Evaluation on clinical performance of the new low-value platelet measurement mode (PLT-8X) of BC-6800Plus
Introduction
Platelets (PLTs) are produced by megakaryocytes in the hematopoietic tissues of bone marrow, and have the functions of maintaining the integrity of the vascular endothelium, as well as adhesion, aggregation, release, coagulation, and clot contraction (1). The PLT count is a measure of the number of PLT in a unit volume of blood. It is relatively stable in normal blood. It not only plays a role in coagulation and thrombotic diseases, but is also vital in inflammation, immune diseases, tumors, and other diseases (2). However, due to the special physiological properties of PLT, including small size and large changes in morphology after activation, and the limitation by the detection principle of the conventional impedance method of hematology analyzers, most instruments are prone to interference by many factors such as small red blood cells (RBCs), cell debris, and noise when they are used to count PLTs, which may lead to a false increase in the PLT count; moreover, a false decrease in the PLT count may be result under conditions such as giant PLT and PLT aggregation (3-5). The accuracy of the results is highly valuable for the diagnosis and treatment as well as clinical decision-making in terms of clinical diseases, especially hemorrhagic disorders and hematological system disorders in patients with thrombopenia. Flow cytometry for PLT counting is currently the reference method recommended by the International Committee for Standardization of Hematology (ICSH) for measuring the PLT value (6,7). It detects the ratio of RBC to PLT in the peripheral blood, and then calculates the accurate result of PLT in combination with the RBC count. As flow cytometry is complex to operate and expensive, it is not suitable for routine test items in clinical laboratories.
The optical method for PLT counting (PLT-O) of the BC-6800Plus analyzer (Mindray Global, Shenzhen, China) starts from the reagents and reactions. It can realize self-disaggregation for most of the PLT clump samples, and solves the problem of interference existing in the impedance method from two dimensions: PLT volume and fluorescence. Moreover, the PLT counting mode of multiple statistics is added to address the problems of small particle quantity and low precision in samples with low-value PLT. In the optical detection channel, the analyzer can automatically extend the time to detect particle flows in low-value samples below the specified threshold, and achieve 8 times statistics to ensure high precision and high accuracy at low concentration levels of PLT.
Hematology analyzers are commonly used in clinical laboratories for PLT detection. For samples with critical values, much attention is paid to the accuracy of low-value PLT counts that may affect clinical decision-making. Therefore, this study intended to evaluate the precision of the detection results in the 8 times optical platelet counting (PLT-8X) mode and the accuracy of the PLT reference results obtained from the BriCyte E6 flow cytometer (Mindray), so as to determine whether the performance of the BC-6800Plus auto hematology analyzer in outputting PLT-8X results can meet the testing needs of clinical laboratories in large general hospitals mainly specializing in hematological system disorders. We present the following article in accordance with the MDAR reporting checklist (available at https://atm.amegroups.com/article/view/10.21037/atm-22-1501/rc).
Methods
Samples
A total of 107 fresh anticoagulated venous whole blood samples with low-value PLT were selected from inpatients of our hospital from November 2019 to December 2019, of which 40 samples with the PLT count ≤50×109/L were used for precision analysis, and the other 67 samples with the PLT count ≤100×109/L were used for accuracy comparison with the flow reference method. All blood samples were collected with dipotassium ethylenediaminetetraacetic acid (EDTA-K2) vacuum anticoagulation tubes (Becton, Dickinson, and Co., Franklin Lakes, NJ, USA), with a blood volume of 1.5–2 mL. They were stored at room temperature (18–26 ℃) after collection, and testing was completed within 4 hours. This study was conducted in accordance with the Declaration of Helsinki (as revised in 2013). This study was approved by the ethics committee of the First Affiliated Hospital of Soochow University (No. 2022-159). Due to the study’s retrospective nature, the requirement to obtain signed informed consent from the patients was waived.
Instruments and reagents
The following instruments and reagents were used in our experiments: BC-6800Plus auto hematology analyzer and matching reagents and quality-control substance for calibration (Mindray, China); BriCyte E6 flow cytometer (Mindray, China) and fluorescein isothiocyanate (FITC)-labeled CD41 and CD61 polyclonal antibodies (Beckman Coulter, Brea, CA, USA); phosphate buffer solution containing 1% bovine serum albumin (PBS-BSA buffer solution; Sigma-Aldrich, St. Louis, MO, USA).
