Quantification of clearance and creep in acetabular wear measurements
Introduction
The consequences of polyethylene (PE) wear remain a principal cause of long-term failure in total hip arthroplasty (THA) (1-5). In vivo measurements of PE wear are regularly made to identify patients at risk, estimate the time for component wear-through, compare performance of bearing couples, and adjust frequency of follow-up of osteolytic lesions in patients at risk (6,7).
Wroblewski et al. (8) and Sychterz et al. (9) were among the first to describe the biphasic pattern of wear in THA, which is rapid in an initial “bedding-in” phase, but relatively slow in a later “steady state” phase. The studies highlighted that femoral head penetration during the “bedding-in” phase is not entirely caused by abrasive wear, and that it should be subtracted from annual penetration measurements, in order to estimate true wear rates. Numerous studies thereafter affirmed that “bedding-in” lasts 6 to 24 months depending on implant design and type of PE (10-18). There is little consensus in the literature, however, on the precise definition of “bedding-in”, other than it comprising a combination of creep and abrasive wear of the PE.
A prosthetic hip joint is considered perfectly spherical from a mechanical perspective, but in reality the outer diameter of the femoral head is slightly smaller than the inner diameter of the acetabular liner, and therefore the components articulate with some eccentricity. This eccentricity is termed ‘clearance’, and is important to ensure smooth tribology, as it prevents jamming and facilitates lubrication with synovial fluid (19-25). Each bearing couple has a unique clearance, which depends on its articular diameter and materials. Consequently, ‘absolute’ measurements of femoral head penetration (at a specific instant of time) include the clearance, which is not caused by wear nor creep, and this is why ‘relative’ measurements of femoral head penetration (subtraction of two absolute measurements at different instants of time) are required to estimate true wear (13,16,17,26). Numerous authors described clearance qualitatively, but to our knowledge, there are no published studies that analyze clearance quantitatively before the occurrence of true wear.
The aim of this study was to measure the initial femoral head penetration before occurrence of true wear, and to quantify the portions caused respectively by clearance and creep in three types of acetabular component: monobloc cemented all-PE (control), modular uncemented metal-backed PE, and modular uncemented dual-mobility PE. Such data would allow measurement of true PE wear and enable more realistic monitoring and comparison of bearing couples and implant survivorship.
Methods
From the database of patients operated for THA between May and July 2015 by the senior author (EV) at our centre, we studied post-operative computed tomography (CT) scans of 15 patients, recruited from a routine surgery waiting list for treatment of primary hip arthritis. For both ethical and cost considerations of exposing patients to additional radiation for the purpose of this research, special permission was approved in advance from our institutional review board, and signed informed consent was obtained from each patient.
The patients included 7 men and 9 women aged 57 to 82 years. All patients received the same cemented Exafit® cobalt-chromium (CoCr) femoral stem (Zimmer, Warsaw, IN, USA), assembled with a diameter 28 mm Exafit® CoCr femoral head (Zimmer, Warsaw, IN, USA), but received one of three different acetabular cups (Figure 1):
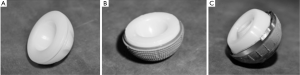
Group A: five patients received a cemented Durasul® highly cross-linked all-PE acetabular cup (Zimmer Inc., Warsaw, IN, USA). The mean weight of patients was 71.4 kg. The engineering drawings indicate that this cup has an articular centre of rotation 3.3 mm above the equatorial titanium ring of cross-sectional diameter 0.8 mm, which is press-fitted in slot of 0.7 mm (Figure 1A).
Group B: five patients received an un-cemented Allofit® metal-backed titanium acetabular cup (Zimmer Inc., Warsaw, IN, USA), with a press-fitted Durasul® highly cross-linked PE liner (Zimmer Inc., Warsaw, IN, USA). The mean weight of patients was 74.4 kg. The engineering drawings indicate that the cup is not a perfect hemisphere but that its articular centre of rotation coincides with the center of its rim (Figure 1B).
