Intrinsic network changes associated with cognitive impairment in patients with hearing loss and tinnitus: a resting-state functional magnetic resonance imaging study
Introduction
Hearing loss affects 6–8% of the global population and has become the third leading cause of years lived with disability (1,2). Sensorineural hearing loss accounts for approximately 90% of all hearing loss cases (3) and is often accompanied by tinnitus (4). Many epidemiological studies have confirmed that hearing loss and tinnitus are closely associated with the progression of cognitive impairment (CI) and may even lead to dementia (5,6).
Tinnitus has a complex relationship with hearing loss, both involving auditory processing disorders. One theory is widely accepted as the cause of tinnitus development: hearing loss is the deprivation of the auditory pathway stimulation, and tinnitus may be the result of the compensation mechanism for hearing loss (7). These mechanisms involve an increase in spontaneous activity and/or a decrease in inhibition in the central auditory pathway (8). Lee et al. found that hearing loss and tinnitus severity significantly contributed to the developing cognitive impairment (9).
Although several authors believe that the progression of hearing loss and tinnitus toward CI is related to brain structural and functional abnormalities, the underlying mechanism remains unclear (10). Jafari et al. proposed the cognitive load and common cause hypotheses to explain the mechanism underlying the coexistence of hearing loss and CI (11). Several authors have also found that abnormal changes in various brain areas of patients with hearing loss or tinnitus were associated with the occurrence of CI, such as reduced prefrontal cortex volume (12), reduced temporal activity, increased frontal activity (13), and hyperactive limbic system and cingulate cortex (14,15). Previous studies have reported significant differences between hearing loss and tinnitus and hearing loss without tinnitus in not only sensory-related brain regions but also cognition-related brain regions, such as the dorsolateral prefrontal cortex (16,17). However, such studies did not recruit hearing loss and tinnitus patients with CI, which limits specific interpretations on the neural mechanisms underlying the progression from hearing loss and tinnitus to CI. Therefore, in this study, we recruited hearing loss and tinnitus patients with and without CI to explore the neural mechanisms underlying CI in hearing loss and tinnitus.
Functional magnetic resonance imaging (fMRI) can sensitively show structural and functional brain changes. Compared to task-state fMRI, resting-state functional MRI (rs-fMRI) requires less patient compliance and can directly reflect spontaneous neural activity and functional intensity (18). Independent component analysis (ICA) is a functional network connection analysis method that can be applied to resting-state functional magnetic resonance imaging (rs-fMRI) data. It allows for hypothesis-free and observer-independent measures of intrinsic connectivity patterns (19). Cognitive processes involve multiple brain networks and different aspects of cognitive tasks, and their mechanisms can be more clearly explained by studying the whole brain. Therefore, we focused on changes in whole-brain intrinsic connectivity patterns in hearing loss and tinnitus patients with CI to understand the neural mechanism of CI in hearing loss and tinnitus. Based on the studies mentioned above on hearing loss and tinnitus, we hypothesized that hearing loss and tinnitus patients with CI exhibit distinct brain network alterations. We present the following article in accordance with the MDAR reporting checklist (available at https://atm.amegroups.com/article/view/10.21037/atm-22-2135/rc).
Methods
Participants
A total of 47 hearing loss and tinnitus patients from Beijing Tongren Hospital were enrolled from May 2020 to May 2021. The inclusion criteria for patients were as follows: (I) aged over 18 years; (II) right-handed Han Chinese; (III) bilateral sensorineural hearing loss and high-pitched hearing loss (≥2 kHz) above 25 dB HL in both ears; and (IV) idiopathic tinnitus. The exclusion criteria were as follows: (I) a history of associated ear diseases, such as hyperacusis, otosclerosis, and Ménière’s disease; (II) other neurological diseases or preexisting mental illnesses; and (III) a history of associated brain diseases confirmed by conventional magnetic resonance imaging (MRI). All participants completed the Montreal Cognitive Assessment (MoCA) to assess the degree of CI. According to the MoCA score, 24 patients were categorized into the CI group (MoCA score <26), and 23 patients were categorized into the cognitive normality (CN) group (MoCA score ≥26) (20). A total of 20 healthy volunteers (MOCA score ≥26) were recruited as healthy controls (HCs). Age, sex, and education were matched among the 3 groups. The inclusion criteria for HCs were: (I) adults; (II) right-handed; and (III) normal hearing assessed by otological tests. The same exclusion criteria above also applied to HCs.
