Endoplasmic reticulum stress triggered autophagy and regulated the phenotype transformation of rheumatoid arthritis synovial fibroblasts via the IRE1/JNK pathway
IntroductionOther Section
Rheumatoid arthritis (RA) is a chronic systemic autoimmune disease characterized by synovial tissue inflammation, which can cause the progressive destruction of cartilage and bone (1). Synovial fibroblasts (SFs) (also known as fibroblast-like synoviocytes) are unique cells that populate the intimal lining of the synovium and normally assure the structural as well as dynamic integrity of joints. The key roles of RA synovial fibroblasts (RASFs) in perpetuating inflammation by producing cytokines and contributing to cartilage destruction by producing proteases have been well acknowledged (2). Moreover, RASFs can also evolve to having a unique “aggressive phenotype” that are autonomous and vertically transmitted, which usually viewed as phenotypic changes of RASFs (2,3). These phenotype-transformed RASFs possess characteristics of increased proliferation, inflammatory and aggressive features, which further mediate inflammation and destruction of the joint.
In the inflammatory microenvironment of the rheumatoid joint, RASFs are stimulated by many pro-inflammatory cytokines, especially the tumor necrosis factor alpha (TNF-α). Macrophage-derived TNF-α has been proved to promote proliferation and the production of other pro-inflammatory cytokines and proteases. After stimulation by TNF-α, endoplasmic reticulum (ER) could be induced to synthesize large amounts of proteins and enzymes. When unfolded or misfolded proteins accumulate in the ER and lead to ER stress conditions, the unfolded protein response (UPR) is activated to recuperate the ER function (4). At the initiation of the UPR process, three transmembrane ER stress sensors, inositol requiring enzyme 1 (IRE1), protein kinase R-like endoplasmic reticulum kinase (PERK) and activating transcription factor 6 (ATF6), would be activated after dissociation from glucose-regulated protein 78 (GRP78) and further trigger a series of downstream events to relieve stress (5,6). If ER stress cannot be alleviated in a timely fashion, the cell may undergo apoptosis. It has been reported that autophagy is indeed involved in UPR-associated events.
Autophagy, a lysosome-mediated degradation pathway for organelles and cellular components, has been reported to be activated as a survival mechanism of protecting cells from ER stress-induced death (7). Thus, autophagy is considered to play important roles in various autoimmune diseases (8-10). Studies from other fields have revealed that the three sensors pathway of ER stress regulates autophagy in different ways (11). Researchers have determined that the ER stress markers including GRP78, PERK, and ATF6, as well as the autophagy marker LC3, is elevated in RA synovial tissue compared with osteoarthritis (OA) (12). Other studies have found increased autophagy in RASFs after stimulation with thapsigargin (TG; an ER stress inducer) in vitro (13,14), indicating the active involvement of ER stress in the pathogenesis of RA by evoking autophagy. However, the underlying mechanism remains largely unknown. Here, we aimed to investigate the effect of autophagy activation triggered by ER stress on the phenotype transformation of RASFs and to explore the molecular pathway involved in this process. We present the following article in accordance with the MDAR reporting checklist (available at https://atm.amegroups.com/article/view/10.21037/atm-22-15/rc).
MethodsOther Section
Patient samples
Synovial tissue was obtained from RA (n=12) or OA (n=9) patients during joint replacement surgery (Department of Orthopedics, Peking University First Hospital, Beijing, China). The patients fulfilled the 2010 ACR/EULAR classification criteria for RA or the 1986 ACR classification for knee OA. The study was conducted in accordance with the Declaration of Helsinki (as revised in 2013). This study was approved by the ethics committee of Peking University First Hospital (permit number J201707), and informed consent was taken from all the patients.
Cell culture and treatment
Primary SFs were isolated from synovial tissue and cultured as described elsewhere (15). Briefly, fresh synovial tissue was digested in type I collagenase (Sigma-Aldrich, St. Louis, MO, USA) and trypsin (Gibco, Grand Island, NY, USA) successively, and then cells were cultured with DMEM (Gibco) containing 10% fetal bovine serum (FBS) (Gibco) in a humidified 5% CO2 incubator. Cells from passages 3–6 were used for all experiments. The RASFs and osteoarthritis synovial fibroblasts (OASFs) were treated with 10 ng/mL recombinant human TNF-α (Peprotech, Rocky Hill, NJ, USA) in the presence or absence of inhibitors, which included 10 mM 4-phenylbutyric acid (4-PBA, Sigma-Aldrich), 6 µM SP600125 (Sigma-Aldrich) and 8 mM 3-methyladenine (3-MA, Sigma-Aldrich), for the indicated times.