Study methods
Precision
A total of 40 fresh anticoagulated venous whole blood samples with the PLT count ≤50×109/L were mixed well, and each sample was tested 10 times in PLT-8X mode by the BC-6800Plus auto hematology analyzer. The test results from PLT-8X and those from the impedance method for PLT counting (PLT-I) were obtained, and the mean, standard deviation (SD), and coefficient of variation (CV%) of the 10 results of the two parameters were calculated respectively. The t-test was performed to analyze imprecision (CV%), a plot was drawn with the mean as the abscissa and the CV% as the ordinate, and the imprecision expander graph (power function) of the two PLT parameters was calculated.
Accuracy
A total of 67 freshly collected anticoagulated venous whole blood samples with the PLT count ≤100×109/L were mixed well, and each sample was tested once in PLT-8X mode by the BC-6800Plus auto hematology analyzer to obtain the PLT-8X results (Y). Moreover, PLT counting was conducted on the BriCyte E6 flow cytometer provided by Mindray by the following method: (I) reaction tubes dedicated for flow cytometer were taken, 100 µL filtered PBS-BSA diluent was added to the bottom of the reaction tubes, and the solution was slightly shaken and mixed well. (II) A pipettor was used to add 5 µL fresh anticoagulated venous whole blood sample to be tested to the 100 µL diluent, and the solution was slight shaken and well mixed. (III) A pipettor was used to add 5 µL CD41-FITC antibody and 5 µL CD61-FITC antibody to the 100 µL diluent, and the solution was slightly shaken and mixed well. (IV) The well-mixed samples were stored at room temperature (18–22 ℃), covered, and incubated away from light for 15 min. (V) A specimen at a dilution of 1:1,000 was prepared by adding 4.85 mL PBS-BSA, and the specimen was turned upside down at least 8–10 times until it was fully mixed. (VI) The test was conducted on the set flow cytometer (at a low rate) 3 consecutive times, the data was recorded, with the total particle count of 50,000 and PLT count of 1,000 as the termination conditions, and the R value was calculated according to the formula R value = (P1 + P3)/(P2 + P3). (VII) The concentration of PLT was calculated according to the formula PLT = RBC × 1,000/R in combination with the calibrated and traceable RBC count (×1012/L) obtained from the BC-6800Plus. (VIII) The mean of the three flow test results was taken as the reference value (X) for the PLT flow method. Passing-Bablok regression analysis was conducted with reference to CLSI EP09-A3, expected biases at different medical decision levels (5×109/L, 10×109/L, 20×109/L and 50×109/L) were calculated, and the Bland-Altman deviation chart was drawn.
Linearity
The linearity was evaluated for PLT within the low-value ranges (0 to 100 and 0 to 30) in PLT-8X mode. The linearity of venous whole blood samples with PLT results slightly higher than the upper limit of the linear range was selected as the linearity at the 100% concentration point in the two ranges, and the linearity of PLT-free plasma was used as the linearity at the 0% concentration point. In addition, venous whole blood and PLT-free plasma were mixed to prepare mixtures at concentration points 25%, 50%, and 75%. That is, there were 5 concentration points (0%, 25%, 50%, 75%, and 100%) in total. All the samples at all concentration points were tested 3 times in PLT-8X autoloading mode and PLT-8X open-vial mode. Statistics on linearity and nonlinearity of the results were collected based on the EP06-A method to evaluate the relative accuracy of PLTs in the low-value range.
Limit of detection (LoD)
The LoD in PLT-8X autoloading mode of BC-6800Plus was evaluated with reference to EP17-A2. A total of 6 tubes of diluent were used as blank samples, and each of them was tested 10 consecutive times in PLT-8X autoloading mode of BC-6800Plus to calculate the limit of blank (LoB) value of the PLT-8X test results. A venous whole blood sample with low-value PLT was selected and tested 3 times in whole blood customized dilution ratio (CDR) mode of BC-6800Plus. The mean of the PLT results was taken as the reference value, and the PLT was diluted with PLT-free plasma at a certain ratio into 6 samples at different low concentrations (1×109/L to 6×109/L), respectively. Each of the 6 samples were tested 12 consecutive times in PLT-8X autoloading mode of BC-6800Plus, and the SD and pooled SD at each concentration level were calculated to further calculate the LoD.
