Ability of modern proximal tibial lateral plates to capture posterolateral tibial plateau fracture fragments
Introduction
The posterolateral tibial plateau fractures are not uncommon, accounting for 15–44.2% (1,2) of all proximal tibial fractures. The surgical treatment of posterolateral tibial plateau fractures is fraught with challenges, one of which is the lack of consensus on internal fixations for the posterolateral plateau. Since the periphery of the posterolateral column is blocked by the fibular head, large muscles and ligaments, and the important neurovascular bundles in the popliteal fossa (3,4), accordingly, it is relatedly challenging for surgeons to expose the posterolateral column through posterolateral surgical approaches and place a small posterolateral buttress plate (5-7), and no unanimous and definitive conclusion on treatment strategy can be drawn (8,9). Nevertheless, the extended anterolateral approach with an anterolateral plate remains the most common and least risky method of fixing posterolateral fractures currently (7,10).
With the continuous evolution and improvement of internal fixations, from the non-locking plate to the monoaxial locking plate to the polyaxial locking plate, the fixation effect of fractures is also improving (11-15). However, it is not uncommon for several types of fractures to exceed the current internal fixation system coverage, especially when the fracture is comminuted or located in particular locations (16). Sassoon et al. (17) previously reported that 41.72% of the entire anteroposterior (AP) depth of lateral plateau was unsupported on average, which was located behind the most posterior raft screw by using six different plates. With the advent of 3.5 mm rafting plate systems and 3.5 mm polyaxial plate systems, the coverage for the lateral tibial plateau has increased to some extent. However, there are still some deficiencies in the fixation of posterolateral plateau fractures (9,17), and there is a lack of detailed studies and normative methodology for evaluating the fixation of posterolateral fragments with current plates.
Although it is important to accurately understand the merits and drawbacks of internal fixations for optimum utilization, to our knowledge, no detailed demonstration about quantitative evaluation for the configuration of internal fixations has been proposed. Consequently, this study aimed to put forward an evaluation methodology of internal fixations based on actual fracture cases and to quantitatively assess the fixation effectiveness of four commercially available proximal tibial lateral plate-screw constructs for posterolateral fragments. This study could provide a better understanding of the inadequacy for configurations of current internal fixations and more information about improving the new implant designs. The proposed evaluation methodology may innovate the pre-study phase of the internal fixation design. We present the following article in accordance with the STROBE reporting checklist (available at https://atm.amegroups.com/article/view/10.21037/atm-22-750/rc).
Methods
Subjects
A retrospective search was conducted for the computed tomography (CT) imaging data of patients presenting with the tibial plateau fracture at a large level-I trauma center from January to December 2017. All fractures were identified according to the latest Orthopaedic Trauma Association/Arbeitsgemeinschaft für Osteosynthesefragen Foundation (OTA/AO) (18) and the Luo (19) classification. Patients with insufficient CT information were excluded, especially with an axial slice thickness of more than 3 mm. Additional exclusion criteria included pathological knee fractures, a history of knee surgery, and congenital or acquired malformation of the tibial plateau. A total of 353 tibial plateau fractures were screened, and 144 (40.8%) of them involved (not limited to purely involved) the posterolateral articular surface, which presented separate split fragments or collapsed center (20) in the posterolateral quadrant of the tibial plateau. The study was conducted in accordance with the Declaration of Helsinki (as revised in 2013). The study was approved by Institutional Ethics Committee of Shanghai Sixth People’s Hospital and individual consent for this retrospective analysis was waived.
Digitally segment and reduce fragments
The CT imaging data were downloaded as DICOM files and imported into Mimics software (Version 17.0, Materialise, Leuven, Belgium) to reconstruct fractures digitally. Each fracture fragment was segmented individually and performed to translate and rotate to achieve fracture reduction, using the occlusal relationship of fracture lines and the osseous contours as reference (Figure 1). The operations above were repeated to finish the virtual fracture reduction for each patient.
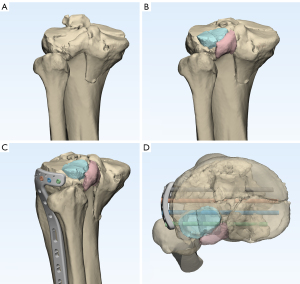
Model internal fixations
Four proximal lateral tibial plate-screw constructs from three leading manufacturers were applied to evaluate in this study (Figure 2):
- 3.5 mm Proximal Tibial Locking Compression Plate (LCP) System (DePuy Synthes, Westchester, NY, USA);
- 3.5 mm Variable Angle (VA)-LCP Proximal Tibial Plate System (DePuy Synthes);
- Periarticular Proximal Tibial Locking Plate (Zimmer, Warsaw, IN, USA);
- Proximal Tibial Plating System (Biomet, Warsaw, IN, USA).
