | 0.849 | 0.171 | −0.162 | 0.791 | 0.271 | ||
PA | −0.567 | 0.524 | 0.000*** | −0.128 | 0.575 | 0.233 | −0.1 | 0.545 | 0.322 | ||
MA | −0.954 | 1.111 | 0.000*** | −0.255 | 1.116 | 0.222 | −0.035 | 1.026 | 0.854 |
Paired t-test (*, P<0.05; **, P<0.01; ***, P<0.001, unit = mm). AA, anterior area; PA, premolar area; MA, molar area; SD, standard deviation.
Measurements of the length of the core line allow the analysis of differences in the length of the dentition within the sample. The dentition length difference in the maxilla ranged from −4.52 to 14.91 mm, and the dentition length difference in the mandible ranged from −2.98 to 14.60 mm. When ALD gradually increased, the difference between the measured values of different methods on the first visit model and the measured values of the aligned model was mostly concentrated in the range of about 2 mm. From the corresponding regression lines, it can be seen that the difference results of Method A were the largest in both maxillary and mandibular measurements, while Method C had the smallest and most concentrated results, and most of the negative values came from Method C (Figures 3,4). In the corresponding correlation analysis results, the difference value of maxillary measurement Group C and mandibular measurement Group A showed moderate correlation with the difference of dental arch length, while the difference value of mandibular measurement Group C showed a strong correlation with the difference of dental arch length (Table 4).
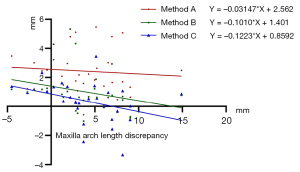
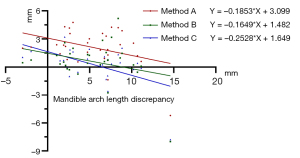
Table 4
Correlation | DEAL-A | DEAL-B | DEAL-C |
---|---|---|---|
UALD | |||
CC | −0.087 | −0.278 | −0.368* |
P value | 0.646 | 0.136 | 0.046 |
LALD | |||
CC | −0.371* | −0.302 | −0.518** |
P value | 0.044 | 0.105 | 0.003 |
Correlation analysis (*, weak correlation; **, moderate correlation). ALD, arch length discrepancy; UALD, upper (maxilla) arch length discrepancy; CC, correlation coefficient; LALD, lower (mandible) arch length discrepancy; DEAL-A, B, C, difference in expected arch length of Method A, B & C.
Discussion
Three different methods were used to measure tooth widths and arch lengths of 50 aligned models from 50 patients and 60 dentition models from 30 patients before and after orthodontic treatment. The effects of different degrees of axis calibration and dentition alignment on the measurement results during model measurement were evaluated. For the consideration of reducing radiation exposure, the models in this study were derived from two groups of patient samples.
For the alignment model of Group 1, the measurement of individual tooth width and arch length using three different methods showed no difference. In other words, when measuring the aligned dentition, it is not necessary to consider the influence of the axis of the teeth, and it is feasible to directly mark the proximal and distal positions, which will not produce statistical differences in the measurement results. Based on this result, the measurements on the aligned model were used as a reference standard for comparison with the measurements on the unaligned model in the analysis of the results of Group 2. At this point, the calibration of the axis began to affect the measurement results. The measurement results of Method A differed from the reference values except for individual teeth. This means that the most accurate measurement results cannot be obtained by mere proximal and distal positioning for the measurement of models with unaligned teeth that are common in clinical practice. Moreover, with the increase of the studied dental arch area or distance, the accumulation of errors also became larger, making the results less reliable. Due to the calibration of the axis of the teeth in Methods B and C, the difference in results began to be reduced and eliminated, and the error range of a single tooth was also slightly reduced. Although the differences between individual teeth in the upper jaw still existed, the measurement of the dental arch length was no different from the results on the aligned model, and the standard deviation was 1.363 mm (maxilla) and 1.916 mm (mandible), which was the smallest among the three methods. It can be seen that the accuracy of the measurement results of the traditional Method a applied to the plaster model is inferior to that of the Methods b and c. In contrast, Methods b and c refer to the overall structure of the tooth to assist in marking the proximal and distal positions of the tooth to obtain more accurate tooth width results. But their disadvantage lies in the need for more complex software operations. Furthermore, the use of method c also requires image data from CBCT to build a synthetic model.