Group C: five patients received an un-cemented Stafit® dual-mobility CoCr acetabular cup (Zimmer Inc., Warsaw, IN, USA), with a mobile Sulene® conventional PE liner (Zimmer Inc., Warsaw, IN, USA). The mean weight of patients was 72.4 kg. The engineering drawings indicate that the cup is not a perfect hemisphere but that its articular centre of rotation coincides with a plane passing through half of its rim (Figure 1C).
The engineering drawings of all femoral and acetabular implants were made available by the manufacturer (Zimmer, Warsaw, IN, USA). The nominal articular diameters and tolerances indicated were mm for the CoCr femoral head and 28.5±0.1 mm for all the PE acetabular liners. The three implant combinations therefore have a clearance between the CoCr femoral head and the PE acetabular liner in the range 0.400 to 0.690 mm diametrically (0.200 to 0.345 mm radially). The dual-mobility cups have an additional clearance, between the outer surface of the PE acetabular liner and the inner surface of the metallic cup, in the range 0.750 and 1.050 mm diametrically, or 0.375 to 0.525 mm radially.
All patients were allowed to walk on the second post-operative day, and had a pelvic CT scan taken on the fifth post-operative day (‘day five’), just before they were discharged. The patients were scanned following the same protocol using a 64 Slices Multi-Detector Scanner (General Electric, Waukesha, WI). The CT scans were taken with a contiguous thickness of 0.625 mm with settings of 12 kV and 70–120 mA. Each CT scan contained 200 to 350 DICOM images and was recorded on a separate CD-ROM. We used the image processing software OsiriX (Pixmeo SARL, Bernex, Switzerland), dedicated to DICOM images, to generate 3D bone reconstructions of each CT scan (27,28). The 3-D surface reconstructions were created using the surface rendering function in OsiriX with software settings optimized for metal (pixel value, 2,000; high resolution, 0.50; smooth iterations, 80). This CT technique has a proven accuracy of 0.050 mm and repeatability of ±0.040 mm, using cemented all-PE Acoplot implants (Zimmer Inc., Warsaw, IN, USA) or uncemented metal-backed PE Pressfit Kappa Acora implants (Zimmer Inc., Warsaw, IN, USA) (29).
To enable comparison of pre- and post-operative femoral head penetration in the three different acetabular cups, we gathered samples of the three head-cup combinations (groups A, B and C) assembled tightly without application of additional load, and scanned them following the same CT protocol described above.
The resulting 18 CT scans (15 post-operative hips and 3 non-implanted samples) were analyzed as follow: we compared the 3D coordinates of center of the femoral head and the center of the acetabular cup (Figure 2). Forty digitized points were evenly placed on a 3D reconstruction of the femoral head, the metal ring of the all PE cup and the equatorial plane of the metal-backed cups. The coordinates of digitized points were exported using OsiriX to a file as comma-separated variables (CSV). A geometric analysis software, 3D Reshaper® (Technodigit, Gleizé, France), was used to deduce the coordinates of the articular head center by fitting a sphere to its points, and the center of the acetabular component by fitting a circle to its points, in both cases using the method of least squares. The point coordinates were further manipulated in spreadsheets using Microsoft Excel® (Microsoft Corp, Redmond, WA, USA) to calibrate all coordinates to the known femoral head diameter and to deduce the head-cup eccentricity as is the case with Muller-type all-PE acetabular components, with reference to the engineering drawings of each acetabular component.