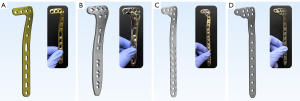
According to the respective manufacturer’s published data and precise physical measurements, the implants were modeled accurately by SolidWorks 2019 Software. The DePuy Synthes VA-LCP and the Biomet plate allowed for both monoaxial locking and polyaxial locking options.
Simulate surgical fixation
The model of implants and the reduced three-dimensional (3D) model of each fracture were imported into the same scene. These implants were then placed respectively on each fracture model at the most suitable position by an experienced surgeon to make sure that the main fragments and as many fragments as possible were fixed firmly to simulate surgical fixation (Figure 1). Significantly, the restriction was that the minimum distance between the fibular head and the plate was not less than 3 mm because of soft tissue attachment around the fibula head.
Evaluate fixation effectiveness
The study concentrated on the positional relationship between intra-articular posterolateral fragments and proximal rafting screws. The evaluation was performed and validated by experienced orthopaedic surgeons. The fragment was considered to be purchased if it was intersected with at least three complete threads of one screw and was deemed to be “captured” if it was purchased by at least two screws (21-23), thereby controlling rotational forces on each fragment. The VA screw was used only if the fragment could not be purchased by a monoaxial screw.
Fracture mapping and heat mapping
The previously reported technology of the 3D fracture mapping (22,24-28) was used to characterize the uncaptured posterolateral fragments by different implants. The virtually reduced fracture models were imported into 3-matic software (Materialise), then were performed to rotate, translate, horizontally flip, and normalize to best match a 3D template of the proximal tibia by aligning specific landmarks—namely, the intercondylar eminence, the lateral and medial plateau, the tibial tubercle and Gerdy’s tubercle. The contours of uncaptured fragments were delineated as enclosed areas by smooth curves on the surface of the 3D template. After that, all these areas were overlaid onto an identical 3D template to produce a spatial fracture map. Lastly, to be more intuitionistic, the graphical superimposition of the uncaptured fragments was transferred as STL-formatted files to E-3D software (Central South University, Changsha, China) to obtain 3D heat maps, which indicated the relative frequency of uncaptured areas for each internal fixation using colors (blue to red: low to high frequency).
Statistical analysis
Patient characteristics and fixation effectiveness were summarized as means and standard deviations or percentages. The difference in the ages of male patients and female patients with posterolateral fractures was conducted using a two-tailed Student t-test. Statistical calculations were performed using SPSS 25.0 (IBM, Armonk, NY, USA). Significance was set at P<0.05. The analysis and characterization of fracture maps and heat maps were descriptive in nature.
Results
Subject
A total of 144 fractures involving the posterolateral articular surface of the tibial plateau were included in the study. Patient demographic and clinical characteristics were summarized in Table 1. The average age of the patient cohort was 49.8 years (range, 16–77 years). The mean age (and standard deviation) was 46.7±12.6 years for males and 52.2±12.1 for females (P<0.05), and there were distinguishing peaks between female patients (51–60 years) and male patients (41–50 years) (Figure 3). The mean number (and standard deviation) of the posterolateral tibial plateau fracture fragments was 1.42±0.66.
Table 1
Items | Results |
---|---|
Patient age†‡ (years) | 49.8 (16–77) |
Total No. of patients§ | 144 (100%) |
Sex‡ | |
Male | 62 (43.1%) |
Female | 82 (56.9%) |
Side of injury§ | |
Left | 92 (63.9%) |
Right | 52 (36.1%) |
Classification§ | |
Schatzker | |
Type II | 31 (21.5%) |
Type III | 37 (25.7%) |
Type V | 63 (43.8%) |
Type VI | 13 (9.0%) |
OTA/AO | |
41.B | 121 (84.0%) |
41.C | 23 (16.0%) |
Three-column¶ | |
PL column | 21 (14.6%) |
PL + L column | 45 (31.3%) |
PL + PM column | 11 (7.6%) |
PL + PM + L column | 3 (2.1%) |
PL + PM + M column | 34 (23.6%) |
PL + PM + L + M column | 30 (20.8%) |
Average No. of the posterolateral tibial plateau fracture fragments* | 1.42±0.66 |
†, the values are given as the mean, with the range in parentheses; ‡, the mean age (and standard deviation) of the 144 patients were 46.7±12.6 years for males and 52.2±12.1 years for females (P<0.05); §, the values are given as the number of patients, with the percentage in parentheses; ¶, PL: posterolateral; L: lateral; PM: posteromedial; M: medial; *, the values are given as the mean and the standard deviation. OTA/AO, Orthopaedic Trauma Association/Arbeitsgemeinschaft für Osteosynthesefragen Foundation.
