Esculentoside A could attenuate apoptosis and inflammation in TNBS-induced ulcerative colitis via inhibiting the nuclear translocation of NF-κB
Introduction
Ulcerative colitis (UC) (1), as a kind of recurrent chronic nonspecific inflammatory disease, is characterized by repeated episodes of stools containing mucous and blood, abdominal pain, and diarrhea (2). Due to its complicated etiology, difficult cure, high recurrence rate, poor prognosis, and increased risk of colon cancer, UC is considered a refractory disease (3,4). Currently, the clinical treatment of UC mainly involves glucocorticoids, 5-aminosalicylic acid, sulfasalazine, mesalazine, and so on, which achieve their therapeutic effect via reducing the intestinal inflammation, promoting intestinal mucosal repair, and improving the intestinal mucosal barrier (5-7). However, these drug treatments cannot effectively inhibit the development of UC, especially in the prevention of recurrence, and long-term use of these drugs often leads to significant side effects (8). For example, non-steroidal anti-inflammatory drugs (NSAIDs) have a great impact on the gastrointestinal tract, and long-term use of glucocorticoids often leads to immune suppression and adrenal cortical suppression (9,10). Considering that multiple cellular signaling pathway, especially NF-κB pathway, oxidative stress, activation of pyrin domain-containing protein 3 (NLRP3) inflammasome and autophagy all take part in the pathogenesis of UC (11), searching safe and effective drugs that can improve inflammatory response and inhibit NF-κB pathway are useful for provide new choice for UC treatment.
To date, a large number of studies have been conducted to seek naturally-sourced drugs for the treatment of UC in order to replace or supplement the available drug options, and lots of naturally-sourced drugs are discovered to exert beneficial influence on UC treatment (12,13). Traditional Chinese medicine (TCM) has the characteristics of multipotency, bidirectional regulation, and fewer adverse reactions (14). It is of great significance to discover new anti-inflammatory drugs derived from natural products with less adverse effects to treat with UC. Esculentoside A (EsA), as the main active ingredient extracted from Euphorphiae saponin, has a wide range of pharmacological effects such as anti-inflammatory, diuretic, and immunological regulation (3,4). Ma et al. showed that EsA can play an inhibitory role in nephritis via anti-inflammatory and pro-apoptotic mechanisms (15). It also exerts an inhibitory effect on various respiratory inflammatory conditions by inhibiting the inflammatory cytokines and signaling pathway-related proteins including the PPAR-γ, NF-κB, and ERK signal pathways and the Nrf-2 pathway (16,17). A study has also demonstrated that EsA suppresses neuroinflammation in the hippocampus of model mice via decreasing the phosphorylation levels of ERK, JNK, and p38 (18). Although EsA exerts an anti-inflammatory effect in various diseases, its function in UC is still unknown.
Often, UC is accompanied by diarrhea, abdominal pain, and abnormal intestinal motor function (3). The biological dysfunction of intestinal smooth muscle cells (SMCs) often leads to abnormal intestinal motility, eventually resulting in abnormal gastrointestinal motility (19). Although the SMCs play the most important role in the progression of UC, most researchers have focused on the intestinal dynamics of SMCs in UC, and studies regarding the apoptosis and inflammation of UC have mainly focused on the intestinal epithelial cells (20). Therefore, we aimed to explore the effects of EsA on the inflammation and apoptosis of SMCs.
In this study, we used lipopolysaccharide (LPS) to treat SMCs to establish the inflammatory cells and 2,4,6-trinitrobenzene sulfonic acid (TNBS) to construct a rat UC model. We then studied the effect of EsA on apoptosis and inflammation in vitro and in vivo, with the aim of providing evidence that EsA could be used as an effective drug for the treatment of UC. To the best of our knowledge, this is the first study concerning the possible beneficial effect of EsA on UC. The findings of our research may offer experimental evidence for further understanding the suppressive effect of EsA on inflammation in UC. We present the following article in accordance with the ARRIVE reporting checklist (available at https://atm.amegroups.com/article/view/10.21037/atm-22-2675/rc).
Methods
Animals
A total of 32 male Wistar rats (180–200 g) were obtained from the Zhejiang Vital River Laboratory Animal Technology Co., Ltd. (Jiaxing, China). We purchased EsA from Shanghai Yuanye Biological Co., Ltd. (Shanghai, China). The animals were raised in plastic cages, the relative humidity of the room was maintained at 50–60%, and the temperature at 20–23 ℃ under 12-hour light/dark cycles. All rats were given free access to water and food. The animal experiments were approved by the Institutional Animal Ethics Committee of Guilin Medical University (No. GLMC202203270), in compliance with Guide for the Care and Use of Laboratory Animals, 8th edition. A protocol was prepared before the study without registration. In this study, the 32 rats were randomly divided into four groups, as follows: (I) sham group (n=8; normal saline); (II) model group (n=8; normal saline + TNBS); (III) model + negative control (NC) group [n=8; normal saline + TNBS + double-distilled H2O (ddH2O)]; and (IV) model + EsA group (n=8, normal saline + TNBS + EsA).