The study was conducted in accordance with the Declaration of Helsinki (as revised in 2013). The experimental procedures were approved by the institutional review board of Beijing Tongren Hospital, Capital Medical University (No. TRECKY2013-KS-23). Written informed consent was obtained from all participants before the study.
Image acquisition
Images were acquired on a 3.0T MRI scanner (General Electric Medical Systems, Milwaukee, WI, USA). During scanning, all subjects were asked to lie on the examination bed and remain as still as possible with their eyes closed and not thinking of anything in particular. A matched 8-channel phase-array head coil was used, with earplugs and foam padding to reduce scanner noise and head motion. Images were acquired parallel to the anterior commissure-posterior commissure line and covered the whole brain. The sagittal three-dimensional T1-weighted sequence comprised the following parameters: repetition time/echo time =8.8/3.5 ms; field of view =240×240 mm2; matrix =256×256; slice thickness =1.0 mm, no gap; flip angle =13°; 176 slices. Rs-fMRI data were acquired using an echo-planar imaging pulse sequence with the following parameters: repetition time/echo time =2,000/35 ms; field of view =240×240 mm2; matrix =64×64; slice thickness =3.00 mm, 1.00 mm gap; flip angle =90°; 180 volumes.
Data preprocessing
For image preprocessing, the first 10 volumes were removed. Slicing timing was corrected, and head motion >2 mm displacement in any direction or an angular rotation greater than 2° for the image was excluded from further analyses. Images were then spatially normalized to the Montreal Neurological Institute template (resampling voxel size =3×3×3 mm3) and spatially smoothed using a Gaussian kernel of 6×6×6 mm3 full width at half maximum.
ICA
ICA was performed using GIFT software version 2.0a (https://www.nitrc.org/projects/gift). The procedure included 3 steps: (I) principal component analysis was performed to reduce the data at the individual level; (II) the InfoMax algorithm was applied to decompose the data into group-independent components; and (III) the time series and spatial maps of the independent components were regular back-reconstructed. At present, there is no consensus on an algorithm for selecting the optimal number of components. In this study, 30 components were extracted from each participant and normalized into Z-values to obtain independent spatial activation distribution maps and corresponding time series that reflected intra-functional connectivity (FC). Eleven meaningful resting-state networks (RSNs) were identified by visual inspection as anatomically and functionally classical RSNs (21-24): 2 auditory networks (ANs), a bilateral frontoparietal network (FPN), a dorsal attention network (DAN), an executive control network (ECN), 3 visual networks (VNs), a salience network (SAN), a motor network (MN), 2 sensorimotor networks (SMNs), 2 default mode networks (DMNs), and a ventral attention network (VAN).
Statistical analysis
SPM12 software (https://www.fil.ion.ucl.ac.uk/spm/) was used for data analysis. Differential brain regions were identified among the 3 groups using one-way analyses of variance (ANOVAs) to determine abnormal RSNs (voxel P<0.001, voxel size >10, no correction). For these abnormal RSNs, a post hoc two-sample t-test was used to compare FC values between the CI and CN groups (voxel P<0.001, cluster P<0.05, AlphaSim correction). Brain areas that displayed significant FC differences between the CI and CN groups were selected as regions of interest (ROIs), and the relationships between the mean Z-values of these ROIs and clinical characteristics, including MoCA score, Tinnitus Handicap Inventory (THI) score, disease duration, and degree of hearing loss (maximum high-pitched hearing loss of ≥2 kHz in both ears), were separately assessed using Pearson correlation analyses with a threshold of P<0.05/11 [family-wise error (FWE) corrected].
Results
Demographics and clinical behavior
Table 1 shows the demographic and clinical characteristics of all participants. There were no significant differences in sex, age, or education among the 3 groups. Compared with the CN group, the CI group had significantly lower MoCA scores (P<0.05). Disease duration and THI scores were not significantly different between the CN and CI groups.