Evaluate fixation effectiveness
When four implants from three leading manufacturers were utilized to evaluate the fixation effectiveness for posterolateral fragments, there came different outcomes (Table 2). In detail, the DePuy Synthes LCP showed optimal fixation effectiveness (58.3%, 84 out of 144) and could capture an average of 0.95 fragments. Posterolateral fragments of 73 patients (50.7%) were captured by the Zimmer plate, which ranked second. Unexpectedly, the DePuy Synthes VA-LCP and the Biomet plate were corroborated the relatively low percentage of fixation effectiveness, neither exceeding 50% (47.9% and 43.8%, respectively). As indicated in the table, the deficiency may be partially compensated by VA screws, and the capturing rates boosted to 76.4% and 71.5%, respectively, which were about 1.6 times as much as using monoaxial screws. The same trend was also reflected in the number of captured fragments. An interesting but expected result was that the purchasing rates of one screw were high in all four plate-screw constructs.
Table 2
Plate manufacturer | Purchased by two screws (effective fixation) | Purchased by one screw | |||
---|---|---|---|---|---|
No. of cases† | No. of fragments‡ | No. of cases† | No. of fragments‡ | ||
Synthes (LCP) | 84 (58.3%) | 0.95±0.56 | 133 (92.4%) | 1.34±0.66 | |
Synthes (VA-LCP at 0°) | 69 (47.9%) | 0.78±0.58 | 108 (75.0%) | 1.15±0.56 | |
Zimmer | 73 (50.7%) | 0.85±0.55 | 111 (77.1%) | 1.17±0.57 | |
Biomet (at 0°) | 63 (43.8%) | 0.70±0.56 | 129 (89.6%) | 1.31±0.61 | |
Synthes (VA-LCP at 0–15°) | 110 (76.4%) | 1.16±0.56 | 137 (95.1%) | 1.37±0.66 | |
Biomet (at 0–25°) | 103 (71.5%) | 1.09±0.58 | 136 (94.4%) | 1.37±0.63 |
†, the values are given as the number of patients, with the percentage in parentheses; ‡, the values are given as the mean and the standard deviation. LCP, locking compression plates; VA-LCP, variable angle locking compression plate.
Fracture mapping and heat mapping
It was indicated that one shared feature from the graphical superimposition of the uncaptured fragments was that high-frequency areas tended to concentrate around the rim of the posterolateral wall for using whichever plates (Figure 4). The heat maps directly showed the density or frequency of the spatial data relating to uncaptured fragments (Figure 5), similar to the 3D fracture maps. In all groups, the densest frequency area had 79 superimposed fragments in the situation of using the Biomet plating system (Figure 5E). The AP depth of the high-frequency distribution area (red to yellow) for uncaptured fragments differed. In the axial view, the widest AP depth occurred in the Biomet group (screws at 0°), accounting for about 34% of the whole lateral plateau (Figure 5E), whereas the narrowest AP distance was observed when using the DePuy Synthes LCP, which accounted for 24% of the whole lateral plateau (Figure 5A). When the DePuy Synthes VA-LCP (screws at 0°) and the Zimmer plate were performed to experiment, the AP distances were about the same, accounting for 31% and 30% of the whole lateral plateau, respectively (Figure 5B,5D). With the fibula head as a reference, these high-frequency regions were mainly distributed in the posterior 1/2 to 3/4 of its parallel position.
