The effects of dexmedetomidine on the cognitive function of mild cognitive impairment (MCI) rats
Introduction
Recently, the phenomenon of aging, accompanied by an increase in the incidence of cognitive dysfunction in the elderly, has become increasingly prominent in China. Mild cognitive impairment (MCI) falls between normal aging and dementia (1,2). The pathogenesis of MCI is still controversial; however, it may be closely related to age, surgical trauma, and the use of anesthetics. Surgery, anesthesia and other traumatic factors cause perioperative inflammatory reactions in elderly patients, which produce a large number of inflammatory factors, enter the nervous system, destroy neurons in the hippocampal area and cause dysfunction in learning, memory, cognition and other functions. Some scholars have emphasized that the risk of developing severe cognitive impairment and even dementia is greatly increased in patients diagnosed with MCI preoperatively. Thus, it is important to highlight the MCI phase and prevent it from developing into Alzheimer’s disease (AD) (3,4). Dexmedetomidine (DEX) is a new type of high selectivity, alpha 2 adrenergic agonists by excited blue spot on the nuclear alpha 2 adrenergic receptor, reduce the sympathetic activity, inhibiting the release of norepinephrine, produce a dose-dependent sedative and hypnotic, anxiety, as a good auxiliary anesthetic drug can reduce the stress response caused by anesthesia. The emergence of DEX has contributed to decreased cognitive dysfunction. In this study, we established an MCI rat model and administered DEX during anesthesia to investigate whether this drug could improve postoperative cognitive function in MCI patients and its possible mechanism of action. We present the following article in accordance with the ARRIVE reporting checklist (available at https://atm.amegroups.com/article/view/10.21037/atm-22-2043/rc).
Methods
Ethical statement
Animal experiments were performed under a project license (No. 2021-9CAARR282) granted by Institutional Animal Care and Use Ethics Committee of the Fujian Medical University, in compliance with Chinese national guidelines for the care and use of animals.
Materials
Forty-eight male Sprague-Dawley (SD) rats (weighing 300±50 g, 3 months old) were provided by the Shanghai SLAC Laboratory Animal Co. Ltd. (license number: SCXK [Shanghai] 2012-0002). All animal experiments were performed in accordance with the relevant regulations of the Animal Protection Commission of Fujian province. D-galactose (D-gal) was provided by AMRESCO. Interleukin (IL)-1, interleukin (IL)-6, and a tumor necrosis factor (TNF)-α test kit was provided by R&D Systerm.
Animals
The 48 rats were fed and provided water freely under standard conditions for 1 week. They were randomly divided into the following 4 groups: Model Groups A, B, C, and D (the control group). D-gal 1,000 mg·kg−1·d−1 was injected subcutaneously under the neck and back of each rat in the model groups for 1 week. Group D was subcutaneously injected with the same amount of normal saline for 1 week.
The rats in each group were kept in cages in a clean animal laboratory in a quiet and well-ventilated environment with a temperature of 20–28 °C, a relative humidity of approximately 50%, and 12 hours of alternate lighting. Four rats were kept in each cage and could feed and drink freely during the experiment period. Each rat was weighed once a day, and its general condition (i.e., hair, mental state, foraging behavior, and mobility) was observed.
Morris water maze (MWM) testing
The MWM test was performed 1 day before the injection of galactose and 24 hours after the injection. For the positioning and navigation experiment, the water pool was divided into 4 quadrants (I, II, III, and IV), and a quadrant was randomly selected as the starting quadrant. The rats were placed in a pool facing the pool wall, and the time between the rat’s entry into the water and when the rat found the platform (i.e., the escape latency period) was recorded. If the rat failed to find the platform after 90 s, it was guided to the platform and allowed to stay there for 15 s, and the escape latency period was recorded as 90 s. All 4 quadrants in the experiment had to be completed. The interval between the 2 experiments was 10–15 min. The experiment was repeated for 5 days. For the space exploration experiment, the escape platform was removed from the pool 1 day after the navigation experiment. The rats were placed in the water in the opposite quadrant of the original platform quadrant, and the time when the rats crossed the platform was recorded for up to 60 s.