Table 1
Demographic | CI (MoCA <26, n=24) | CN (MoCA ≥26, n=23) | HCs (n=20) | F/T-value | P value |
---|---|---|---|---|---|
Gender (M/F) | 18/6 | 18/5 | 11/9 | 3.184 | 0.203a |
Age (years) | 54.13±11.20 | 48.70±13.40 | 48.20±10.35 | 1.789 | 0.175b |
Education (years) | 12.45±2.75 | 12.57±3.26 | 13.8±3.52 | 1.168 | 0.318b |
Duration (years) | 3.70±4.91 | 4.65±6.72 | – | −0.557 | 0.580c |
Hearing loss (dB) | 69.78±22.49 | 73.5±21.79 | – | 0.582 | 0.563c |
THI | 46±23.02 | 51.04±28.15 | – | −0.575 | 0.565c |
MoCA | 23.5±1.47 | 27.13±0.92 | – | −5.932 | 0.000c |
Data are presented as mean ± SD. a, chi-square test; b, one-way ANOVA test; c, two-sample t-test. CI, cognitive impairment group; CN, cognitive normality group; HC, healthy control; MoCA, Montreal Cognitive Assessment; THI, Tinnitus Handicap Inventory; ANOVA, analysis of variance.
Aberrant intrinsic connectivity
The abnormal RSNs identified among the 3 groups were AN, left FPN (LFPN), right FPN (RFPN), DAN, ECN, VN, SAN, MN, SMN, DMN, and VAN (P<0.05, AlphaSim correction). The FCs of the AN, LFPN, RFPN, DAN, ECN, VN, and SAN were significantly different between the CI and CN groups (P<0.05, AlphaSim correction; Figure 1). Compared with the CN group, the CI group showed higher connectivity in the right supramarginal (R_SMG) and the left middle temporal gyrus (L_MTG) within the AN, the left inferior parietal, but supramarginal and angular gyrus (L_IPL) within the RFPN, the right middle occipital gyrus (R_MOG) and the left superior frontal gyrus (L_SFG) within the DAN, the right middle frontal gyrus (R_MFG) within the ECN, the right cuneus (R_cuneus) within the VN, and the left inferior frontal gyrus (L_IFG) within the SAN, as well as lower connectivity in the right superior temporal gyrus (R_STG) within the AN and the R_STG and right superior frontal gyrus (R_SFG) within the LFPN. Table 2 and Figure 2 provide detailed information of the brain regions that showed significantly different intrinsic FC between the CI and CN groups.
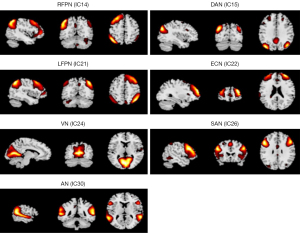
Table 2
RSN | Brain region | Cluster size, mm³ | Peak MNI, mm | Peak T value | |||
---|---|---|---|---|---|---|---|
x | y | z | |||||
CI > CN | AN | R_SM | 94 | 66 | −42 | 15 | 4.2077 |
L_MTG | 43 | −51 | −54 | 15 | 4.0314 | ||
RFPN | L_IPL | 49 | −51 | −51 | 51 | 4.0384 | |
DAN | R_MOG | 41 | 48 | −72 | 24 | 3.8232 | |
R_SFG | 43 | −21 | 39 | 45 | 4.3808 | ||
ECN | R_MFG | 46 | 24 | 60 | 33 | 3.2725 | |
VN | R_cuneus | 94 | 12 | −75 | 27 | 3.9469 | |
CI < CN | SAN | L_IFG | 49 | −45 | 12 | 15 | 3.854 |
AN | R_STG | 58 | 45 | −30 | −3 | −5.038 | |
LFPN | R_STG | 44 | 63 | −18 | 0 | −3.3934 | |
R_SFG | 48 | 30 | 27 | 54 | −4.549 |
Post hoc two-sample t-tests, P<0.05, AlphaSim correction. CI vs. CN. FC, functional connectivity; CI, cognitive impairment group; CN, cognitive normality group; RSN, resting-state network; MNI, Montreal Neurological Institute; AN, auditory network; RFPN, right frontoparietal network; DAN, dorsal attention network; ECN, executive control network; VN, visual network; SAN, salience network; LFPN, left frontoparietal network; R_SM, right supramarginal; L_MTG, left middle temporal gyrus; L_IPL, left inferior parietal, but supramarginal and angular gyrus; R_MOG, right middle occipital gyrus; R_SFG, right superior frontal gyrus; R_MFG, right middle frontal gyrus; R_cuneus, right cuneus; L_IFG, left inferior frontal gyrus, triangle part; R_STG, right superior temporal gyrus.