RNA extraction and real-time quantitative polymerase chain reaction (RT-PCR)
Total messenger RNA (mRNA) was extracted from the synovial tissue, and complementary DNA (cDNA) was prepared with the cDNA Synthesis Kit (Thermo Fisher Scientific, Waltham, MA, USA) according to the manufacturer’s directions. RT-PCR was performed using SYBR Green PCR Master Mix (Applied Biosystems) in triplicated. The relative gene expression level was normalized to the expression of β-actin and calculated using the comparative Ct method (2−∆∆CT method). The primer sequences for the target genes and internal control gene are shown in Table 1.
Table 1
Gene | Protein name | Primers |
---|---|---|
ACTB | β-actin | F: 5'-ggACTTCgAgCAAgAgATgg-3' |
R: 5'-AgCACTgTgTTggCgTACAg-3' | ||
HSPA5 | GRP78 | F: 5'-ggTgCCTACCAAgAAgTCTCAgA-3' |
R: 5'-AgCAggAggAATTCCAgTCAgA-3' | ||
ERN1 | IRE1 | F: 5'-CACAgTgACgCTTCCTgAAAC-3' |
R: 5'-gCCATCATTAggATCTgggAgA-3' | ||
EIF2AK3 | PERK | F: 5'-gTCCggAACCAgACgATgAg-3' |
R: 5'-CCCACTgCTTTTTACCATgATTT-3' | ||
ATF6 | ATF6 | F: 5'-TCCTCggTCAgTggACTCTTA-3' |
R: 5'-CTTgggCTgAATTgAAggTTTTg-3' | ||
MAP1LC3B | LC3 | F: 5'-CCgTCggAgAAgACCTTCAA-3' |
R: 5'-ggTTggATgCTgCTCTCgAA-3' | ||
ATG5 | Atg5 | F: 5'-AgAAgCTgTTTCgTCCTgTgg-3' |
R: 5'-AggTgTTTCCAACATTggCTC-3' | ||
ATG7 | Atg7 | F: 5'-ATgATCCCTgTAACTTAgCCCA-3' |
R: 5'-CACggAAgCAAACAACTTCAAC-3' | ||
ATG12 | Atg12 | F: 5'-TAgAgCgAACACgAACCATCC-3' |
R: 5'-CACTgCCAAAACACTCATAgAgA-3' |
RT-PCR, real-time quantitative polymerase chain reaction.
Western blot
Total protein samples were separated on 10% or 8% SDS-polyacrylamide gels and transferred onto PVDF membranes (Millipore, Billerica, MA, USA). The membranes were blocked in 5% nonfat milk or 5% bovine serum albumin for 1 h at room temperature. Then, the membranes and primary antibodies were incubated overnight at 4 ℃. The primary antibodies included GRP78 (Cell Signaling Technology, Beverly, MA, USA), PERK (Cell Signaling Technology), IRE1 (Cell Signaling Technology), ATF6 (Santa Cruz, Dallas, TX, USA), p-JNK (Cell Signaling Technology), p-PI3K (Cell Signaling Technology), p-AKT (Cell Signaling Technology), LC3B (Cell Signaling Technology), and p62 (Abcam, Cambridge, UK). β-actin (Abcam) was chosen as an endogenous control. Next, the PVDF membranes were incubated with peroxidase-conjugated secondary antibody for 1 h at room temperature. After washing with TBST three times, the proteins were visualized with ECL detection solution (Millipore) using automatic gel imaging analysis system.
Immunohistochemistry
Paraffin-embedded tissue samples from RA and OA patients were deparaffinized in xylene and rehydrated in graded ethanol. Then, the sections were heated in 10 mM sodium citrate buffer (pH 6.0) for epitope retrieval. After inactivating endogenous peroxidase activity with 3% H2O2, the sections were blocked in 5% goat serum (ZSGB-BIO, Beijing, China). Primary antibodies were applied to the slides overnight at 4 ℃. Goat anti-mouse or goat anti-rabbit antibodies (SAB, Greenbelt, MD, USA) were incubated with sections at 37 ℃ for 30 min. The diaminobenzidine (DAB) reagent kit (ZSGB-BIO) was used to detect the positive signals, while hematoxylin was used for nuclear staining.