Inhalation anesthesia experiment
After the completion of the experiment, each rat’s caudal veins were opened and connected to a trace pump. Next, the rat was placed in an acrylic, rectangle, transparent box with a 5-mm diameter hole on 3 sides. One of the side holes was connected to the oxygen flow pipe and another extension tube to monitor the concentration of sevoflurane in the box. The 2 other holes were used for the extension of the caudal vein and exhaust. Rats in Group A continuously inhaled 2% sevoflurane for 2 h, which was intraoperatively pumped with 0.25 mL·100 g−1·h−1 0.9% normal saline. Rats in Group B continuously inhaled 2% sevoflurane for 2 h, which was intraoperatively pumped with 10 µg·kg−1 DEX. Rats in Group C were pumped normal saline (0.25 mL·100 g−1·h−1). Rats in Group D inhaled 2% sevoflurane for 2 h, which was intraoperatively pumped with 0.25 mL·100 g−1·h−1 0.9% normal saline. The Minimum Alveolar Concentration (MAC) values of sevoflurane and carbon dioxide at the end of the expiratory breath of the rats were monitored intraoperatively, and the breathing conditions of the rats were observed according to the respiratory waveform. The breathing rate, extremity color, and labial color of the rats were monitored during the operation. According to animal experiments, the dose of DEX for rats is 10–30 times that of adults, and according to the recommended dose of DEX in the manual (initial dose is 0.5 µg/kg, maintenance dose is 0.2–0.6 µg/kg), it can be inferred that the initial dose of DEX for rats is 5–15 µg/kg and maintenance dose is 2–21 µg/kg. Preliminary studies have confirmed that 10 µg/kg DEX can improve cognitive function in septic rats, possibly by inhibiting the release of inflammatory factors.
Tissue preparation and histological assessment
The brain tissue (hippocampus) of the rats were harvested 12 hours after the MWM testing. The rats were anesthetized and perfused with normal saline, followed by 4% paraformaldehyde in a 0.1-m phosphate buffer (pH, 7.4). After perfusion, the brain tissue was quickly removed and fixed in a fixative solution for 24 h. The next day, paraffin embedding was performed, a coronal section of the hippocampal tissue was removed, and hematoxylin and eosin staining was performed. The pathological changes of the hippocampal tissues were observed under the light microscope under 100× and 400× magnification.
Enzyme-linked immunosorbent assay (ELISA) brain homogenate inflammatory mediator measurement
Twenty-four hours after the completion of the MWM testing, the rats were anesthetized with 10% chloral hydrate. The harvested brain tissue was quickly dried with filter paper, 4% phosphate-buffered saline homogenate medium was added, and the paper was homogenized in an ice bath. The concentration of IL-1, IL-6, and TNF-α in the homogenate was determined by an ELISA after the supernatant was extracted with a pipette for 20 min at 2,500 RPM.
MCI rat identification
The previous work of this study has confirmed that the rats injected with 1,000 mg·kg−1·d−1 D-gal in the neck and back showed mild neuropathological changes and decreased cholinergic system function, resulting in slight oxidative damage and weakened antioxidant capacity, and mild impairment of spatial learning and memory ability, while walking and foraging behavior remained unchanged. In line with the pathological process and characteristics requirements of MCI, the previous work of this study has confirmed that the rats injected with 1,000 mg·kg−1·d−1 d-Gal on the neck and back showed mild neuropathological changes and decreased cholinergic system function, resulting in slight oxidative damage and weakened antioxidant capacity, and mild impairment of spatial learning and memory ability. There was no change in locomotion and foraging behavior, which was consistent with the pathological process and characteristics of MCI (5).
Statistical analysis
Data are expressed as mean ± standard error of the mean. Data for rats’ weight, swim speed, platform crossing time, and inflammatory factor levels were tested for normality and variance homogeneity. When these conditions were met, group differences were evaluated by a 1-way analysis of variance (ANOVA), using the least significant difference for multiple pairwise comparisons. When the conditions of normality and variance homogeneity were not satisfied, group differences were evaluated using a nonparametric rank-sum test. Interactions between time (in the MWM testing procedure) and group factors were evaluated by a 2-way ANOVA with repeated measurements. P values less than 0.05 were considered statistically significant.
Results
Animal characteristics
No accidental deaths occurred during the administration of anesthesia.
There was no significant difference in body weight and swimming speed before and after modeling (Figures 1,2; P<0.05). Additionally, the daily life behaviors of the rats in terms of drinking water and foraging were not affected. The signs of life in rats in the inhalation experiment were relatively stable, and only minimal reductions in rats’ respiratory and heart rates during the operation were noted. The color of rats’ skin and mucous membranes were normal, and did not show any significant signs of hypoxia. There was no significant difference in recovery time of rats.