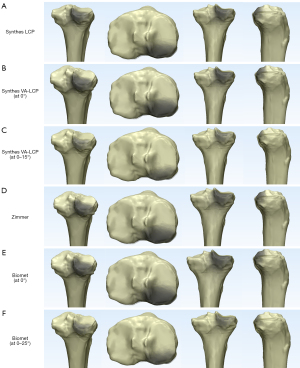
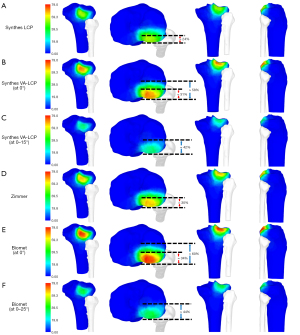
When the VA screws were performed, as shown in the 3D fracture maps, the uncaptured fragments decreased and the coverage areas expanded in the DePuy Synthes VA-LCP group and the Biomet group (Figure 4C,4F). Also, the heat maps’ original red and yellow regions were replaced by green (Figure 5C,5F), representing a significant reduction in the number of uncaptured fragments in the area. The entire AP distances of the uncaptured fragments were reduced respectively from 58% to 42% and 60% to 44% of the whole lateral plateau due to the participation of VA screws in the DePuy Synthes VA-LCP group and the Biomet group.
VA screws
For the DePuy Synthes VA-LCP and the Biomet plate, when monoaxial screws could not purchase the targeted fragment, we tried to apply the VA screw at an appropriate angulation. Different plates and different locations of raft screws showed their relatively high-frequency usage in a disparate range of angles (Figure 6). For the DePuy Synthes VA-LCP, the high-frequency angles of the fourth (posterior-most) raft screw were ranging from 6° to 14°, while the range from 8° to 15° was dominant in the use of the VA manner for the third raft screw. For the Biomet plate, the application of the VA manner for the third and the fourth raft screws varied considerably from 8° to 17° and 1° to 8°, respectively. The most frequently used angles were at 13° and 14° for the third raft screw and at 6° for the fourth raft screw. It’s worth noting that the angle options ranging from 18° to 25° were utterly unutilized in this study.
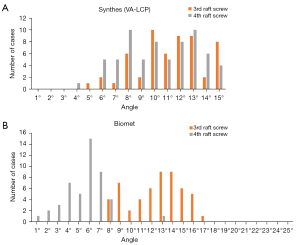
Discussion
The most important purpose of this study was to propose an innovative evaluation method for internal fixations. The method was based on actual fractures to achieve a quantitative evaluation of the fixation effectiveness of implants. Sassoon et al. (17) demonstrated a method to evaluate the configurations of internal fixation systems for proximal tibial fractures through synthetic sawbone models. Compared to their work, the avenue we introduced was based on large quantities of actual fracture cases, which enabled us to draw a more reliable and universal conclusion. Several studies (21,23,29) reported a two-dimensional method to assess the fixation effectiveness of the targeted plates for tibial plateau fractures or pilon fractures. The method of these studies was placing the plates on the cadaveric bone or sawbones and using CT scans to project the location of screws. A slice of axial CT images at a specific distance below (in tibial plateau fracture study) or above (in pilon fracture study) the articular surface was compared with a two-dimensional fracture line map. In other words, this was merely an assessment of the position relationship between the screw and the fracture line on an axial image. Compared to their work, the method in our study was placing each modeled plate-screw construct on virtually reduced 3D fracture models at a suitable position. The position of the implant changed with different fracture morphology to simulate actual surgery. Therefore, the 3D digital method in our study is more reasonable, accurate and valuable in internal fixation evaluation. Furthermore, combined with a qualitative evaluation of fracture maps and heat maps, the purchasing analyses could be further elaborated to the location and distribution of certain fragments missed by the targeted implants, which allowed results to be visualized. The detailed statistics and map demonstration provided by this methodology had certain realistic directive significance in the improvement and innovation of the implant designing for manufacturers. Besides, this study also highlighted the importance of collaboration between surgeons and manufacturers in the design of implants in the future.