Statistical analysis
Microsoft Excel (Microsoft Corp., Redmond, WA, USA) and Analyse-it v5.11 (https://analyse-it.com/) statistical software were used. The resulting data was expressed as mean ± SD. An imprecision (CV%) expander graph was drawn, and the paired t-test was performed for intergroup comparison. Passing-Bablok regression and Bland-Altman deviation were used to analyze the data for methodological comparison; linear data was analyzed using polynomial regression, and the significant differences in nonlinear coefficients were analyzed using the t-test.
Results
Precision
Precision profiles were drawn with the mean of PLT as the horizontal axis and the CV% between PLT-I and PLT-8X as the vertical axis, as shown in Figure 1. In general, the CV% between the two parameters gradually increased with the decrease in the PLT value, which conformed to the statistical law and the Poisson distribution characteristics. The PLT results were analyzed in different range (Table 1), the CV% of the PLT-8X was basically below 10%, and the imprecision of samples above 10×109/L was generally below 5%. Based on the data distribution and performance, the power function (CV% = C0 × C1) was selected for fitting, and the functional equations of PLT-I and PLT-8X were y = 0.4126x−0.57 and y = 0.1946x−0.599, respectively. The precision of PLT-8X was significantly superior to that of PLT-I (P<0.0001).
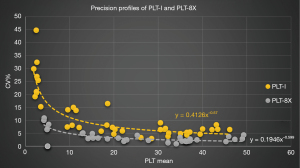
Table 1
PLT range | PLT-8X | PLT-I | P value | |||||
---|---|---|---|---|---|---|---|---|
N | SD | CV% | N | SD | CV% | |||
0 to 10×109/L | 10 | 0.238 | 5.23% | 12 | 0.724 | 23.66% | 0.0021 | |
10×109/L to 20×109/L | 7 | 0.569 | 3.87% | 9 | 1.429 | 9.63% | 0.0040 | |
20×109/L to 30×109/L | 6 | 0.646 | 2.73% | 4 | 1.082 | 4.68% | 0.0439 | |
30×109/L to 50×109/L | 17 | 0.959 | 2.36% | 15 | 2.241 | 6.02% | <0.0001 |
PLT, platelet; PLT-8X, 8 times optical platelet counting; PLT-I, the impedance method for platelet counting; SD, standard deviation; CV%, coefficient of variation.
Accuracy
The flow cytometer RBC/PLT ratio method recommended by ICSH was selected as the reference method for evaluating PLT accuracy. The results of the 67 samples with PLT ≤100×109/L in PLT-8X mode were compared with those obtained from the BriCyte E6 flow cytometer (the flow scatter plot is shown in Figure 2A). Regression analysis (Figure 2B) was conducted on the data using the Passing-Bablok method with reference to EP09-A3. The regression function was y = 1.049x − 0.9807, where the slope was 1.049 (1.007, 1.099), the intercept was −0.9807 (−2.116, 0.2351), and the correlation coefficient was 0.992. Consistency analysis was conducted on the data using the Bland-Altman deviation chart (Figure 2C). Most of the deviations were distributed in 95% limit of agreement (LoA). Moreover, the expected biases at the key low-value medical decision levels (5×109/L, 10×109/L, 20×109/L and 50×109/L) of PLT were −0.73×109/L, −0.49×109/L, 0.0×109/L, and 1.48×109/L, respectively.
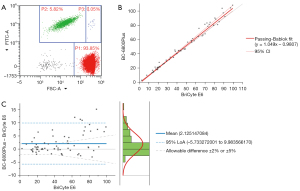
Linearity
The linear results of the PLT-8X parameters in the low-value ranges of 0 to 100 and 0 to 30 are shown in Figure 3. The horizontal axis represents the concentration points of the samples, and the vertical axis represents the PLT-8X test results. In the 0 to 100 low-value range of PLT, the regression equation was y = 100.13x + 0.2667, and the squared R2 of the correlation coefficient was 0.9999; in the 0 to 30 low-value range of PLT, the regression equation was y = 31.867x − 0.1333, and the squared R2 of the correlation coefficient was 0.9977. The polynomial analysis according to EP06-A showed that the linear data of PLT-8X presented a linear model, and there was no significant difference between its nonlinearity coefficient and 0 (P>0.05).