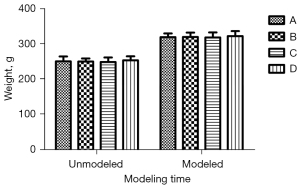
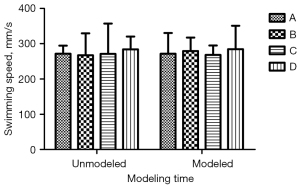
MWM test performance
As shown by the ANOVA results for repeated measurements, there was no statistically significant difference in the escape latency between Groups A, B, C, and D before modeling. After modeling, there was no statistical difference in the escape latency between Groups A, B, and C, but the difference was statistically significant when compared to Group D (Figure 3, P<0.05).
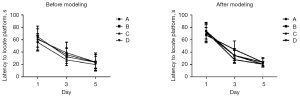
Experimental results after the intervention of DEX
As shown by the ANOVA results for repeated measurements, compared to Group D, the escape latencies of Groups A, B, and C were significantly prolonged, and the difference was statistically significant (Figure 4, P<0.05). Among Groups A, B, and C, Group A took the longest time, and the difference between the Groups B and C was not statistically significant (Figure 4, P>0.05). The 1-way ANOVA showed that compared to Group D, the number of times that rats in Groups A, B, and C crossed the platform was significantly reduced, and the difference was statistically significant (P<0.05). Further, in relation to Groups A, B, and C, rats in Group A crossed the platforms the least number of times; however, there was no statistically significant difference between Groups B and C (Figure 5, P>0.05).
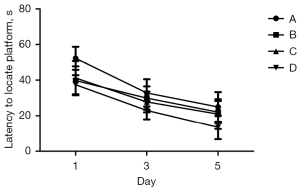
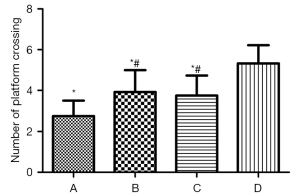
Protein levels detected by an ELISA
Compared to Group D, the contents of IL-1, IL-6, and TNF-α in the brain homogenates of rats in Groups A, B, and C were significantly increased (Figure 6, P<0.05). The levels of IL-1, IL-6 and TNF-α in Groups A, B, and C were the highest, followed by those in Groups B and C; however, there was no statistically significant difference between Groups B and C (Figure 6, P>0.05).
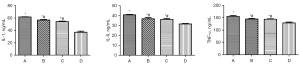
Histological assessments
The histopathological morphological changes of the hippocampus are shown in Figures 7,8. The histopathological morphological results of the rat hippocampal tissue showed that the number of vertebral somatic cells in Group D was normal and arranged in an orderly manner and had a normal density, normal cell size, complete cell structure, clear intracytoplasmic structure, centered nucleus, and obvious nucleoli. However, in Group A, the number of vertebral body cells decreased, the arrangement was disordered and loose, and the density decreased, and a large number of vertebral body cells showed nuclear shrinkage, hyper chromaticity, and neuronal apoptosis. The changes of vertebral cells in Group B and Group C were slight, with orderly arrangement and intact cell structure.
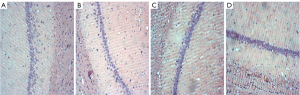
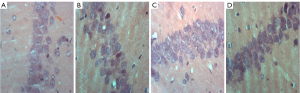
Discussion
Some scholars have confirmed that compared with non-MCI patients, MCI patients are more likely to develop dementia or AD (6-8). Previous research has shown that MCI is associated with shrinkage of the hippocampal structure and volume in the hippocampus, entorhinal cortex, neocortex, and other brain regions. In the morphometry of the high-resolution hippocampus, atrophy of the bilateral hippocampal CA1 and right hippocampal CA2 and CA3 regions is more significant in patients with mild cognitive dysfunction (9) than patients without mild cognitive dysfunction, and there is a loss of cerebral prefrontal gray matter (10). As the anatomical basis of learning and memory, the hippocampus is an important structure of the central memory circuit of the nerve and an ideal model for studying the learning and memory functions of the brain. The CA1 region is believed to be mainly related to spatial learning and memory. Thus, in this experiment, pathological sections were selected from the hippocampus and pathological changes in the CA1 region were observed, as these could more sensitively reflect the pathological basis of cognitive impairments in rats.