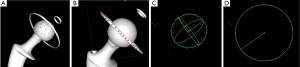
The 3D surface rendering was different for each type of acetabular cup (Figure 3). For the all-PE implants, both the metallic femoral head and the metallic equatorial ring were perfectly visualized, despite few irregularities on the metallic ring. For metal-backed cups, both the CoCr femoral head and the Titanium cup were perfectly visualised without beam-hardening artifacts, but the proximity of components created an equatorial band of increased thickness. Our CT scan analysis remained valid as long as the points were not placed on artifacts. The dual-mobility cups were not well visualized because of beam-hardening artifacts, created by the proximity of the two metallic parts, which degraded the sphericity of the femoral head and rendered it impossible to position points on the femoral head and the PE liner using the 3D reconstructions. We therefore used the 2D orthogonal reconstructions created by OsiriX to calculate the eccentricity between the head and cup for dual-mobility implants.
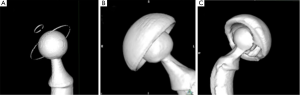
We studied the intra-observer error of our method by blindly repeating the measurement procedure ten times on all 18 CT scans by the same senior surgeon (EV). Statistical analyses were done using SPSS (IBM Corporation, Armonk, New York, USA) and its statistical module.
Results
The total femoral head penetration in PE acetabular liner, measured in vivo from CT scans taken after weight bearing on ‘day five’ (Table 1), was considerably lower for Group A (Durasul® all-PE cups) (0.196 mm) than for Groups B (Allofit® metal-backed cups) (0.551 mm) and C (Stafit® dual-mobility cups (0.634 mm).
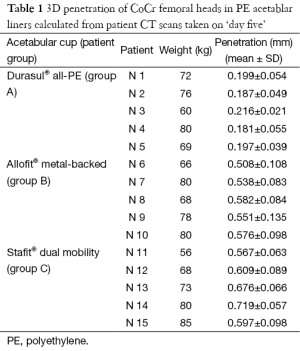
Full table
The clearance measured in vitro from CT scans of assembled implant samples (Table 2) was also lower for the cemented Durasul® all-PE cups (0.149 mm) than for the un-cemented Allofit® metal-backed cups (0.455 mm) and the un-cemented Stafit® dual-mobility cups (0.367 mm).
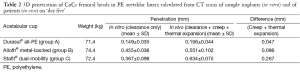
Full table
The intra-observer repeatability of measurements is indicated by the standard deviation (SD), which was generally less than 0.1 mm. For in vitro measurements, the intra-observer repeatability was best for the Durasul® all-PE cups (SD 0.035 mm) and worst for the Stafit® dual-mobility cups (SD 0.098 mm). For in vivo measurements, the intra-observer repeatability was again best for the Durasul® all-PE cups (SD 0.044 mm) but worst for the Allofit® metal-backed cups (SD 0.102 mm).
Discussion
The aim of this study was to measure the initial femoral head penetration in THA before occurrence of true wear, and to quantify the portions attributable to clearance and plastic deformation of PE, in three types of acetabular component. The results revealed that femoral head penetration on ‘day five’ amounts to 0.2 to 0.6 mm, of which 58% to 83% could be attributed to ‘clearance’. The extent of this initial penetration depends on the type of acetabular cup, and is considerable when compared to total ‘bedding-in’ observed in the first years after implantation (8,11).
The total femoral head penetration in a PE liner during the lifetime of a THA could be considered as the sum of four factors (Figure 4): (I) clearance between articular components; (II) thermal expansion of the PE liner upon implantation; (III) rapid creep or plastic deformation upon weight-bearing; and (IV) slow true wear from walking and motion. The penetration measured on ‘day five’ includes only the first three factors and excludes the fourth factor. The existence of multiple factors could explain the usual overestimation of radiological wear measurements in comparison to direct measurement (30). It remains difficult, however, to estimate with certainty the portions due to clearance and to plastic deformation, notably because the effects of thermal expansion are unknown. The in vitro samples were scanned at room temperature around 22 °C, whereas the in vivo implants were scanned at body temperature around 37 °C. This temperature difference of 15 °C would change the liner thickness by 0.2% to 0.3%, through exact dimensional increases cannot be predicted for complex shapes (21). Thermal expansion is an issue rarely raised in orthopedic literature, although PE has one of the highest coefficients of thermal expansion among plastics, about 10 to 20 times greater than that of metals (31).