Digital models, or related virtual patient setups, are an in vitro surrogate for human anatomy like traditional plaster casts (18,19). The proviso that they can be used as clinical tools is that the accuracy and efficiency of measurement can meet the requirements of certain clinical work, and can improve the efficiency of diagnostic and treatment and obtain better results. At present, a large number of studies have confirmed that digital models from various sources can show the same reliability as plaster models in terms of measurement reliability and model analysis results (20,21). Park et al. (8) compared the tooth width, dental arch length, and dental arch length differences in digital models obtained from plaster models, model scanners, and intraoral scanners, and found that the measurement of digital models has high validity, repeatability and accuracy. This is similar to the conclusions obtained by Malik et al. (22) using the reference model. At the same time, they and some other studies involving full dental arch scanning also paid attention to a phenomenon, that is, when the optical scanning range exceeds half of the full length of the dental arch, the shape of the dental arch tends to be distorted in curvature, especially for intraoral scans, the risk of arcuate distortion is relatively greater (23). However, in such studies comparing the substitutability of digital models from different sources relative to traditional models in terms of measurement and analysis, many scholars draw conclusions on the premise that different degrees of differential results are considered clinically acceptable. This may be related to the fact that the accuracy requirements for digital models in orthodontic clinics have not yet reached the same standard (9). From the perspective of precision medicine, higher accuracy requirements and standards are still recommended, so as to give full play to the advantages of digital technology and obtain good results.
The substitutability of digital models relative to plaster models has been demonstrated in many studies, whether the data are derived from intraoral scans or physical model scans. The measurement method of the digital model does not seem to have changed with the update of the tools. Most of the studies involving digital model measurement have still seemed to use a similar contact method, that is, direct calibration of the proximal and distal teeth, ignoring the correction of the results by the axis of the teeth or other 3D features of the teeth (24-26). The results of tooth width measurement will be affected by the degree of tooth inclination, and simply marking the proximal and distal ends may cause a large bias in the measurement results, especially for inexperienced or untrained operators (27,28). Small differences in measurement results have generally been considered clinically insignificant, perhaps due to differing requirements for precision or clinical needs, although these conclusions have long been reported (4,9). However, the precision requirements of dental practice are higher than that of the era of using plaster models under the background of digital technology, and new diagnostic and treatment methods and tools will be accompanied by the improvement of treatment goals. More precise and personalized treatment should become the new pursuit (29,30). Taking orthodontic treatment as an example, when using clear appliances for personalized treatment, IPR is a very common treatment method. Its design and clinical operation are often carried out within the range of 0.1 to 0.5 mm. If the models’ measurement error cannot be controlled within this range, then the IPR work seems to have lost its clinical significance (31,32).
In this study, we also took the dentition alignment as one of the evaluation objects to discuss its influence on the measurement results. From the results of statistical analysis, the mean error of measurement on the unaligned dentition was almost always negative, which means that the measured value of tooth width was always relatively small. The same was true for measurements with reference to the axis. During model analysis, length measurements can be affected by factors such as tooth adjacency, intraoral appliances, and soft tissue. For the unaligned dentition, the twist, slant, and incomplete eruption of the teeth will directly increase the occlusion of the proximal and distal ends, thus affecting the operator’s observation (24). However, in the model of Group 2, as the ALD increased, the differences in measurement were mostly concentrated in the range of about 2 mm. Furthermore, Method C showed the smallest difference and the most concentrated distribution in both the maxilla and mandible. Correlation analysis also showed that the differences in results caused by the three measurement methods were not all related to ALD, and the statistically significant results only appeared in the maxillary Method C (P<0.05, CC =0.368) and mandibular Method A and C groups (P<0.05, CC =−0.371; P<0.01, CC =−0.518). With the increase of the ALD, the unaligned teeth will not evenly appear in all areas of the dentition, but remain concentrated in a certain area. In this instance, severely ectopic teeth tend to appear outside the arc of the dental arch, resulting in a more obvious exposed proximal surface. Dentition crowding often occurs in the anterior teeth area, and the crowding degree in the middle and rear of the dental arch is less, and the situation is often represented by the buccal and lingual exposure, relatively speaking, this cannot cause much exposure to the proximal and distal teeth (33,34). It can also be seen from the differences in the measurement results of different regions within the dentition that the anterior teeth, especially the maxilla, are most prone to deviations in the measurement results. In the comparison of mandible and maxilla, the error of mandibular measurement results is often higher than that of maxillary, and the average width of mandibular anterior teeth is smaller than that of maxillary anterior teeth, which may be the main reason for this problem.