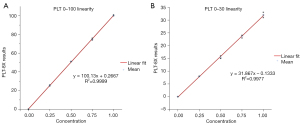
LoD
We took 6 tubes of diluent as blank samples, and each of them was tested 10 consecutive times in whole-blood PLT-8X mode to obtain the test results of PLT-I and PLT-8X. The maximum test values of PLT-I and PLT-8X were 2×109/L and 1×109/L, respectively. We prepared 6 low-value samples with the PLT range of 1 to 6×109/L with EDTA-K2 anticoagulated venous whole blood through the dilution method, and each of them was tested 10 consecutive times in whole-blood PLT-8X mode to obtain the test results of PLT-I and PLT-8X. The frequency distribution diagram of PLT counts in the blank samples and the low-value samples is shown in Figure 4. The LoBs calculated for PLT-I and PLT-8X with reference to EP17-A2 were 0.58×109/L and 0.33×109/L, respectively, and the LoDs were 1.98×109/L and 0.89×109/L, respectively.
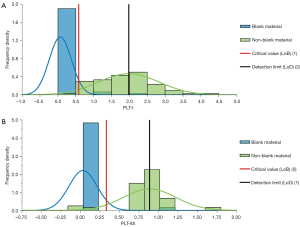
Discussion
The PLT count is one of the important reference indicators for clinically evaluating the coagulation function and bleeding risk of patients, and for making decisions on whether PLT transfusion or surgery can be carried out. Therefore, an accurate PLT count is highly valuable to the clinical diagnosis and treatment process. The high cost resulting from growing PLT transfusions brings great economic burden to patients. Moreover, with the presence of blood group antibodies of PLT, blind PLT transfusions may be ineffective, which will affect subsequent rescue and treatment. Therefore, with improved clinical awareness and control of bleeding risks, it has gradually become a consensus to reduce the threshold for preventive or therapeutic PLT transfusion. However, inaccurate low-value PLT counts will mislead clinical diagnosis and treatment and increase patients’ risks (8). The reference interval for PLTs is between 125×109/L and 350×109/L. In the past, the threshold for PLT transfusion in clinical practice was usually 20×109/L, but in recent years, it has been studied and expected to be 10×109/L or even 5×109/L in the industry (9). Therefore, as demands in clinical practice increase, higher precision and accuracy of PLT results reported in the laboratory are required.
At present, PLT counting methods commonly used in laboratories mainly include the manual counting method, electrical impedance method, and light scattering method, and the latter 2 are automatic analysis methods. Although great progress has been made in theories and technologies of hematology analyzers, the results of PLT counts obtained in many clinical laboratories are still unsatisfactory (10). In the flow cytometer RBC/PLT ratio method recommended by ICSH, CD41 and CD61 antibodies are used to specifically identify PLT. By measuring the ratio of RBC/PLT ratio, the concentration of PLT can be accurately calculated based on the concentration of RBC. However, this method fails to avoid interfering factors. All the factors that affect RBC and PLT are the influencing factors of the flow PLT reference method, and the flow reference method is unsuitable for routine laboratorial use due to its complex operations and high cost. Therefore, a simple and fast method with high precision and accuracy is needed in laboratories, especially for testing samples with low-value PLT (11). Possible inaccuracy caused by various errors or interferences to the test results should be considered in laboratorial reports of PLT results. It is recommended that retesting be carried out by manual counting method if necessary to ensure result accuracy. In addition, PLT aggregation may occur due to some physiological or pathological reasons, partly due to the introduction of interstitial fluid induced by poor blood flow during blood collection, and partly due to EDTA-dependent PLT aggregation, which often results in a false decrease in the test results. Therefore, possible false reductions of PLT caused by small RBCs, RBC debris, large PLT, PLT aggregation, and so on. should be excluded in PLT reports in clinical laboratories (12). At present, laboratories mainly rely on automated instruments to handle massive samples. However, when low-value PLT trigger the critical value on the instrument, the laboratories must prevent false test results with special attention (13).