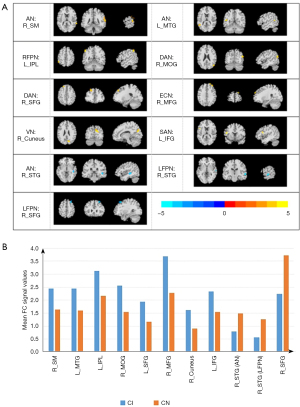
Correlation between the FC of RSNs and clinical characteristics
The Pearson correlation analysis showed that MoCA scores were negatively correlated with the FC values of the R_MFG of the ECN and positively correlated with the FC values of the R_STG of the AN and the R_SFG of the LFPN (P<0.05/11, FWE corrected, Table 3, Figure 3). Disease duration of tinnitus and THI scores were not significant difference between CI and CN.
Table 3
Brian regions | MoCA scores | Degree of hearing loss | Duration | THI | |||||||
---|---|---|---|---|---|---|---|---|---|---|---|
r | P | r | P | r | P | r | P | ||||
R_SMG | −0.386 | 0.007 | 0.014 | 0.927 | 0.235 | 0.112 | 0.246 | 0.096 | |||
L_MTG | −0.320 | 0.029 | −0.064 | 0.667 | −0.160 | 0.235 | −0.136 | 0.363 | |||
L_IPL | −0.230 | 0.120 | −0.096 | 0.520 | 0.084 | 0.572 | 0.248 | 0.093 | |||
R_MOG | −0.389 | 0.007 | −0.106 | 0.479 | −0.030 | 0.840 | −0.243 | 0.100 | |||
R_SFG | −0.131 | 0.378 | −0.023 | 0.877 | −0.134 | 0.370 | 0.100 | 0.502 | |||
R_MFG | −0.512 | 0.000* | 0.078 | 0.600 | 0.029 | 0.848 | −0.104 | 0.485 | |||
R_Cuneus | −0.396 | 0.006 | 0.177 | 0.234 | −0.058 | 0.700 | 0.265 | 0.072 | |||
L_IFG | −0.336 | 0.021 | 0.035 | 0.813 | 0.072 | 0.630 | 0.159 | 0.286 | |||
R_STG(AN) | 0.509 | 0.000* | −0.373 | 0.010 | 0.015 | 0.922 | 0.156 | 0.294 | |||
R_STG(LFPN) | 0.303 | 0.038 | 0.025 | 0.869 | 0.090 | 0.547 | 0.025 | 0.869 | |||
R_SFG | 0.564 | 0.000* | −0.039 | 0.797 | −0.057 | 0.706 | 0.245 | 0.097 |
*, Pearson correlation analyses, P<0.05/11, FWE. FC, functional connectivity; MoCA, Montreal Cognitive Assessment; THI, Tinnitus Handicap Inventory; R_SMG, right supramarginal; L_MTG, left middle temporal gyrus; L_IPL, left inferior parietal, but supramarginal and angular gyrus; R_MOG, right middle occipital gyrus; R_SFG, right superior frontal gyrus; R_MFG, right middle frontal gyrus; R_cuneus, right cuneus; L_IFG, left inferior frontal gyrus, triangle part; R_STG, right superior temporal gyrus; FWE, family-wise error.
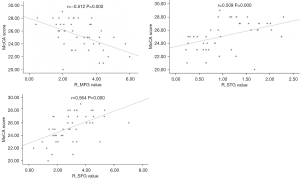
Discussion
In this study, we investigated the resting-state FC of RSNs in hearing loss and tinnitus patients with CI. We found 5 abnormal RSNs that are involved in cognitive processing, particularly attention, memory, and executive function (DAN, SN, LFPN, RFPN, and ECN), and 2 abnormal RSNs that are related to sensory processing (AN and VN). Moreover, we found that the FC of the R_MFG within the ECN was positively correlated with the severity of CI, and the FCs of the R_STG within the AN and the R_SFG within the LFPN were negatively correlated with the severity of CI. These findings provide novel insights into the mechanisms underlying the progression of hearing loss and tinnitus into CI.
We found abnormalities in the AN and VN, which are related to sensory processing. Although under normal conditions the SMG usually belongs to the VN, the FC between the R_SMG and AN was significantly higher in the CI group than in the CN group. The study by Gilley et al. in a group of children with long-term hearing loss showed that the SMG cortex responds to auditory stimuli (25), in line with the theory of cross-modal plasticity proposed by Glick and Sharma (26). In line with our findings, enhanced FC connectivity has been reported in some visually relevant brain regions (like SMG) in the AN (27). In addition, we observed higher FC between the R_cuneus and the VN. The cuneus is a major component of the visual cortex and is involved in the modification and transmission of visual information to the extrastriate cortex, where spatial processing is performed (28). Glick and Sharma showed that when children with hearing loss were presented with a radially modulated visual grating stimulus, additional activation was observed in the temporal cortex, whereas children with normal hearing showed activation of cortical areas associated with the typical processing of visuomotor stimuli (26). Therefore, hearing loss and tinnitus patients with CI may have an overactive sensory cortex in the VN and AN, which results in excess sensory information (29).