Analysis of cell proliferation
Cells were planted into 96-well plates and treated with the recombinant human TNF-α in the presence or absence of 4-PBA, SP600125 and 3-MA. After incubated at 37 ℃ with 5% CO2 for 24 h, each well was added with CCK-8 reagents (DOJINDO, Kumamoto, Japan). Then, the incubation continued for 1.5 h under the same conditions. The absorbance at 450 nm was then detected by using an ELISA plate reader.
Analysis of cell migration and invasion
Cells were planted into the upper chamber of the transwell plates (Corning, New York, NY, USA) and treated with the indicated reagents, while DMEM containing 10% FBS was added to the lower compartment as a chemoattractant. After 24 h of incubation, cells remaining on the upper surface of the membrane were removed with a cotton swab. Then, the cells adhering to beneath the membrane were fixed in 4% paraformaldehyde and stained with 0.25% crystal violet. For cell invasion analysis, Matrigel matrix (Corning) was applied to the upper surface of the membrane before cell plantation. The subsequent process was almost the same as that of the cell migration analysis described above.
Lentivirus transfection
The synthesized lentivirus shRNA vector (GeneChem, Shanghai, China) was used to knockdown the target gene ERN1, which is responsible for the expression of the IRE1 protein. The targeting sequence was GAAACTTCCTTTTACCATC. Culture supernatants containing short hairpin RNA-ERN1 (shRNA-ERN1) were added to RASF in the presence of polybrene. The cells were selected by 24 h of incubation with 1.5 µg/mL puromycin. Stable cell lines were verified by western blot.
Statistical analysis
SPSS 22.0, GraphPad Prism 5.0 and ImageJ software were applied to analyze the experimental data. All the experiments were repeated at least 3 times using cells from different patients. Data are presented as the means ± standard deviation (SD). The difference between 2 groups was calculated by Student’s t-test, while differences among 3 or more groups were calculated by one-way analysis of variance. A P value <0.05 was defined as statistically significant.
ResultsOther Section
The signature markers of the ER stress/autophagy pathway were increased in RA synovium
To verify whether the ER stress/autophagy pathway participates in the pathological mechanisms of RA, the expression of the involved proteins was initially examined in the synovium. The immunohistochemical staining of GRP78, PERK, IRE1 and ATF6 was increased to various degrees in the RA synovial lining layer compared with OA synovium, especially GRP78 and IRE1 expression at significantly higher levels in RA patients (Figure 1A,1B). LC3, an autophagy signature protein, was also upregulated in RA synovial tissue. In addition to immunohistochemical analyses, the expression levels of key genes in the ER stress/autophagy pathway in the synovium were also analyzed. As shown in Figure 1, ER stress-related genes, such as HSPA5 (GRP78), ERN1 (IRE1), and EIF2AK3 (PERK), as well as autophagy-related genes, including MAPLC3 (LC3), ATG5 (Atg5), and ATG7 (Atg7), were expressed at significantly higher levels in RA patients than in OA controls, while the expression of ATF6 (ATF6), BECN1 (beclin-1) and ATG12 (Atg12) was not significantly different between the two groups (Figure 1C). Similar results were also observed in the detection of protein expression by western blot (Figure 1D,1E). These results, consistent with previous studies, suggested that the ER stress/autophagy pathway may be involved in RA pathogenesis.
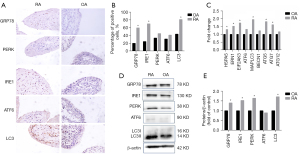
The expression of ER stress/autophagy pathway proteins was upregulated in RASF in vitro
To further explore the molecular mechanism involved in the ER stress/autophagy pathway, SFs were isolated and cultured in vitro. In our study, recombinant human TNF-α was used to induce ER stress, and SFs were incubated with 10 ng/mL TNF-α and analyzed at 0, 3, 6, 9, 12, and 24 h. As expected, the expression of ER stress-related proteins was induced by TNF-α at various degrees in both RASFs and OASFs, except for PERK and ATF6. GRP78 and IRE1 expression was significantly higher in RASF than in OASF. Incubation with TNF-α also evoked the statistically significant upregulation of autophagy in RASFs, as proven by the increased conversion from LC3I to LC3II, as well as the decreased expression of p62, one of the known targets of autophagic degradation (Figure 2). These results indicated that the ER stress/autophagy pathway could be activated by inflammatory mediators in RASF.