During the perioperative period, a variety of risk factors may further aggravate the deterioration of postoperative cognitive functions in patients with MCI, even in patients with dementia, and reduce patients’ quality of life postoperatively. Many inflammatory factors, such as IL-6, IL-1, and TNF-α, are produced in the perioperative period in elderly patients due to trauma related to surgery and the administration of anesthesia. These inflammatory cytokines can enter the nervous system, destroy neurons, and interfere with the transmission of nerve impulses. DEX is a novel, highly selective and highly efficient α-2 adrenoceptor agonist. Its receptor binding ratio of 2 to 1 is 1,620:1, and its specificity is 8 times higher than that of clonidine. It can produce a dose-dependent sedation and analgesia and has anti-anxiety properties. A number of clinical trials have confirmed that DEX has a significant neuroprotective effect (11,12).
During the earlier stage of this study the backs of the necks of the rats injected with 1,000 mg·kg−1·d−1 showed mild nerve pathological changes, cholinergic system function impairment, and mild oxidative damage. Further, oxidation resistance was abated, and minimal damage to spatial learning and memory ability was noted. No changes in the motivation and foraging behaviors were observed that would meet the requirements of the pathological process and characteristics of MCI (13).
The MWM test is sensitive to hippocampal lesions, and its escape latency can be used as the main indicator of the learning and memory ability of rats (14). In relation to the water maze experiment, compared to Group C, the swimming speeds of Groups A and B were slower, the escape latencies of Groups A and B were prolonged, and the number of times that the rats crossed the platform for 60 s in Groups A and B were significantly reduced. After the inhalation of 2% sevoflurane for 2 h, the administration of DEX significantly shortened the escape incubation period, increased the number of times that the rats traversed the platform, and improved the learning and memory impairment caused by sevoflurane.
Traumatic stress induced by surgery and the use of anesthetics can lead to changes in systemic endocrine hormones and the release of inflammatory cytokines. Inflammatory cytokines can cause neuroinflammation, cellular apoptosis, and damage to the blood-brain functional barrier and endothelium, which in turn can aggravate cognitive impairment. Multiple studies (15-17) have confirmed that the central inflammatory response may be the main mechanism leading to cognitive dysfunction. DEX has a highly effective anti-inflammatory effect. The results showed that the levels of IL-1, IL-6, and TNF-α in the brain homogenates of Groups A and B were higher than those of Group C, and the levels of inflammatory factors in Group A were significantly increased. The pathological section of the experimental hippocampus also showed obvious pathological changes in the CA1 area of the hippocampus in Group A. Pathological changes observed in Group B, compared to Group A, showed that in cases of mild cognitive dysfunction, receiving sevoflurane inhalation anesthesia can lead to an increase in the levels of rat tissue inflammatory factors (IL-6, IL-1, and TNF-α), a lack of vertebral body cells, and abnormal cell structure. Further, DEX can significantly reduce the inflammatory cytokine levels of tissues, thereby protecting the neurons.
This experimental study found that DEX may have a neuroprotective effect via its anti-inflammatory effect, inhibit the production of pro-inflammatory factors IL-1 and TNF-α, and reduce neuronal apoptosis caused by surgery and anesthesia stress, thereby exerting a brain protection effect and reducing the incidence of inflammatory cytokines (POCD). However, this study is limited in that it only confirmed the clinical effect of DEX at the molecular level; thus, its in-depth mechanism needs to be explored further.
Acknowledgments
Funding: This work was supported by Fujian Provincial Health Technology Project (No. 2020CXB023), and Startup Fund for Scientific Research of Fujian Medical University (No. 2019QH1081).
Footnote
Reporting Checklist: The authors have completed the ARRIVE reporting checklist. Available at https://atm.amegroups.com/article/view/10.21037/atm-22-2043/rc
Data Sharing Statement: Available at https://atm.amegroups.com/article/view/10.21037/atm-22-2043/dss
Conflicts of Interest: All authors have completed the ICMJE uniform disclosure form (available at https://atm.amegroups.com/article/view/10.21037/atm-22-2043/coif). QZ received consulting fees from AMCA. The other authors have no conflicts of interest to declare.
Ethical Statement: The authors are accountable for all aspects of the work in ensuring that questions related to the accuracy or integrity of any part of the work are appropriately investigated and resolved. Animal experiments were performed under a project license (No. 2021-9CAARR282) granted by Institutional Animal Care and Use Ethics Committee of the Fujian Medical University, in compliance with Chinese national guidelines for the care and use of animals.