Because of the excessive sensory information and the overactive sensory network, the cognitive network of patients with hearing loss may encounter numerous ways to process this information, which could lead to cognitive conflict (4,29). We also found that the FCs of the R_MFG in the ECN and the L_IFG in the SAN were higher in the CI group than in the CN group. The ECN, SAN, and DMN form a tri-network system, which is considered a core cognitive network (30). The ECN is associated with externally directed cognitive tasks, such as self-reflection, working memory, decision making, and other executive functions (31). The middle frontal gyrus (MFG) is activated by cognitive conflict, negative emotions, and pain (29). Abnormal MFG neural activity in the ECN was also reported in a study by Chen et al., which showed that presbycusis disrupts spontaneous activity (32). The SAN is associated with directing attention to the most consistently salient events (31). The inferior frontal gyrus (IFG) constitutes the neuroanatomical network of cognitive function (33). The L_IFG has been shown to be driven by the selection rather than the retrieval of semantic knowledge (34). The enhanced FC of the MFG within the ECN and the IFG within the SAN may be attributed to the high selection and monitoring demands during cognitive tasks (35). In line with this, the MoCA scores were negatively correlated with the FC values of the R_MFG within the ECN.
In CI, the higher FC between the L_IPL within the RFPN and between the R_MOG and L_SFG within the DAN was related to the cognitive abnormalities of hearing loss and tinnitus patients, particularly working memory (17). The IPL, MOG, and SFG are all involved in visual working memory and form the parietal-frontal circuit and occipitoparietal pathway that process spatial information (36). When attention is directed to external stimuli, the activity of the FPN and DAN is increased, and activating the FPN/DAN reduces working memory information capacity (37). The enhanced FC between the left IPL within the RFPN and the right MOG and L_SFG within the DAN in CI indicates that the FPN and DAN are active, which would result in a decrease in working memory capacity. The study by Cai et al. on brain networks involved in working memory found that the connection between the IPL and the FPN was significantly increased when working memory load was enhanced (38). Furthermore, the study by Xue et al. (39) reported that the FC of the L_IPL and L_SFG were increased in patients in the early stage of Alzheimer’s disease. Our study suggests that the predominant effect of hearing loss and tinnitus on cognition is in working memory, where information load leads to a decrease in the processing capacity of the FPN and DAN, which clinically manifests as reduced working memory capacity.
In contrast, the FCs between the R_STG within the AN and LFPN and between the R_SFG within the LFPN were shown to be lower in hearing loss and tinnitus patients with CI than in those without CI. The AN is involved in the processing of auditory information, and the integration of sensory signals is the hallmark of self-awareness (40). The LFPN is thought of as a linguistic network and has been reported to be involved in language-related cognitive processes, such as phonological, semantic, episodic, and working memory (41). The STG is a key area not only for auditory perception but also for visual working memory, decision-making, and emotional processing (42,43). Hearing loss may lead to hypoacusis of the auditory cortex (i.e., the STG), which results in reduced FC between the STG and the AN or LFPN. We hypothesize that CI in hearing loss and tinnitus patients is due to insufficient information being transmitted by the STG to the AN and RFPN to support normal auditory and language-related cognitive functions (44). Ding et al. also found a positive correlation between STG activity and cognitive performance in people with early deafness (45). Therefore, decreased FC of the STG within the AN and LFPN due to hearing loss may lead to reduced cognitive function in hearing loss and tinnitus patients. The right frontal gyrus is active during the early stages of sensory processing (46). Because of the reduction in sensory information, the connection between the SFG and LFPN is weakened, which results in difficulties in the early processing phase of cognition. In hearing loss and tinnitus patients with CI, the reduced FC between the R_STG within the AN and LFPN and between the R_SFG within the LFPN contributes to poor cognitive performance because of reduced semantic information input. We found a positive correlation between MoCA scores and FC of the R_STG within the AN and the R_SFG within the LFPN, which suggests that the higher the severity of the CI, the weaker the FC between these regions.