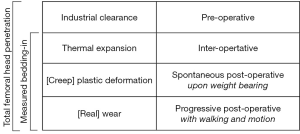
It is worth noting that material properties, including mechanical strength and thermal expansion coefficients, are not identical for all implanted PE liners. Groups A and B received Durasul® highly cross-linked PE liners, whereas Group C received Sulene® conventional PE liners. The elastic limit and creep resistance of highly cross-linked PE is greater than the mechanical strength of conventional PE. This probably explains why the difference between in vitro and in vivo penetration (Table 2) is considerably smaller for Groups A and B than for Group C—and inversely—why the proportion of penetration attributed to clearance is greater for Groups A and B than for Group C. Lee and Pienkowski (32) observed that creep occurs in less than three days, whereas Deng et al. (33) argued that it progresses over the first month. It is therefore possible that our measurements at ‘day five’ may not represent the full extent of creep that those implants could exhibit after the first month.
It is also worth noting that the clearances are not identical for all implanted PE liners: Group A received monolithic all-PE liners which exhibit a single ‘primary’ head-liner clearance, whereas Groups B and C received modular PE liners assembled within metallic shell and thus exhibit an additional ‘secondary’ liner-shell clearance (34). This probably explains why both in vivo and in vitro penetration (Table 2) are considerably lower for Group A than for Groups B and C. Barrack et al. (30) reported that the liner-shell clearance is in the range 0.1 to 0.6 mm for a particular implant model, whereas Sychterz et al. (9) argued that this gap reduces over time with creep.
The repeatability of our measurements is consistent with values reported for the method of Vandenbussche et al. (29) which has a proven accuracy of 0.050 mm and repeatability of ±0.040 mm. The method used in the present study had been validated by comparison with direct measurements, which in our opinion are the Gold Standard, but was not compared to the accuracy of radiological measurement methods such as RSA or other digitized radiological methods such as that of Martell and Berdia (35). The advantages of our method are the use of open-source software, and its rapidity and applicability to all types of acetabular cups whether all-PE or metal-backed. Furthermore, there were no significant weight differences among patients, which implies that head penetration due to plastic deformation under weight bearing is comparable among the three groups. The disadvantages of our method are the need for the engineering drawings of the implants used and the need for postoperative pelvic CT scans with thin 0.625 mm slices.
The impact of clearances is virtually unknown in the orthopaedic community because it has little or no impact on clinical practice. Surgeons may be aware of some clearance to avoid jamming and facilitate lubrication, but they seldom relate this clearance to wear measurements. Few surgeons realize that the average diameter of a “28 mm” ceramic femoral head is 27.975 mm (), and for a cobalt-chromium femoral head is 27.955 mm (
). Penetration of a femoral head in PE over time is usually represented on graphs starting from a zero value on the ordinate (13,16,17,26). It would seem more appropriate to indicate the clearance on such graphs (Figure 5).
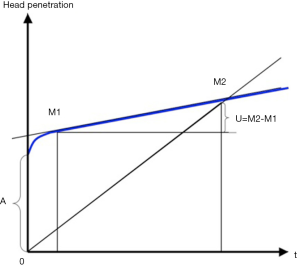
The present study indicates that isolated measurements of femoral head penetration include 0.15 to 0.46 mm of radial clearance and 0.05 to 0.27 mm of creep. The study also confirms that the majority of so-called bedding-in observed in the first post-operative months or years is not entirely due to wear.
Acknowledgements
In memory of, and with gratitude to, Mr. Jean-Pierre Bouraly, former Scientific and Technical Director at Zimmer France, who kindly provided the engineering drawings of implants used this study. The authors are also thankful to Dr. Céline Mutschler, Clinical Radiologist at Hôpital Européen Georges Pompidou, for provision of CT scans.
Footnote
Conflicts of Interest: The authors have no conflicts of interest to declare.