The BC-6800Plus auto hematology analyzer is a high-end instrument newly launched by Shenzhen Mindray. It has the advantages of fast test speed [up to 200 samples/hour for complete blood count (CBC) and differential detection], high accuracy of results, and strong ability to detect abnormal values and abnormal cells. It uses the impedance method and laser scatter combined with fluorescence staining flow cytometry to classify and count peripheral blood cells, and at the same time uses the colorimetric method for hemoglobin measurement. It can output three parameters in the detection of PLT: PLT-I, PLT-O, and PLT-8X. When the analyzer identifies a PLT-I result lower than the default threshold (50×109/L) during the sample test, it will automatically trigger the 8 times statistics rule, automatically extend the optical PLT counting time, and increase the number of PLT counts included in the statistics. It can significantly reduce the detection imprecision and improve the accuracy of low-value PLT detection. So, the PLT-8X mode takes about half a minute longer than the normal whole blood mode. Although the test efficiency is affected, the test results are more accurate. This study focused on whether the results of the analyzer’s new PLT-8X parameters can meet the clinical needs for low-value PLT detection.
This study has shown that the analyzer’s PLT-8X mode has lower imprecision than the conventional PLT-I mode for samples with PLT ≤50×109/L (P<0.001). For samples with PLT in the range of 20×109/L to 50×109/L, the CV% of PLT-I fell within 10%, while that of PLT-8X fell within 5%. For samples with PLT <20×109/L, the CV% of PLT-8X was mostly less than 10% due to the small mean value, which is significantly lower than the imprecision (CV%) of PLT-I (P<0.05). For samples with PLT <15×109/L, the SD value of PLT-I was less than 2, while that of PLT-8X was less than 1; and the range (R) of PLT-I was less than 6, while that of PLT-8X was less than 3, indicating that the results have a very small fluctuation. In the accuracy evaluation, the comparison between the PLT-8X result of BC-6800Plus and that of the flow reference method showed that the results were highly consistent. The expected biases at different clinical medical decision levels (5×109/L, 10×109/L, 20×109/L, and 50×109/L) were 0.7×109/L, 0.5×109/L, 0.0×109/L, and 1.5×109/L, respectively, which can meet the testing needs of hematology laboratories in large hospitals mainly specializing in hematological system disorders. Considering the clinical significance of the low-value PLT detection, this study focused on evaluating the linear performance of the PLT-8X parameters in the 2 low-value PLT ranges (0 to 100 and 0 to 30). It was found that the linear correlation coefficient of low-value PLT was above 0.995, which suggested a good straight line. There was no significant difference between the non-linear coefficient and 0 (P>0.05), indicating that the measurement result of PLT-8X has a good proportional relationship with concentration in the low-value ranges concerned in clinical practice. Considering the clinical significance of the low-value PLT detection, this study also evaluated the LoDs of PLT-I and PLT-8X for low-value PLT. The LoBs of both modes were less than 1×109/L, and the LoDs were 1.98×109/L and 0.89×109/L, respectively. This indicates that at low concentration levels, especially in the detection and quantification of samples with PLT <5×109/L, PLT-8X is superior to PLT-I, and can better meet the clinical needs for low-value PLT detection.
On the whole, the PLT-8X mode has better precision and accuracy in the low-value PLT detection. Compared with the conventional PLT detection mode PLT-I, PLT-8X has better performance, which is helpful for clinicians to accurately quantify peripheral blood PLT in patients with thrombopenia-related diseases, and can better meet the usage needs of clinical laboratories, thereby more effectively assisting doctors in making accurate clinical decisions, and laying a solid foundation for clinically reducing the threshold for PLT transfusion (14). Moreover, this analyzer features a speed up to 200 samples/hour for CD detection and 120 samples/hour for CDR detection, which can fully meet the detection needs for sample quantity in most large or super large laboratories and for accuracy in the low-value PLT detection in laboratories of hospitals specializing in hematonosis.
Acknowledgments
Funding: None.