In summary, patients with hearing loss and tinnitus showed higher inputted perceptual information due to the reorganization of perceptual brain areas (i.e., the R_SMG, STG, and cuneus) within the AN and VN. For the core cognitive networks of the ECN and SAN, the increased FC of the R_MFG in the ECN and the L_IFG in the SAN were suggested to be highly selective in cognitive tasks, which may, in turn, lead to cognitive conflict and decline. These cognitive effects may manifest specifically in working memory because of the higher FC between the L_IPL within the RFPN and the R_MOG and L_SFG within the DAN, as well as the lower working memory capacity of the RFPN and DAN. In contrast, the auditory cortex (R_STG) receives less normal information, and less information is transmitted to the AN and LFPN, which leads to abnormal cognitive function. Moreover, the R_SFG is less active in the early stages of cognitive processing. Taken together, our findings suggest that the disruption of perceptual information processing contributes to the CI of hearing loss and tinnitus patients.
Limitations
The current study has several limitations. First, the sample size of our study was relatively small, so further validation of our findings with a larger sample size is needed. Second, the degree of CI in our cases was mild. Thus, we aim to investigate different subtypes of CI to conduct more detailed examinations using neurophysiological and neuroimaging methods. Third, only patients with bilateral hearing loss and tinnitus were studied. In the future, we plan to further explore whether the mechanisms of CI differ in patients with unilateral hearing loss and tinnitus.
Conclusions
Abnormalities in the FC of cognitive and sensory brain regions within RSNs contribute to the CI of hearing loss and tinnitus patients. Furthermore, the FC of the MFG within the ECN, the SFG within the AN, and the STG within the LFPN may be used to predict the extent of CI in hearing loss and tinnitus patients.
Acknowledgments
Funding: The study was supported by National Natural Science Foundation of China (No. 82071882), Beijing Municipal Natural Science Foundation (No. 7222029) and Science and Technology Planning Project of Beijing Municipal Commission of Education (No. KM202010025019).
Footnote
Reporting Checklist: The authors have completed the MDAR reporting checklist. Available at https://atm.amegroups.com/article/view/10.21037/atm-22-2135/rc
Data Sharing Statement: Available at https://atm.amegroups.com/article/view/10.21037/atm-22-2135/dss
Conflicts of Interest: All authors have completed the ICMJE uniform disclosure form (available at https://atm.amegroups.com/article/view/10.21037/atm-22-2135/coif). The authors have no conflicts of interest to declare.
Ethical Statement: The authors are accountable for all aspects of the work in ensuring that questions related to the accuracy or integrity of any part of the work are appropriately investigated and resolved. The study was conducted in accordance with the Declaration of Helsinki (as revised in 2013). The experimental procedures were approved by the institutional review board of Beijing Tongren Hospital, Capital Medical University (No. TRECKY2013-KS-23). Written informed consent was obtained from all participants before the study.
Open Access Statement: This is an Open Access article distributed in accordance with the Creative Commons Attribution-NonCommercial-NoDerivs 4.0 International License (CC BY-NC-ND 4.0), which permits the non-commercial replication and distribution of the article with the strict proviso that no changes or edits are made and the original work is properly cited (including links to both the formal publication through the relevant DOI and the license). See: https://creativecommons.org/licenses/by-nc-nd/4.0/.