References
- Goodman SB, Ma T. Cellular chemotaxis induced by wear particles from joint replacements. Biomaterials 2010;31:5045-50. [Crossref] [PubMed]
- Harris WH. The problem is osteolysis. Clin Orthop Relat Res 1995.46-53. [PubMed]
- Havelin LI, Fenstad AM, Salomonsson R, et al. The Nordic Arthroplasty Register Association: a unique collaboration between 3 national hip arthroplasty registries with 280,201 THRs. Acta Orthop 2009;80:393-401. [Crossref] [PubMed]
- Holt G, Murnaghan C, Reilly J, et al. The biology of aseptic osteolysis. Clin Orthop Relat Res 2007.240-52. [PubMed]
- Sochart DH. Relationship of acetabular wear to osteolysis and loosening in total hip arthroplasty. Clin Orthop Relat Res 1999.135-50. [PubMed]
- Dumbleton JH, D'Antonio JA, Manley MT, et al. The basis for a second-generation highly cross-linked UHMWPE. Clin Orthop Relat Res 2006.265-71. [Crossref] [PubMed]
- Dowd JE, Sychterz CJ, Young AM, et al. Characterization of long-term femoral-head-penetration rates. Association with and prediction of osteolysis. J Bone Joint Surg Am 2000;82-A:1102-7. [PubMed]
- Wroblewski BM, Siney PD, Dowson D, et al. Prospective clinical and joint simulator studies of a new total hip arthroplasty using alumina ceramic heads and cross-linked polyethylene cups. J Bone Joint Surg Br 1996;78:280-5. [PubMed]
- Sychterz CJ, Engh CA Jr, Shah N, et al. Radiographic evaluation of penetration by the femoral head into the polyethylene liner over time. J Bone Joint Surg Am 1997;79:1040-6. [PubMed]
- D'Antonio JA, Capello WN, Ramakrishnan R. Second-generation annealed highly cross-linked polyethylene exhibits low wear. Clin Orthop Relat Res 2012;470:1696-704. [Crossref] [PubMed]
- Dai X, Omori H, Okumura Y, et al. Serial measurement of polyethylene wear of well-fixed cementless metal-backed acetabular component in total hip arthroplasty: an over 10 year follow-up study. Artif Organs 2000;24:746-51. [Crossref] [PubMed]
- García-Rey E. Bedding-in and true wear in two different generations cementless porous-coated acetabular cups. Hip Int 2010;20 Suppl 7:S86-93. [PubMed]
- Glyn-Jones S, Isaac S, Hauptfleisch J, et al. Does highly cross-linked polyethylene wear less than conventional polyethylene in total hip arthroplasty? A double-blind, randomized, and controlled trial using roentgen stereophotogrammetric analysis. J Arthroplasty 2008;23:337-43. [Crossref] [PubMed]
- Jacobs CA, Christensen CP, Greenwald AS, et al. Clinical performance of highly cross-linked polyethylenes in total hip arthroplasty. J Bone Joint Surg Am 2007;89:2779-86. [Crossref] [PubMed]
- McCalden RW, MacDonald SJ, Rorabeck CH, et al. Wear rate of highly cross-linked polyethylene in total hip arthroplasty. A randomized controlled trial. J Bone Joint Surg Am 2009;91:773-82. [Crossref] [PubMed]
- McCalden RW, Naudie DD, Yuan X, et al. Radiographic methods for the assessment of polyethylene wear after total hip arthroplasty. J Bone Joint Surg Am 2005;87:2323-34. [Crossref] [PubMed]
- Sychterz CJ, Engh CA Jr, Yang A, et al. Analysis of temporal wear patterns of porous-coated acetabular components: distinguishing between true wear and so-called bedding-in. J Bone Joint Surg Am 1999;81:821-30. [PubMed]
- Wroblewski BM, Siney PD, Fleming PA. Low-friction arthroplasty of the hip using alumina ceramic and cross-linked polyethylene. A 17-year follow-up report. J Bone Joint Surg Br 2005;87:1220-1. [Crossref] [PubMed]