The current internal fixations were insufficient in capturing posterolateral fragments and may necessitate additional screws or implants. It is undeniable that with the continuous improvement of the internal fixations, the clinical follow-up outcomes of the fixation of the current lateral proximal tibial plates are relatively satisfactory. However, these plates do not capture all posterolateral fragments well. Fracture maps and heat maps demonstrated that the uncaptured areas tended to locate around the rim of the posterolateral wall of the tibial plateau and distributed in the posterior 1/2 to 3/4 of the fibula head’s parallel position (Figures 4,5). Without a suitable or well-designed internal fixation (16,17,21) and with the block of the special anatomical structures (3,4), the exposure of posterolateral tibial plateau is difficult in the surgery, and the reduction and fixation of posterolateral fragments are challenging for orthopedists. Therefore, it is noteworthy that a combination of plates or a design-improved plate should be used to increase the possibility of capturing posterolateral fragments if the fragment locates in the high-frequency uncaptured region mentioned above. Kim et al. (16) invented a rim plate supplemented for the lateral plate, which can wrap up the posterolateral split fragments and provided multiple rafting screws. Yi et al. (30) reported a new fixation, “Barrel Hoop plate”, to treat the posterolateral depression fracture, and the technique prevented the posterolateral aspect reduction loss. A biomechanical study by Zhang et al. (31) investigated the posterolateral plate provided the strongest resistance for the posterolateral shearing tibial plateau fracture and demonstrated biomechanical superiority.
The use of VA-LCP is both risky and rewarding. As for the DePuy Synthes VA-LCP and the Biomet plate, the fixation effectiveness was relatively poor when monoaxial screws were used, 47.9% and 43.8%, respectively, whereas the rates reached 76.4% and 71.5% when VA screws were utilized. It is undeniable that polyaxial locking plates allow variation in the angle of insertion of each screw, enabling orthopedists to respond to any variation in the bony anatomy, target specific fragments, and improve purchase in higher bone density areas (13,32). Nevertheless, some previous studies presented concerns about them. Tidwell et al. (33) reported an average decrease of 0.4 Nm screw-plate interface strength for every 1° increase in screw-plate angle. Hebert-Davies et al. (34) indicated that two different polyaxial systems showed significant reductions in force of 45% and 34% at extremes of angulation, which may jeopardize the construct and lead to failure in fixation. Consequently, surgeons should significantly evaluate the benefits and risks of using VA screws instead of the pursuit of capturing all fragments. Interestingly, we noticed that the angulation of variable screws from 18° to 25° for the Biomet plate in our study was not applied. Therefore, we predicted that large angulation from 18° to 25° might be rarely used, and this part of the design could be improved in further research and development.
It is essential for posterolateral fragments to achieve anatomical restoration for stable fixation. The result indicated that the fixation effectiveness of proximal tibial lateral plates for posterolateral fragments ranged from 43.8% to 58.3% when using monoaxial screws (Table 2). This study was carried out after the anatomical reduction of the fracture in the virtual surgery scenario. Thus, without good anatomical restoration (35,36) of fracture in the actual surgery, the AP diameter of the tibial plateau could be widened, the effective coverage of implants could be smaller, and the capturing rate of implants for posterolateral fragments could be lower.
Some limitations of this study have to be considered. First, the reconstructed 3D-fracture models had no soft tissue coverage, so it was impossible to reproduce the actual situation during the operation altogether. We could make it as real as possible through the experience of knee trauma specialists and impose a three-millimeter restriction on the placement of modeled plates around the fibular head. Second, this study focused on proximal rafting screws of plates and aimed to evaluate fixation effectiveness for posterolateral intra-articular fragments. However, the interfacial fitness between the whole internal fixations and the osseous surface was not included in this study, which was also one of the significant factors for evaluating the configuration of internal fixations. Lastly, the criterion that the fragment is considered “captured” in this study if at least two screws purchased it, is based on the actual tibial plateau fracture fixation requirement and our clinical experience. However, no such criterion has been defined in the literature. Therefore, biomechanical tests are still needed to specify how many screws and threads should be used as the criterion for determining the capturing.
Conclusions
The proposed new digital methodology was demonstrated feasible and may improve the quantitative evaluation of the implants and optimize the design of implants. The commercially available proximal tibial lateral plate-screw constructs were insufficient in capturing posterolateral fragments, and design-improved or additional implants may be necessitated.
Acknowledgments
Funding: None.
Footnote
Reporting Checklist: The authors have completed the STROBE reporting checklist. Available at https://atm.amegroups.com/article/view/10.21037/atm-22-750/rc
Data Sharing Statement: Available at https://atm.amegroups.com/article/view/10.21037/atm-22-750/dss
Peer Review File: Available at https://atm.amegroups.com/article/view/10.21037/atm-22-750/prf
Conflicts of Interest: All authors have completed the ICMJE uniform disclosure form (available at https://atm.amegroups.com/article/view/10.21037/atm-22-750/coif). The authors have no conflicts of interest to declare.