References
- GBD 2016 Disease and Injury Incidence and Prevalence Collaborators. Global, regional, and national incidence, prevalence, and years lived with disability for 328 diseases and injuries for 195 countries, 1990-2016: a systematic analysis for the Global Burden of Disease Study 2016. Lancet 2017;390:1211-59. [Crossref] [PubMed]
- Wilson BS, Tucci DL, Merson MH, et al. Global hearing health care: new findings and perspectives. Lancet 2017;390:2503-15. [Crossref] [PubMed]
- Li L, Chao T, Brant J, et al. Advances in nano-based inner ear delivery systems for the treatment of sensorineural hearing loss. Adv Drug Deliv Rev 2017;108:2-12. [Crossref] [PubMed]
- Knipper M, Van Dijk P, Nunes I, et al. Advances in the neurobiology of hearing disorders: recent developments regarding the basis of tinnitus and hyperacusis. Prog Neurobiol 2013;111:17-33. [Crossref] [PubMed]
- Ruan Q, Chen J, Zhang R, et al. Heterogeneous Influence of Frailty Phenotypes in Age-Related Hearing Loss and Tinnitus in Chinese Older Adults: An Explorative Study. Front Psychol 2020;11:617610. [Crossref] [PubMed]
- Jafari Z, Kolb BE, Mohajerani MH. Age-related hearing loss and tinnitus, dementia risk, and auditory amplification outcomes. Ageing Res Rev 2019;56:100963. [Crossref] [PubMed]
- Savastano M. Tinnitus with or without hearing loss: are its characteristics different? Eur Arch Otorhinolaryngol 2008;265:1295-300. [Crossref] [PubMed]
- Sedley W, Friston KJ, Gander PE, et al. An Integrative Tinnitus Model Based on Sensory Precision. Trends Neurosci 2016;39:799-812. [Crossref] [PubMed]
- Lee SY, Lee JY, Han SY, et al. Neurocognition of Aged Patients With Chronic Tinnitus: Focus on Mild Cognitive Impairment. Clin Exp Otorhinolaryngol 2020;13:8-14. [Crossref] [PubMed]
- Lee HY. Beyond Hearing Loss: Does Tinnitus Cause Cognitive Impairment? Clin Exp Otorhinolaryngol 2020;13:2-3. [Crossref] [PubMed]
- Jafari Z, Kolb BE, Mohajerani MH. Age-related hearing loss and cognitive decline: MRI and cellular evidence. Ann N Y Acad Sci 2021;1500:17-33. [Crossref] [PubMed]
- Wong PC, Ettlinger M, Sheppard JP, et al. Neuroanatomical characteristics and speech perception in noise in older adults. Ear Hear 2010;31:471-9. [Crossref] [PubMed]
- Campbell J, Sharma A. Compensatory changes in cortical resource allocation in adults with hearing loss. Front Syst Neurosci 2013;7:71. [Crossref] [PubMed]
- Vanneste S, Faber M, Langguth B, et al. The neural correlates of cognitive dysfunction in phantom sounds. Brain Res 2016;1642:170-9. [Crossref] [PubMed]
- Leaver AM, Seydell-Greenwald A, Turesky TK, et al. Cortico-limbic morphology separates tinnitus from tinnitus distress. Front Syst Neurosci 2012;6:21. [Crossref] [PubMed]
- Koops EA, de Kleine E, van Dijk P. Gray matter declines with age and hearing loss, but is partially maintained in tinnitus. Sci Rep 2020;10:21801. [Crossref] [PubMed]
- Luan Y, Wang C, Jiao Y, et al. Prefrontal-Temporal Pathway Mediates the Cross-Modal and Cognitive Reorganization in Sensorineural Hearing Loss With or Without Tinnitus: A Multimodal MRI Study. Front Neurosci 2019;13:222. [Crossref] [PubMed]
- Hu J, Cui J, Xu JJ, et al. The Neural Mechanisms of Tinnitus: A Perspective From Functional Magnetic Resonance Imaging. Front Neurosci 2021;15:621145. [Crossref] [PubMed]
- Cole DM, Smith SM, Beckmann CF. Advances and pitfalls in the analysis and interpretation of resting-state FMRI data. Front Syst Neurosci 2010;4:8. [Crossref] [PubMed]
- Hu JB, Zhou WH, Hu SH, et al. Cross-cultural difference and validation of the Chinese version of Montreal Cognitive Assessment in older adults residing in Eastern China: preliminary findings. Arch Gerontol Geriatr 2013;56:38-43. [Crossref] [PubMed]
- Mueller S, Costa A, Keeser D, et al. The effects of methylphenidate on whole brain intrinsic functional connectivity. Hum Brain Mapp 2014;35:5379-88. [Crossref] [PubMed]
- Di X, Biswal BB. Dynamic brain functional connectivity modulated by resting-state networks. Brain Struct Funct 2015;220:37-46. [Crossref] [PubMed]
- Wang C, Qin W, Zhang J, et al. Altered functional organization within and between resting-state networks in chronic subcortical infarction. J Cereb Blood Flow Metab 2014;34:597-605. [Crossref] [PubMed]