From the perspective of tooth anatomy, in the direction from the mesial to the distal of the dentition, the area of the interproximal contact area of the anterior teeth, premolars, and molars gradually increases (35). Therefore, in the process of tooth width measurement, when calibrating the proximal and distal ends, the same degree of calibration deviation will bring greater errors to teeth with relatively smaller contact areas of the proximal surfaces. For molars with a larger interproximal contact area, the measurement error caused by unilateral or bilateral marking deviation is smaller. Similarly, the aspect ratio of the buccal-lingual morphology of the teeth gradually increases in this direction, which will lead to the gradual reduction of the influence of the deviation of the angle on the measurement results when the axis of the teeth is calibrated. The deviation angle of the same tooth axis calibration has a greater impact on the anterior teeth than the molars.
In summary, the crowding of the dentition and the shape of the teeth may cause errors in the model measurement process, because both of them will affect the accuracy of the proximal and distal markings of the teeth, thereby affecting the accuracy of the model measurement. In clinical practice, the object of model analysis by dentists is often the unaligned dentition in most cases. A single measurement method that marks the proximal and distal ends of the teeth may not yield very accurate data results. Especially when the precision of the treatment is high, this approach is not recommended. In clinical practice, Method c can obtain the most accurate model measurement results, compared with the other two methods. Although the measurement method considering the overall 3D axis of the tooth obtained the most accurate results in this study, it requires data from CBCT scans to perform registration reconstruction of the 3D digital model to obtain the complete tooth structure. However, this will increase the patient’s additional radiation intake, especially if there is no clear need for CBCT scanning at the first visit, such as evaluation of temporomandibular joint status, alveolar bone defects, or severe craniomaxillofacial deformities. Therefore, the measurement method referring to the axis of the clinical crown is the most recommended method when CBCT data is not available which can obtain a relatively accurate model measurement result with less error in a convenient model acquisition method. It is worth mentioning that the measurements taken at this time are often smaller than the true size.
Conclusions
When the digital dental model is measured for the unaligned dentition, more accurate measurement results can be obtained by referring to the long axis of the teeth to help the width calibration, but this rule does not apply to the aligned dentition. Clinicians should be mindful that the measurement results are undersized relative to the actual size when using the digital model for diagnosis and analysis, especially when measuring the maxillary dentition. Part of the error in the model measurement comes from the different crowding conditions of the dentition and the anatomical characteristics of the teeth. Considering the overall 3D shape of the teeth will help to obtain more accurate model analysis results. Clinicians should choose an appropriate measurement method according to the actual treatment requirements.
Acknowledgments
Funding: This work was supported by National Natural Science Foundation of China (Nos. 81801005 and 82170994), Health Commission of Jilin Province (No. 2018-33-05), and Natural Science Foundation of Jilin Province (No. 20200201344JC).
Footnote
Reporting Checklist: The authors have completed the MDAR reporting checklist. Available at https://atm.amegroups.com/article/view/10.21037/atm-22-2497/rc
Data Sharing Statement: Available at https://atm.amegroups.com/article/view/10.21037/atm-22-2497/dss
Conflicts of Interest: All authors have completed the ICMJE uniform disclosure form (available at https://atm.amegroups.com/article/view/10.21037/atm-22-2497/coif). The authors have no conflicts of interest to declare.
Ethical Statement: The authors are accountable for all aspects of the work in ensuring that questions related to the accuracy or integrity of any part of the work are appropriately investigated and resolved. The study was conducted in accordance with the Declaration of Helsinki (as revised in 2013). The study was approved by the Medical Ethics Committee of Hospital of Stomatology, Jilin University (No. 2020041), and all participants provided their informed consent.