Open Access Statement: This is an Open Access article distributed in accordance with the Creative Commons Attribution-NonCommercial-NoDerivs 4.0 International License (CC BY-NC-ND 4.0), which permits the non-commercial replication and distribution of the article with the strict proviso that no changes or edits are made and the original work is properly cited (including links to both the formal publication through the relevant DOI and the license). See: https://creativecommons.org/licenses/by-nc-nd/4.0/.
References
- Nelson AP, O'Connor MG. Mild cognitive impairment: a neuropsychological perspective. CNS Spectr 2008;13:56-64. [Crossref] [PubMed]
- Petersen RC. Mild cognitive impairment as a diagnostic entity. J Intern Med 2004;256:183-94. [Crossref] [PubMed]
- Kazmierski J, Banys A, Latek J, et al. Mild cognitive impairment with associated inflammatory and cortisol alterations as independent risk factor for postoperative delirium. Dement Geriatr Cogn Disord 2014;38:65-78. [Crossref] [PubMed]
- Hudetz JA, Patterson KM, Pagel PS. Comparison of pre-existing cognitive impairment, amnesic mild cognitive impairment, and multiple domain mild cognitive impairment in men scheduled for coronary artery surgery. Eur J Anaesthesiol 2012;29:320-5. [Crossref] [PubMed]
- Chen T, Zhou Q, Xie D, et al. The method and effect of rapid establishment of a mild cognitive impairment model. Ann Palliat Med 2020;9:4119-26. [Crossref] [PubMed]
- Petersen RC, Smith GE, Waring SC, et al. Mild cognitive impairment: clinical characterization and outcome. Arch Neurol 1999;56:303-8. [Crossref] [PubMed]
- Lim A, Tsuang D, Kukull W, et al. Clinico-neuropathological correlation of Alzheimer's disease in a community-based case series. J Am Geriatr Soc 1999;47:564-9. [Crossref] [PubMed]
- Knopman DS, Parisi JE, Boeve BF, et al. Vascular dementia in a population-based autopsy study. Arch Neurol 2003;60:569-75. [Crossref] [PubMed]
- Yassa MA, Stark SM, Bakker A, et al. High-resolution structural and functional MRI of hippocampal CA3 and dentate gyrus in patients with amnestic Mild Cognitive Impairment. Neuroimage 2010;51:1242-52. [Crossref] [PubMed]
- Barbeau EJ, Ranjeva JP, Didic M, et al. Profile of memory impairment and gray matter loss in amnestic mild cognitive impairment. Neuropsychologia 2008;46:1009-19. [Crossref] [PubMed]
- Li Y, He R, Chen S, et al. Effect of dexmedetomidine on early postoperative cognitive dysfunction and peri-operative inflammation in elderly patients undergoing laparoscopic cholecystectomy. Exp Ther Med 2015;10:1635-42. [Crossref] [PubMed]
- Lewis LD, Weiner VS, Mukamel EA, et al. Rapid fragmentation of neuronal networks at the onset of propofol-induced unconsciousness. Proc Natl Acad Sci U S A 2012;109:E3377-86. [Crossref] [PubMed]
- Chen T, Zhou Q, Xie D, et al. The method and effect of rapid establishment of a mild cognitive impairment model. Ann Palliat Med 2020;9:4119-26. [Crossref] [PubMed]
- de Vasconcellos AP, Zugno AI, Dos Santos AH, et al. Na+,K(+)-ATPase activity is reduced in hippocampus of rats submitted to an experimental model of depression: effect of chronic lithium treatment and possible involvement in learning deficits. Neurobiol Learn Mem 2005;84:102-10. [Crossref] [PubMed]
- Wan Y, Xu J, Ma D, et al. Postoperative impairment of cognitive function in rats: a possible role for cytokine-mediated inflammation in the hippocampus. Anesthesiology 2007;106:436-43. [Crossref] [PubMed]
- Rosczyk HA, Sparkman NL, Johnson RW. Neuroinflammation and cognitive function in aged mice following minor surgery. Exp Gerontol 2008;43:840-6. [Crossref] [PubMed]
- Cibelli M, Fidalgo AR, Terrando N, et al. Role of interleukin-1beta in postoperative cognitive dysfunction. Ann Neurol 2010;68:360-8. [Crossref] [PubMed]
(English Language Editor: L. Huleatt)