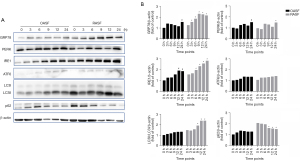
Notably, among the three ER stress sensors, only IRE1 was significantly increased in RASFs after TNF-α treatment in a time-dependent manner. This indicated that the ER stress signals induced by inflammatory mediators in RASFs may be transduced downstream through the IRE1 sensor. Then, we examined several target molecules of IRE1 that have been reported in previous studies, including PI3K/AKT/mTOR, AMPK and JNK. After incubation with TNF-α for the indicated durations, the expression of phosphorylated JNK in RASFs was observed in a similarly time-dependent way to that of IRE1 and LC3II, while such expression pattern was hardly found for other molecules (Figure 3). These results suggested that ER stress caused by inflammatory cytokines may be transduced to activate autophagy through the IRE1/JNK pathway in RASF.

The ER stress/autophagy pathway was involved in the phenotype transformation of RASF
To investigate the effect of the ER stress/autophagy pathway on RASF phenotype transformation, we first investigated the proliferation of RASFs treated with pathway inhibitors. RASFs and OASFs were incubated with TNF-α in the presence of 4-PBA (ER stress inhibitor), sp600125 (JNK inhibitor) or 3-MA (autophagy inhibitor) for 24 h and then assessed by CCK-8 assays. As shown in Figure 4A, TNF-α induced significant proliferation of RASFs compared with OASFs. As expected, ER stress inhibition could reduce the pro-proliferation effect of inflammatory cytokines on RASFs. Similar effects were observed when RASFs were treated with sp600125 or 3-MA. However, no significant inhibitory effects were observed on OASFs, regardless of whether they were treated with 4-PBA or sp600125 (Figure 4A). These results indicated that the ER stress/autophagy pathway might participate in the induction of proliferation by inflammatory cytokines.
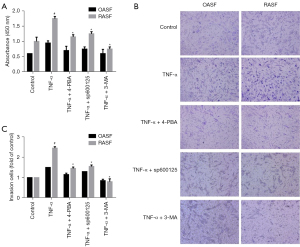
For cell invasion analysis, transwell assays with Matrigel matrix were performed. After incubation with the indicated reagents for 24 h, the cells that invaded through the gel and migrated into lower chamber were counted. RASFs and OASFs were seeded in the upper-chamber with equal amount, and recombinant human TNF-α was used to stimulate and restore SFs phenotypes. As illustrated in Figure 4B, a greater number of invaded cells were observed in RASFs than OASFs, indicating a more aggressive feature of RASFs. Interestingly, the invasive capability of RASFs was significantly weakened when they were treated with ER stress/autophagy pathway inhibitors, including 4-PBA, sp600125 and 3-MA (Figure 4B,4C). Although there was a trend towards more invasive cells in OASFs after TNF-α stimulation, no statistical difference was noted.
Taken together, the aforementioned results suggest that activation of ER stress/autophagy may promote the transformation of the RASF phenotype through the JNK pathway.
IRE1 knockdown impaired RASF phenotype transformation
As mentioned above, IRE1 was the only sensor to be upregulated when ER stress evoked autophagy in RASF. Therefore, we hypothesized that ER stress activated autophagy through the IRE1/JNK pathway and promoted RASF phenotype transformation. Lentivirus-mediated sh-RNA-ERN1 transfection into RASFs was performed to knock down the expression of IRE1. Compared with the negative control, RASFs transfected with sh-RNA-ERN1 significantly reduces IRE1 expression of both mRNA and protein (Figure 5A-5C). IRE1 knockdown also showed a strong trend toward decreased expression of GRP78, PERK and LC3, but did not significantly downregulate the expression of ATF6 (Figure 5A). Functional experiments indicated that the proliferation, migration, and invasion of RASFs were suppressed after IRE1 knockdown. When co-incubated with 3-MA, the suppressive effects were further exacerbated (Figure 6). All these results suggested that in RASFs, IRE1 senses ER stress, triggers downstream autophagy, and eventually promotes the phenotype transformation.