Ethical Statement: The authors are accountable for all aspects of the work in ensuring that questions related to the accuracy or integrity of any part of the work are appropriately investigated and resolved. The study was conducted in accordance with the Declaration of Helsinki (as revised in 2013). The study was approved by Institutional Ethics Committee of Shanghai Sixth People’s Hospital and individual consent for this retrospective analysis was waived.
Open Access Statement: This is an Open Access article distributed in accordance with the Creative Commons Attribution-NonCommercial-NoDerivs 4.0 International License (CC BY-NC-ND 4.0), which permits the non-commercial replication and distribution of the article with the strict proviso that no changes or edits are made and the original work is properly cited (including links to both the formal publication through the relevant DOI and the license). See: https://creativecommons.org/licenses/by-nc-nd/4.0/.
References
- Xiang G, Zhi-Jun P, Qiang Z, et al. Morphological characteristics of posterolateral articular fragments in tibial plateau fractures. Orthopedics 2013;36:e1256-61. [Crossref] [PubMed]
- Sohn HS, Yoon YC, Cho JW, et al. Incidence and fracture morphology of posterolateral fragments in lateral and bicondylar tibial plateau fractures. J Orthop Trauma 2015;29:91-7. [Crossref] [PubMed]
- Dee M, Sojka JM, Daccarett MS, et al. Evaluation of popliteal artery injury risk with locked lateral plating of the tibial plateau. J Orthop Trauma 2011;25:603-7. [Crossref] [PubMed]
- Heidari N, Lidder S, Grechenig W, et al. The risk of injury to the anterior tibial artery in the posterolateral approach to the tibia plateau: a cadaver study. J Orthop Trauma 2013;27:221-5. [Crossref] [PubMed]
- Solomon LB, Stevenson AW, Baird RP, et al. Posterolateral transfibular approach to tibial plateau fractures: technique, results, and rationale. J Orthop Trauma 2010;24:505-14. [Crossref] [PubMed]
- Solomon LB, Stevenson AW, Lee YC, et al. Posterolateral and anterolateral approaches to unicondylar posterolateral tibial plateau fractures: a comparative study. Injury 2013;44:1561-8. [Crossref] [PubMed]
- Frosch KH, Korthaus A, Thiesen D, et al. The concept of direct approach to lateral tibial plateau fractures and stepwise extension as needed. Eur J Trauma Emerg Surg 2020;46:1211-9. [Crossref] [PubMed]
- Garner MR, Warner SJ, Lorich DG. Surgical Approaches to Posterolateral Tibial Plateau Fractures. J Knee Surg 2016;29:12-20. [PubMed]
- Cho JW, Kim J, Cho WT, et al. Approaches and fixation of the posterolateral fracture fragment in tibial plateau fractures: a review with an emphasis on rim plating via modified anterolateral approach. Int Orthop 2017;41:1887-97. [Crossref] [PubMed]
- Kfuri M, Schatzker J, Castiglia MT, et al. Extended Anterolateral Approach for Complex Lateral Tibial Plateau Fractures. J Knee Surg 2017;30:204-11. [Crossref] [PubMed]
- Schütz M, Südkamp NP. Revolution in plate osteosynthesis: new internal fixator systems. J Orthop Sci 2003;8:252-8. [Crossref] [PubMed]
- Kubiak EN, Fulkerson E, Strauss E, et al. The evolution of locked plates. J Bone Joint Surg Am 2006;88:189-200. [PubMed]
- Haidukewych G, Sems SA, Huebner D, et al. Results of polyaxial locked-plate fixation of periarticular fractures of the knee. J Bone Joint Surg Am 2007;89:614-20. [Crossref] [PubMed]
- Haidukewych GJ, Ricci W. Locked plating in orthopaedic trauma: a clinical update. J Am Acad Orthop Surg 2008;16:347-55. [Crossref] [PubMed]
- Miranda MA. Locking plate technology and its role in osteoporotic fractures. Injury 2007;38:S35-9. [Crossref] [PubMed]