- Zhang Q, Wang D, Qin W, et al. Altered resting-state brain activity in obstructive sleep apnea. Sleep 2013;36:651-659B. [Crossref] [PubMed]
- Gilley PM, Sharma A, Dorman MF. Cortical reorganization in children with cochlear implants. Brain Res 2008;1239:56-65. [Crossref] [PubMed]
- Glick H, Sharma A. Cross-modal plasticity in developmental and age-related hearing loss: Clinical implications. Hear Res 2017;343:191-201. [Crossref] [PubMed]
- Ciaramelli E, Grady CL, Moscovitch M. Top-down and bottom-up attention to memory: a hypothesis (AtoM) on the role of the posterior parietal cortex in memory retrieval. Neuropsychologia 2008;46:1828-51. [Crossref] [PubMed]
- Otsuka S, Saiki J. Neural Mechanisms of Memory Enhancement and Impairment Induced by Visual Statistical Learning. J Cogn Neurosci 2020;32:1749-63. [Crossref] [PubMed]
- Castronovo V, Canessa N, Strambi LF, et al. Brain activation changes before and after PAP treatment in obstructive sleep apnea. Sleep 2009;32:1161-72. [Crossref] [PubMed]
- Menon V. Large-scale brain networks and psychopathology: a unifying triple network model. Trends Cogn Sci 2011;15:483-506. [Crossref] [PubMed]
- Seeley WW, Menon V, Schatzberg AF, et al. Dissociable intrinsic connectivity networks for salience processing and executive control. J Neurosci 2007;27:2349-56. [Crossref] [PubMed]
- Chen YC, Chen H, Jiang L, et al. Presbycusis Disrupts Spontaneous Activity Revealed by Resting-State Functional MRI. Front Behav Neurosci 2018;12:44. [Crossref] [PubMed]
- Dolcos F, Wang L, Mather M. Current research and emerging directions in emotion-cognition interactions. Front Integr Neurosci 2014;8:83. [Crossref] [PubMed]
- Thompson-Schill SL, D'Esposito M, Aguirre GK, et al. Role of left inferior prefrontal cortex in retrieval of semantic knowledge: a reevaluation. Proc Natl Acad Sci U S A 1997;94:14792-7. [Crossref] [PubMed]
- Rushworth MF, Kennerley SW, Walton ME. Cognitive neuroscience: resolving conflict in and over the medial frontal cortex. Curr Biol 2005;15:R54-6. [Crossref] [PubMed]
- Ren Z, Zhang Y, He H, et al. The Different Brain Mechanisms of Object and Spatial Working Memory: Voxel-Based Morphometry and Resting-State Functional Connectivity. Front Hum Neurosci 2019;13:248. [Crossref] [PubMed]
- Fornito A, Harrison BJ, Zalesky A, et al. Competitive and cooperative dynamics of large-scale brain functional networks supporting recollection. Proc Natl Acad Sci U S A 2012;109:12788-93. [Crossref] [PubMed]
- Cai W, Ryali S, Pasumarthy R, et al. Dynamic causal brain circuits during working memory and their functional controllability. Nat Commun 2021;12:3314. [Crossref] [PubMed]
- Xue C, Yuan B, Yue Y, et al. Distinct Disruptive Patterns of Default Mode Subnetwork Connectivity Across the Spectrum of Preclinical Alzheimer's Disease. Front Aging Neurosci 2019;11:307. [Crossref] [PubMed]
- Ehrsson HH. The experimental induction of out-of-body experiences. Science 2007;317:1048. [Crossref] [PubMed]
- Leavitt ML, Pieper F, Sachs AJ, et al. A Quadrantic Bias in Prefrontal Representation of Visual-Mnemonic Space. Cereb Cortex 2018;28:2405-21. [Crossref] [PubMed]
- Cao H, Cannon TD. Distinct and temporally associated neural mechanisms underlying concurrent, postsuccess, and posterror cognitive controls: Evidence from a stop-signal task. Hum Brain Mapp 2021;42:2677-90. [Crossref] [PubMed]
- Yang XH, Huang J, Lan Y, et al. Diminished caudate and superior temporal gyrus responses to effort-based decision making in patients with first-episode major depressive disorder. Prog Neuropsychopharmacol Biol Psychiatry 2016;64:52-9. [Crossref] [PubMed]
- Cai Y, Xie M, Su Y, et al. Aberrant Functional and Causal Connectivity in Acute Tinnitus With Sensorineural Hearing Loss. Front Neurosci 2020;14:592. [Crossref] [PubMed]
- Ding H, Qin W, Liang M, et al. Cross-modal activation of auditory regions during visuo-spatial working memory in early deafness. Brain 2015;138:2750-65. [Crossref] [PubMed]
- Alain C, McDonald KL, Kovacevic N, et al. Spatiotemporal analysis of auditory "what" and "where" working memory. Cereb Cortex 2009;19:305-14. [Crossref] [PubMed]