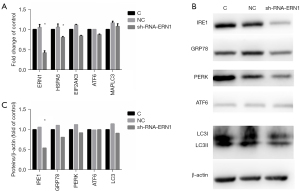
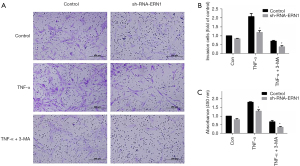
DiscussionOther Section
RA is a systemic autoimmune disease characterized by joint synovitis. It is well recognized that phenotype transformation of RASFs characterized by excessive proliferation and invasion plays a key role in the pathogenesis of synovitis (3), but the underlying mechanism is unknown. The ER stress/autophagy pathway is a novel signaling pathway that regulates cellular homeostasis and has been investigated in many diseases (16). Previous studies have indicated that the ER stress/autophagy pathway may also be involved in the development of RA (13,14,17,18), but the specific mechanism has not been fully elucidated.
In a previous study, researchers extracted synovial tissue microarray data from the GEO database and found that a variety of proteins related to ER stress were significantly increased in synovium from RA patients compared with synovium from OA patients (12). In this study, we initially examined the marker protein expression of the ER stress/autophagy pathway in synovium from RA patients and observed consistent results. Furthermore, we chose to induce ER stress in vitro with recombinant TNF-α instead of commonly used inducers, such as TG and tunicamycin, as TNF-α could better mimic the inflammatory environment of RASF (19-21). In addition, since SFs become fairly quiescent after several passages in vitro, a generally adopted way to restore SF phenotype is by exposing to cytokines like IL-1 and TNF (2). Considering ER stress and autophagy are both dynamic processes, protein expression was examined at multiple time points in our study to explore how the stress signal was transduced downstream. Remarkably, we observed a similar time-dependent changes in the expression of stress sensor IRE1 and the downstream signaling factor JNK under inflammatory stimulation, hinting that the IRE1/JNK pathway is very likely to be involved in autophagy activation in RASF.
Indeed, autophagy triggered by the IRE1/JNK pathway has been reported to be involved in other diseases, but never in RA. In a study focused on SK-N-SH neuroblastoma (22), researchers found that autophagy was decreased in IRE1-knockdown and JNK inhibitor-treated cells, suggesting that the IRE1/JNK pathway is necessary for ER stress-induced autophagy. Another study reported that IRE1 silencing or treatment with the JNK inhibitor sp600125 suppressed drug-induced autophagy activation in H28 and H2452 mesothelioma cells (23). Since RASFs display some tumor-like features, these studies in oncology may support our conclusions to some extent. Further research is needed to determine which autophagy-related proteins are affected when the IRE1/JNK pathway triggers autophagy.
Besides IRE1, other two classic ER stress sensors PERK and ATF6, have also been reported to be vital in autophagy activation (24,25). In cardiomyocytes treated with LPS to induce ER stress (26), decreased autophagy and increased cell death were observed in PERK-knockout cells. In our study, although the expression of PERK and ATF6 was higher in RA synovium than in OA synovium, the increase in both PERK and ATF6 expression after TNF-α incubation in vitro was not significant. This suggested that PERK and ATF6 might be less involved in ER stress-triggered autophagy in RASFs. Consequently, IRE1 was chosen for subsequent research. Previous studies have focused on the connection between the ER stress/autophagy pathway and cell death (27). The ER stress/autophagy pathway may also participate in other physiological processes. In this study, we examined the proliferation, invasion, and migration of RASFs when the ER stress/autophagy pathway was inhibited. As expected, RASF phenotypic transformation was suppressed after IRE1 knockdown or treatment with the autophagy inhibitor 3-MA.