- Goebel P, Kluess D, Wieding J, et al. The influence of head diameter and wall thickness on deformations of metallic acetabular press-fit cups and UHMWPE liners: a finite element analysis. J Orthop Sci 2013;18:264-70. [Crossref] [PubMed]
- Jalali-Vahid D, Jagatia M, Jin ZM, et al. Prediction of lubricating film thickness in UHMWPE hip joint replacements. J Biomech 2001;34:261-6. [Crossref] [PubMed]
- Katayama K, Maezawa H, Uesato N, et al. Physicochemical and biomechanical examination of surfaces of retrieved polyethylene heads from total hip prostheses with rotating polyethylene head system. J Orthop Sci 2001;6:503-9. [Crossref] [PubMed]
- Korhonen RK, Koistinen A, Konttinen YT, et al. The effect of geometry and abduction angle on the stresses in cemented UHMWPE acetabular cups--finite element simulations and experimental tests. Biomed Eng Online 2005;4:32. [Crossref] [PubMed]
- Santavirta S, Böhler M, Harris WH, et al. Alternative materials to improve total hip replacement tribology. Acta Orthop Scand 2003;74:380-8. [PubMed]
- Teoh SH, Chan WH, Thampuran R. An elasto-plastic finite element model for polyethylene wear in total hip arthroplasty. J Biomech 2002;35:323-30. [Crossref] [PubMed]
- Wang A, Essner A, Klein R. Effect of contact stress on friction and wear of ultra-high molecular weight polyethylene in total hip replacement. Proc Inst Mech Eng H 2001;215:133-9. [Crossref] [PubMed]
- Hui AJ, McCalden RW, Martell JM, et al. Validation of two and three-dimensional radiographic techniques for measuring polyethylene wear after total hip arthroplasty. J Bone Joint Surg Am 2003;85-A:505-11. [PubMed]
- Rosset A, Spadola L, Ratib O. OsiriX: an open-source software for navigating in multidimensional DICOM images. J Digit Imaging 2004;17:205-16. [Crossref] [PubMed]
- Rosset C, Rosset A, Ratib O. General consumer communication tools for improved image management and communication in medicine. J Digit Imaging 2005;18:270-9. [Crossref] [PubMed]
- Vandenbussche E, Saffarini M, Hansen U, et al. Measurement of femoral head penetration in polyethylene using a 3-dimensional CT-scan technique. Acta Orthop 2010;81:563-9. [Crossref] [PubMed]
- Barrack RL, Lavernia C, Szuszczewicz ES, et al. Radiographic wear measurements in a cementless metal-backed modular cobalt-chromium acetabular component. J Arthroplasty 2001;16:820-8. [Crossref] [PubMed]
- Ehrenstein GW, Riedel G, Trawiel P. Thermal Analysis Of Plastics: Theory and Practice. Carl Hanser Verlag GmbH & Co. KG, 2004.
- Lee KY, Pienkowski D. Compressive creep characteristics of extruded ultrahigh-molecular-weight polyethylene. J Biomed Mater Res 1998;39:261-5. [Crossref] [PubMed]
- Deng M, Latour RA, Ogale AA, et al. Study of creep behavior of ultra-high-molecular-weight polyethylene systems. J Biomed Mater Res 1998;40:214-23. [Crossref] [PubMed]
- Kurtz SM, Ochoa JA, White CV, et al. Backside nonconformity and locking restraints affect liner/shell load transfer mechanisms and relative motion in modular acetabular components for total hip replacement. J Biomech 1998;31:431-7. [Crossref] [PubMed]
- Martell JM, Berdia S. Determination of polyethylene wear in total hip replacements with use of digital radiographs. J Bone Joint Surg Am 1997;79:1635-41. [PubMed]