Footnote
Reporting Checklist: The authors have completed the MDAR reporting checklist. Available at https://atm.amegroups.com/article/view/10.21037/atm-22-1501/rc
Data Sharing Statement: Available at https://atm.amegroups.com/article/view/10.21037/atm-22-1501/dss
Conflicts of Interest: All authors have completed the ICMJE uniform disclosure form (available at https://atm.amegroups.com/article/view/10.21037/atm-22-1501/coif). The authors have no conflicts of interest to declare.
Ethical Statement: The authors are accountable for all aspects of the work in ensuring that questions related to the accuracy or integrity of any part of the work are appropriately investigated and resolved. This study was conducted in accordance with the Declaration of Helsinki (as revised in 2013). This study was approved by the ethics committee of the First Affiliated Hospital of Soochow University (No. 2022-159). Due to the study’s retrospective nature, the requirement to obtain signed informed consent from the patients was waived.
Open Access Statement: This is an Open Access article distributed in accordance with the Creative Commons Attribution-NonCommercial-NoDerivs 4.0 International License (CC BY-NC-ND 4.0), which permits the non-commercial replication and distribution of the article with the strict proviso that no changes or edits are made and the original work is properly cited (including links to both the formal publication through the relevant DOI and the license). See: https://creativecommons.org/licenses/by-nc-nd/4.0/.
References
- Wu J, Heemskerk JWM, Baaten CCFMJ. Platelet Membrane Receptor Proteolysis: Implications for Platelet Function. Front Cardiovasc Med 2020;7:608391. [Crossref] [PubMed]
- Franco AT, Corken A, Ware J. Platelets at the interface of thrombosis, inflammation, and cancer. Blood 2015;126:582-8. [Crossref] [PubMed]
- Kamath V, Sarda P, Chacko MP, et al. Pseudothrombocytopenia observed with ethylene diamine tetra acetate and citrate anticoagulants, resolved using 37°C incubation and Kanamycin. Indian J Pathol Microbiol 2013;56:306-8. [Crossref] [PubMed]
- Wang L, Wang H, Liang H, et al. Giant Platelets Induce Double Interference in Complete Blood Count. Ann Clin Lab Sci 2019;49:554-6. [PubMed]
- Wu W, Guo Y, Zhang L, et al. Clinical utility of automated platelet clump count in the screening for ethylene diamine tetraacetic acid-dependent pseudothrombocytopenia. Chin Med J (Engl) 2011;124:3353-7. [PubMed]
- Harrison P, Ault KA, Chapman S, et al. An interlaboratory study of a candidate reference method for platelet counting. Am J Clin Pathol 2001;115:448-59. [Crossref] [PubMed]
- International Council for Standardization in Haematology Expert Panel on Cytometry. Platelet counting by the RBC/platelet ratio method. A reference method. Am J Clin Pathol 2001;115:460-4. [Crossref] [PubMed]
- Lozano M, Mahon A, van der Meer PF, et al. Counting platelets at transfusion threshold levels: impact on the decision to transfuse. A BEST Collaborative - UK NEQAS(H) International Exercise. Vox Sang 2014;106:330-6. [Crossref] [PubMed]
- Lozano M, Cid J. Consensus and controversies in platelet transfusion: trigger for indication, and platelet dose. Transfus Clin Biol 2007;14:504-8. [Crossref] [PubMed]
- Kunz D. Possibilities and limitations of automated platelet counting procedures in the thrombocytopenic range. Semin Thromb Hemost 2001;27:229-35. [Crossref] [PubMed]
- Kim HY, Bang SH, Cho D, et al. Performance evaluation of platelet counting of Abbott Alinity hq and Sysmex XN-9000 automated hematology analyzer compared with international reference method. Int J Lab Hematol 2021;43:387-94. [Crossref] [PubMed]
- Zandecki M, Genevieve F, Gerard J, et al. Spurious counts and spurious results on haematology analysers: a review. Part I: platelets. Int J Lab Hematol 2007;29:4-20. [PubMed]
- McFarlane A, Aslan B, Raby A, et al. Critical values in hematology. Int J Lab Hematol 2015;37:36-43. [Crossref] [PubMed]
- Marwaha N, Sharma RR. Consensus and controversies in platelet transfusion. Transfus Apher Sci 2009;41:127-33. [Crossref] [PubMed]
(English Language Editor: J. Jones)