- Kim Y, Yoon YC, Cho JW, et al. Rim Plate Augmentation of the Posterolateral Bare Area of the Tibial Plateau Using a 3.5-mm Precontoured Locking Compression Plate: A Cadaveric Study. J Orthop Trauma 2018;32:e157-60. [Crossref] [PubMed]
- Sassoon AA, Torchia ME, Cross WW, et al. Fibular shaft allograft support of posterior joint depression in tibial plateau fractures. J Orthop Trauma 2014;28:e169-75. [Crossref] [PubMed]
- Meinberg EG, Agel J, Roberts CS, et al. Fracture and Dislocation Classification Compendium-2018 J Orthop Trauma 2018;32:S1-S170. [Crossref] [PubMed]
- Luo CF, Sun H, Zhang B, et al. Three-column fixation for complex tibial plateau fractures. J Orthop Trauma 2010;24:683-92. [Crossref] [PubMed]
- Zhai Q, Hu C, Xu Y, et al. Morphologic study of posterior articular depression in Schatzker IV fractures. Orthopedics 2015;38:e124-8. [Crossref] [PubMed]
- Penny P, Swords M, Heisler J, et al. Ability of modern distal tibia plates to stabilize comminuted pilon fracture fragments: Is dual plate fixation necessary? Injury 2016;47:1761-9. [Crossref] [PubMed]
- Hadad MJ, Sullivan BT, Sponseller PD. Surgically Relevant Patterns in Triplane Fractures: A Mapping Study. J Bone Joint Surg Am 2018;100:1039-46. [Crossref] [PubMed]
- Aneja A, Luo TD, Liu B, et al. Anterolateral distal tibia locking plate osteosynthesis and their ability to capture OTAC3 pilon fragments. Injury 2018;49:409-13. [Crossref] [PubMed]
- Armitage BM, Wijdicks CA, Tarkin IS, et al. Mapping of scapular fractures with three-dimensional computed tomography. J Bone Joint Surg Am 2009;91:2222-8. [Crossref] [PubMed]
- Mellema JJ, Doornberg JN, Dyer GS, et al. Distribution of coronoid fracture lines by specific patterns of traumatic elbow instability. J Hand Surg Am 2014;39:2041-6. [Crossref] [PubMed]
- Molenaars RJ, Mellema JJ, Doornberg JN, et al. Tibial Plateau Fracture Characteristics: Computed Tomography Mapping of Lateral, Medial, and Bicondylar Fractures. J Bone Joint Surg Am 2015;97:1512-20. [Crossref] [PubMed]
- Xie X, Zhan Y, Dong M, et al. Two and Three-Dimensional CT Mapping of Hoffa Fractures. J Bone Joint Surg Am 2017;99:1866-74. [Crossref] [PubMed]
- Xie X, Zhan Y, Wang Y, et al. Comparative Analysis of Mechanism-Associated 3-Dimensional Tibial Plateau Fracture Patterns. J Bone Joint Surg Am 2020;102:410-8. [Crossref] [PubMed]
- McGonagle L, Cordier T, Link BC, et al. Tibia plateau fracture mapping and its influence on fracture fixation. J Orthop Traumatol 2019;20:12. [Crossref] [PubMed]
- Yi Z, Hui S, Binbin Z, et al. A new strategy to fix posterolateral depression in tibial plateau fractures: Introduction of a new modified Frosch approach and a "Barrel hoop plate" technique. Injury 2020;51:723-34. [Crossref] [PubMed]
- Zhang W, Luo CF, Putnis S, et al. Biomechanical analysis of four different fixations for the posterolateral shearing tibial plateau fracture. Knee 2012;19:94-8. [Crossref] [PubMed]
- Cullen AB, Curtiss S, Lee MA. Biomechanical comparison of polyaxial and uniaxial locking plate fixation in a proximal tibial gap model. J Orthop Trauma 2009;23:507-13. [Crossref] [PubMed]
- Tidwell JE, Roush EP, Ondeck CL, et al. The biomechanical cost of variable angle locking screws. Injury 2016;47:1624-30. [Crossref] [PubMed]
- Hebert-Davies J, Laflamme GY, Rouleau D, et al. A biomechanical study comparing polyaxial locking screw mechanisms. Injury 2013;44:1358-62. [Crossref] [PubMed]
- Buckley RE, Moran CG, Apivatthakakul T. AO Principles of Fracture Management. Stuttgart: Georg Thieme Verlag, 2018.
- Singleton N, Sahakian V, Muir D. Outcome After Tibial Plateau Fracture: How Important Is Restoration of Articular Congruity? J Orthop Trauma 2017;31:158-63. [Crossref] [PubMed]