TNBS-induced UC
Rats were anesthetized by intraperitoneal injection of 10% chloral hydrate (0.3 mL/kg) after fasting for 24 hours with free access to water. We induced UC in the rats by gentle and slow intrarectal administration of TNBS (Sigma-Aldrich, St. Louis, MO, USA; 100 mg/kg dissolved in 0.25 mL of 50% ethanol) using a flexible gastric lavage needle inserted 8 cm into the colon. Then, the rats were kept head down for 1 minute and lain on their backs in the cage. The Sham model group was administered with 0.25 mL saline as a control. In the model + EsA group, the rats were intraperitoneally injected with EsA (20 mg/kg, Shanghai Yuanye Biological Co., Ltd.) immediately after the UC model was induced and the rats were sacrificed after 10 days of continuous injection of EsA (21). In the model + NC group, the model rats were administered with an equal volume of saline as a NC.
Isolation of colonic SMCs
Rats were euthanized by CO2 inhalation, abdominal incisions were made vertically, about 10 cm of the colons were quickly removed starting from 2 cm distal to the anus and placed in normal saline, enema irrigation was repeatedly applied, and the serum was obtained for the subsequent measurements. Then, the colons were placed in HEPES-buffered Ringer solution (G-clone, Beijing, China) containing 300 U/mL streptomycin (Sigma-Aldrich, USA) and soaked for 15 minutes. The mucosal and serosa layers were gently scraped from the muscle layer by mechanical erasion until the remaining tissue layer was transparent under the stereomicroscope. The tissue layer was cut up and homogenized, added to digestive fluid (4 mL) containing the 0.1% type II collagenase (Worthington Biochemical Corp., Lakewood, NJ, USA) and 0.01% soybean trypsin inhibitor (Worthington, USA), then incubated at 30 ℃ for 20 minutes, centrifuged, and the supernatant was discarded. The precipitates were again digested by digestive fluid (6 mL) at 30 ℃ for 30 minutes. The partially digested tissues were washed using collagenase-free medium and collected via centrifuging at 1,000 r/min for 5 minutes. After that, the partially digested tissues were resuspended in Dulbecco’s modified Eagle medium (DMEM) and the SMCs were collected via filtration using 100 mesh cell sieves. The SMCs were plated at a concentration of 1×105 cells/mL and cultured with DMEM (Gibco, Grand Island, NY, USA) medium containing streptomycin (200 µg/mL), penicillin (Sigma-Aldrich, 200 U/mL), amphotericin B (Sigma-Aldrich, 2.5 µg/mL), gentamycin (Sigma-Aldrich, 100 µg/mL), and 10% fetal bovine serum (FBS) (HyClone, Logan, UT, USA) at 37 ℃ with 5% CO2. The cell viability was detected using the Trypan blue assay (Thermo Fisher Scientific, Waltham, MA, USA; 0.4%, w/v). The cells were cultured for 24 hours, then the medium was replaced and the non-adherent cells were discarded. After that, the DMEM medium was replaced every 3–4 days as needed until reaching about 90% confluence.
Cell treatment
In this study, the in vitro experiments were divided into 3 parts. In the first part, the SMCs cells were pretreated with EsA (0, 4.5, 9, and 18 µM; Topscience, Shanghai, China) for 1 hour and then treated with LPS (Sigma-Aldrich, 1 µg/mL) for 24 hours. In the second part, the SMCs cells divided into four groups: (I) control group; (II) EsA (9 µM) group (cells were treated with EsA for 24 h); (III) LPS group (1 µg/mL 24 h); and (IV) LPS (1 µg/mL) + EsA (9 µM) group (cells were co-treated with LPS and EsA for 24 h). In the third part, the SMCs cells divided into four groups: (I) control; (II) LPS (1 µg/mL) group (cells were treated with LPS for 24 h); (II) LPS (1 µg/mL) + NC group (cells were pretreated with LPS for 24 h and then treated with solvent control); and (IV) LPS (1 µg/mL) + EsA (9 µM) group (cells were pretreated with LPS for 24 h and then treated with EsA for 2 h).
Immunofluorescence staining
Generally, SMCs were fixed, permeabilized, blocked, and then incubated with anti-α-actin [Cell Signaling Technology (CST), Danvers, MA, USA; 6487, 1:100 dilution] or anti-p65 (CST, 69994, 1:400 dilution) antibodies at 4 ℃ overnight. Then, cells were stained with FITC-conjugated immunoglobulin G (IgG; 1:100 dilution) and the nucleus were stained with 4’,6-diamidono-2-phenylindole (DAPI). Lastly, the pictures were captured using a fluorescence microscope (Olympus, Tokyo, Japan) at 400× magnification.
3-(4,5-dimethylthiazolyl-2)-2,5-diphenyltetrazolium bromide (MTT) assay
The total of 3×103 SMCs were seeded into 96-well plates and treated as described previously. The cell viability was tested using a MTT assay kit (Sangon Biotech Co., Ltd.) at 24 hours after drug treatment, according to the manufacturer instructions. Lastly, the MTT in cells were dissolved with 150 µL dimethyl sulfoxide (DMSO; Sigma-Aldrich, USA) and the absorbance at 490 nm was measured using a microplate reader (BMG LABTECH, Offenburg, Germany).