We showed that autophagy induction by ER stress was more pronounced in RASFs than in OASFs, which is consistent with previous data (13,14), indicating the important roles of ER stress/autophagy pathways in RA pathogenesis. Unlike the previous studies, we used TNF-α instead of TG to induce ER stress, which could be a better mimicry of the inflammatory microenvironment and largely maintain the original features of RASFs. Thus, our study again suggested the clinical importance of TNF inhibitors in RA therapy. Besides, an increasing number of studies have summarized the potentiality of ER stress pathways and autophagy pathways as therapeutic targets in RA (8,28). Inhibition of both ER stress [by inhibitors of IRE1a (STF-083010), PERK (salubrinal), or proteasome (bortezomib and MG132)] and autophagy (by chloroquine or 3-methyladenine) pathways abrogated the viability of RASFs, thereby suggesting the therapeutic importance of these two pathways in targeting apoptosis-resistant RASFs (28). Our study firstly reported that IRE1/JNK pathway may be involved in the ER stress-autophagy pathways of RASFs. This will further improve our knowledge on the pathogenesis of this inflammatory arthritis, and consequently facilitate the future therapeutic evolution to regulating the ER stress-IRE1/JNK-autophagy axis in RA.
ConclusionsOther Section
The ER stress/autophagy pathway was strongly activated in RA synovium and RASFs. ER stress triggered autophagy and regulated the phenotype transformation of RASFs via the IRE1/JNK pathway, suggesting the ER stress/autophagy pathway played important roles in the pathological process of RA synovitis.
AcknowledgmentsOther Section
Funding: This research was supported by the National Natural Science Foundation of China (Nos. 81771740 and 82000060).
FootnoteOther Section
Reporting Checklist: The authors have completed the MDAR reporting checklist. Available at https://atm.amegroups.com/article/view/10.21037/atm-22-15/rc
Data Sharing Statement: Available at https://atm.amegroups.com/article/view/10.21037/atm-22-15/dss
Conflicts of Interest: All authors have completed the ICMJE uniform disclosure form (available at https://atm.amegroups.com/article/view/10.21037/atm-22-15/coif). YF reports funding received from National Natural Science Foundation of China (No. 82000060) to support this study. ZZ reports funding received from National Natural Science Foundation of China (No. 81771740) to support this study. The other authors have no conflicts of interest to declare.
Ethical Statement: The authors are accountable for all aspects of the work in ensuring that questions related to the accuracy or integrity of any part of the work are appropriately investigated and resolved. The study was conducted in accordance with the Declaration of Helsinki (as revised in 2013). This study was approved by the ethics committee of Peking University First Hospital (permit number J201707), and informed consent was taken from all the patients.
Open Access Statement: This is an Open Access article distributed in accordance with the Creative Commons Attribution-NonCommercial-NoDerivs 4.0 International License (CC BY-NC-ND 4.0), which permits the non-commercial replication and distribution of the article with the strict proviso that no changes or edits are made and the original work is properly cited (including links to both the formal publication through the relevant DOI and the license). See: https://creativecommons.org/licenses/by-nc-nd/4.0/.
ReferencesOther Section
- Smolen JS, Aletaha D, Bijlsma JW, et al. Treating rheumatoid arthritis to target: recommendations of an international task force. Ann Rheum Dis 2010;69:631-7. [Crossref] [PubMed]
- Bartok B, Firestein GS. Fibroblast-like synoviocytes: key effector cells in rheumatoid arthritis. Immunol Rev 2010;233:233-55. [Crossref] [PubMed]
- Bottini N, Firestein GS. Duality of fibroblast-like synoviocytes in RA: passive responders and imprinted aggressors. Nat Rev Rheumatol 2013;9:24-33. [Crossref] [PubMed]
- Wickner W, Schekman R. Protein translocation across biological membranes. Science 2005;310:1452-6. [Crossref] [PubMed]