Open Access Statement: This is an Open Access article distributed in accordance with the Creative Commons Attribution-NonCommercial-NoDerivs 4.0 International License (CC BY-NC-ND 4.0), which permits the non-commercial replication and distribution of the article with the strict proviso that no changes or edits are made and the original work is properly cited (including links to both the formal publication through the relevant DOI and the license). See: https://creativecommons.org/licenses/by-nc-nd/4.0/.
References
- Fleming PS, Marinho V, Johal A. Orthodontic measurements on digital study models compared with plaster models: a systematic review. Orthod Craniofac Res 2011;14:1-16. [Crossref] [PubMed]
- Tanaka Y, Hattori Y. Dimensional and occlusal accuracy of a novel three-dimensional digital model of articulated dental arches. Int J Prosthodont 2013;26:282-7. [Crossref] [PubMed]
- Cai HX, Jia Q, Shi H, et al. Accuracy and Precision Evaluation of International Standard Spherical Model by Digital Dental Scanners. Scanning 2020;2020:1714642. [Crossref] [PubMed]
- Gül Amuk N, Karsli E, Kurt G. Comparison of dental measurements between conventional plaster models, digital models obtained by impression scanning and plaster model scanning. Int Orthod 2019;17:151-8. [Crossref] [PubMed]
- Sękowska A, Chałas R. An odontometric study of tooth dimension in diastematic dentition. Folia Morphol (Warsz) 2020;79:604-9. [Crossref] [PubMed]
- Kirschneck C, Kamuf B, Putsch C, et al. Conformity, reliability and validity of digital dental models created by clinical intraoral scanning and extraoral plaster model digitization workflows. Comput Biol Med 2018;100:114-22. [Crossref] [PubMed]
- Rangel FA, Maal TJJ, de Koning MJJ, et al. Integration of digital dental casts in cone beam computed tomography scans-a clinical validation study. Clin Oral Investig 2018;22:1215-22. [Crossref] [PubMed]
- Park SH, Byun SH, Oh SH, et al. Evaluation of the Reliability, Reproducibility and Validity of Digital Orthodontic Measurements Based on Various Digital Models among Young Patients. J Clin Med 2020;9:2728. [Crossref] [PubMed]
- Wesemann C, Muallah J, Mah J, et al. Accuracy and efficiency of full-arch digitalization and 3D printing: A comparison between desktop model scanners, an intraoral scanner, a CBCT model scan, and stereolithographic 3D printing. Quintessence Int 2017;48:41-50. [PubMed]
- Kihara H, Hatakeyama W, Komine F, et al. Accuracy and practicality of intraoral scanner in dentistry: A literature review. J Prosthodont Res 2020;64:109-13. [Crossref] [PubMed]
- Kang SH, Kim CS, Lee JY. Digital setting of postoperative planned occlusion using occlusal contact points on a dental cast model in digital maxillofacial surgery. Oral Radiol 2020;36:307-12. [Crossref] [PubMed]
- Ho CT, Lin HH, Lo LJ. Intraoral Scanning and Setting Up the Digital Final Occlusion in Three-Dimensional Planning of Orthognathic Surgery: Its Comparison with the Dental Model Approach. Plast Reconstr Surg 2019;143:1027e-36e. [Crossref] [PubMed]
- Koretsi V, Kirschbauer C, Proff P, et al. Reliability and intra-examiner agreement of orthodontic model analysis with a digital caliper on plaster and printed dental models. Clin Oral Investig 2019;23:3387-96. [Crossref] [PubMed]
- Yau HT, Yang TJ, Chen YC. Tooth model reconstruction based upon data fusion for orthodontic treatment simulation. Comput Biol Med 2014;48:8-16. [Crossref] [PubMed]
- Barone S, Paoli A, Razionale AV. Geometrical modeling of complete dental shapes by using panoramic X-ray, digital mouth data and anatomical templates. Comput Med Imaging Graph 2015;43:112-21. [Crossref] [PubMed]
- O'Neil R, Kau CH. Comparison of dental arch forms created from assessment of teeth, alveolar bone, and the overlying soft tissue. J Orofac Orthop 2021;82:413-21. [Crossref] [PubMed]