- Oakes SA, Papa FR. The role of endoplasmic reticulum stress in human pathology. Annu Rev Pathol 2015;10:173-94. [Crossref] [PubMed]
- Bettigole SE, Glimcher LH. Endoplasmic reticulum stress in immunity. Annu Rev Immunol 2015;33:107-38. [Crossref] [PubMed]
- Mizushima N, Klionsky DJ. Protein turnover via autophagy: implications for metabolism. Annu Rev Nutr 2007;27:19-40. [Crossref] [PubMed]
- Karami J, Masoumi M, Khorramdelazad H, et al. Role of autophagy in the pathogenesis of rheumatoid arthritis: Latest evidence and therapeutic approaches. Life Sci 2020;254:117734. [Crossref] [PubMed]
- Yin H, Wu H, Chen Y, et al. The Therapeutic and Pathogenic Role of Autophagy in Autoimmune Diseases. Front Immunol 2018;9:1512. [Crossref] [PubMed]
- Chen YM, Chang CY, Chen HH, et al. Association between autophagy and inflammation in patients with rheumatoid arthritis receiving biologic therapy. Arthritis Res Ther 2018;20:268. [Crossref] [PubMed]
- Levine B, Mizushima N, Virgin HW. Autophagy in immunity and inflammation. Nature 2011;469:323-35. [Crossref] [PubMed]
- Yoo SA, You S, Yoon HJ, et al. A novel pathogenic role of the ER chaperone GRP78/BiP in rheumatoid arthritis. J Exp Med 2012;209:871-86. [Crossref] [PubMed]
- Kato M, Ospelt C, Gay RE, et al. Dual role of autophagy in stress-induced cell death in rheumatoid arthritis synovial fibroblasts. Arthritis Rheumatol 2014;66:40-8. [Crossref] [PubMed]
- Shin YJ, Han SH, Kim DS, et al. Autophagy induction and CHOP under-expression promotes survival of fibroblasts from rheumatoid arthritis patients under endoplasmic reticulum stress. Arthritis Res Ther 2010;12:R19. [Crossref] [PubMed]
- Zhou JJ, Ma JD, Mo YQ, et al. Down-regulating peroxisome proliferator-activated receptor-gamma coactivator-1 beta alleviates the proinflammatory effect of rheumatoid arthritis fibroblast-like synoviocytes through inhibiting extracellular signal-regulated kinase, p38 and nuclear factor-kappaB activation. Arthritis Res Ther 2014;16:472. [Crossref] [PubMed]
- Glick D, Barth S, Macleod KF. Autophagy: cellular and molecular mechanisms. J Pathol 2010;221:3-12. [Crossref] [PubMed]
- Lin NY, Stefanica A, Distler JH. Autophagy: a key pathway of TNF-induced inflammatory bone loss. Autophagy 2013;9:1253-5. [Crossref] [PubMed]
- Lin NY, Beyer C, Giessl A, et al. Autophagy regulates TNFα-mediated joint destruction in experimental arthritis. Ann Rheum Dis 2013;72:761-8. [Crossref] [PubMed]
- Yorimitsu T, Nair U, Yang Z, et al. Endoplasmic reticulum stress triggers autophagy. J Biol Chem 2006;281:30299-304. [Crossref] [PubMed]
- Vomero M, Manganelli V, Barbati C, et al. Reduction of autophagy and increase in apoptosis correlates with a favorable clinical outcome in patients with rheumatoid arthritis treated with anti-TNF drugs. Arthritis Res Ther 2019;21:39. [Crossref] [PubMed]
- Rashid HO, Yadav RK, Kim HR, et al. ER stress: Autophagy induction, inhibition and selection. Autophagy 2015;11:1956-77. [Crossref] [PubMed]
- Ogata M, Hino S, Saito A, et al. Autophagy is activated for cell survival after endoplasmic reticulum stress. Mol Cell Biol 2006;26:9220-31. [Crossref] [PubMed]
- Lee J, Sohn EJ, Yoon S, et al. Activation of JNK and IRE1 is critically involved in tanshinone I-induced p62 dependent autophagy in malignant pleural mesothelioma cells: implication of p62 UBA domain. Oncotarget 2017;8:25032-45. [Crossref] [PubMed]
- B'chir W, Maurin AC, Carraro V, et al. The eIF2α/ATF4 pathway is essential for stress-induced autophagy gene expression. Nucleic Acids Res 2013;41:7683-99. [Crossref] [PubMed]
- Kalvakolanu DV, Gade P. IFNG and autophagy: a critical role for the ER-stress mediator ATF6 in controlling bacterial infections. Autophagy 2012;8:1673-4. [Crossref] [PubMed]
- Zou X, Xu J, Yao S, et al. Endoplasmic reticulum stress-mediated autophagy protects against lipopolysaccharide-induced apoptosis in HL-1 cardiomyocytes. Exp Physiol 2014;99:1348-58. [Crossref] [PubMed]
- Wirawan E, Vanden Berghe T, Lippens S, et al. Autophagy: for better or for worse. Cell Res 2012;22:43-61. [Crossref] [PubMed]
- Rahmati M, Moosavi MA, McDermott MF ER. Stress: A Therapeutic Target in Rheumatoid Arthritis? Trends Pharmacol Sci 2018;39:610-23. [Crossref] [PubMed]