- Richter AE, Arruda AO, Peters MC, et al. Incidence of caries lesions among patients treated with comprehensive orthodontics. Am J Orthod Dentofacial Orthop 2011;139:657-64. [Crossref] [PubMed]
- Baan F, de Waard O, Bruggink R, et al. Virtual setup in orthodontics: planning and evaluation. Clin Oral Investig 2020;24:2385-93. [Crossref] [PubMed]
- Talaat S, Ghoneima A, Kaboudan A, et al. Three-dimensional evaluation of the holographic projection in digital dental model superimposition using HoloLens device. Orthod Craniofac Res 2019;22:62-8. [Crossref] [PubMed]
- Grünheid T, McCarthy SD, Larson BE. Clinical use of a direct chairside oral scanner: an assessment of accuracy, time, and patient acceptance. Am J Orthod Dentofacial Orthop 2014;146:673-82. [Crossref] [PubMed]
- Aragón ML, Pontes LF, Bichara LM, et al. Validity and reliability of intraoral scanners compared to conventional gypsum models measurements: a systematic review. Eur J Orthod 2016;38:429-34. [Crossref] [PubMed]
- Malik J, Rodriguez J, Weisbloom M, et al. Comparison of Accuracy Between a Conventional and Two Digital Intraoral Impression Techniques. Int J Prosthodont 2018;31:107-13. [Crossref] [PubMed]
- Jeong ID, Lee JJ, Jeon JH, et al. Accuracy of complete-arch model using an intraoral video scanner: An in vitro study. J Prosthet Dent 2016;115:755-9. [Crossref] [PubMed]
- Moreira FC, Vaz LG, Guastaldi AC, et al. Potentialities and limitations of computer-aided design and manufacturing technology in the nonextraction treatment of Class I malocclusion. Am J Orthod Dentofacial Orthop 2021;159:86-96. [Crossref] [PubMed]
- Di Ventura A, Lanteri V, Farronato G, et al. Three-dimensional evaluation of rapid maxillary expansion anchored to primary molars: direct effects on maxillary arch and spontaneous mandibular response. Eur J Paediatr Dent 2019;20:38-42. [PubMed]
- Tepedino M, Cornelis MA, Chimenti C, et al. Correlation between tooth size-arch length discrepancy and interradicular distances measured on CBCT and panoramic radiograph: an evaluation for miniscrew insertion. Dental Press J Orthod 2018;23:39 e1- e13.
- Felter M, Lenza MMO, Lenza MG, et al. Comparative study of the usability of two software programs for visualization and analysis of digital orthodontic models. J Dent Res Dent Clin Dent Prospects 2018;12:213-20. [Crossref] [PubMed]
- Brandão MM, Sobral MC, Vogel CJ. Reliability of Bolton analysis evaluation in tridimensional virtual models. Dental Press J Orthod 2015;20:72-7. [Crossref] [PubMed]
- Collins FS, Varmus H. A new initiative on precision medicine. N Engl J Med 2015;372:793-5. [Crossref] [PubMed]
- Francisco I, Ribeiro MP, Marques F, et al. Application of Three-Dimensional Digital Technology in Orthodontics: The State of the Art. Biomimetics (Basel) 2022;7:23. [Crossref] [PubMed]
- De Felice ME, Nucci L, Fiori A, et al. Accuracy of interproximal enamel reduction during clear aligner treatment. Prog Orthod 2020;21:28. [Crossref] [PubMed]
- Kalemaj Z, Levrini L. Quantitative evaluation of implemented interproximal enamel reduction during aligner therapy. Angle Orthod 2021;91:61-6. [Crossref] [PubMed]
- Normando D, Almeida MA, Quintão CC. Dental crowding: the role of genetics and tooth wear. Angle Orthod 2013;83:10-5. [Crossref] [PubMed]
- Kato M, Arai K. Relationship between dental and basal arch forms in mandibular anterior crowding. Am J Orthod Dentofacial Orthop 2022;161:53-64. [Crossref] [PubMed]
- Kolte AP, Kolte RA, Bawankar P. Proximal contact areas of maxillary anterior teeth and their influence on interdental papilla. Saudi Dent J 2018;30:324-9. [Crossref] [PubMed]
(English Language Editor: J. Jones)