EdU staining
The treated SMCs cells were labeled with 50 µM 5-ethynyl-2’-deoxyuridine (EdU) (Thermo Fisher Scientific, USA) for 2 hours according to the protocol, and the cells nuclei were stained with DAPI. The cells were observed using a fluorescence microscope (Olympus, Japan) with the scale bar set at 50 µm.
Cell apoptosis assay
After treatment as previously described, SMCs were collected and re-suspended with 600 µL biding buffer containing 1% propidium iodide and Annexin V-FITC (Thermo Fisher Scientific, USA), then the cells suspension was incubated away from light for 20 minutes. A flow cytometer (Beckman Coulter, Miami, FL, USA) was used to analyze cell apoptosis.
Nuclear and cytoplasmic separation
Nuclear and cytoplasmic separation were carried out using Nuclear and Cytoplasmic Extraction Reagents (CER; Thermo Fisher Scientific, USA). We used CER I to resuspend the cells and incubate on ice for 10 minutes, and then CER II was added to the tube and incubated on ice for 1 minute. Then, the supernatant (cytoplasmic extract) was obtained by centrifuging the tube at maximum speed and the insoluble (nuclear pellets) fractions were extracted with the nuclear extraction reagent (NER) on ice and stored at −80 ℃ until use.
Western blotting
Briefly, SMCs isolated from the colon were solubilized in radioimmunoprecipitation assay (RIPA) lysate (Beyotime, Nanjing, China) containing protease and phosphatase inhibitors. The concentrations of protein were determined with the bicinchoninic acid (BCA) kit (Beyotime). The proteins were fractionated by sodium dodecyl sulfate polyacrylamide gel electrophoresis (SDS-PAGE) and the separated proteins were transferred to polyvinylidene fluoride (PVDF) membranes. The blots were incubated with the primary antibodies against Bax (CST, 14796, 1:1,000), Bcl-2 (Abcam, ab194583,1:1,000), cleaved caspase-3 (Invitrogen, Carlsbad, CA, USA; PA5-114687, 1:1,000), p65 (CST, 69994, 1:1,000), GAPDH (CST, 5174, 1:1,000), β-actin (CST, 4970, 1:1,000), and Lamin B1 (CST, 13435, 1:1,000) at 4 ℃ for 12 hours, and then incubated with secondary antibody conjugated with horseradish peroxidase (HRP) for 2 hours. Then, the protein bands were visualized by ECL-Plus reagent (Thermo Fisher Scientific, USA) using the Gel Imaging System.
Enzyme-linked immunosorbent assay (ELISA)
The inflammatory cytokines IL-6 and TNF-α in the supernatant and serum were detected via ELISA. All the detection reagents were obtained from R&D Systems (Minneapolis, MN, USA).
Hematoxylin and eosin (H&E) staining and histological score
The colonic tissues were collected and fixed with 4% paraformaldehyde immediately after the rats were sacrificed. The 4-µm colonic tissues sections were stained with H&E solution (H8070, Solarbio, Beijing, China) according to the standard procedures. The images were captured using a microscope (Nikon, Japan) at 200× and 400× magnification, respectively. The TNBS-induced colitis model was assessed using histological scoring system. Briefly, each colon was scored in consideration of (I) the extent of inflammation; (II) the severity of inflammation; and (III) crypt damage. The total score of histology was between 0 and 10. The specific scoring criteria were as described by Xiao and Fattahi et al. (22,23).
TdT-mediated dUTP nick end labeling (TUNEL) staining
Briefly, the colonic tissues on slides (4 µm) were colored using TUNEL probes (Beyotime, Nanjing, China), and the cell nuclei were stained with DAPI. A fluorescence microscope (Olympus, Tokyo, Japan) was used to capture the images at 400× magnification.
Immunohistochemistry (IHC)
Briefly, the colonic tissues on slides (4 µm) were treated according to the instructions. The sections were incubated with a p65 antibody (CST; 1:100) at 4 ℃ overnight, and then incubated with a secondary antibody at room temperature for 2 hours. Immuno-reactivity was visualized by using the 3,3-diaminobenzidine (DAB) chromogen. The sections were stained with hematoxylin and examined under a microscope (Olympus, Japan).
Statistical analysis
The software GraphPad Prism 7.0 (GraphPad Software Inc., San Diego, CA, USA) was used to process all data, and mean ± standard deviation was used to evaluate the data. One-way analysis of variance (ANOVA) was used to compare the multiple groups to evaluate the statistical significance. A P value <0.05 was considered as statistically significant.
Results
The effect of LPS on apoptosis and proliferation in colonic SMCs were partially reversed by EsA
We selected EsA due to it having the highest content of saponins in the Euphorphiae family and the structure is shown in Figure 1A. It has a remarkable curative effect in inflammatory diseases (24). However, the role of EsA in the pathological process of colitis is still unknown. To study the effect of EsA on colitis, the SMCs were isolated from rat colons and identified via immunofluorescence staining. The data showed that the isolated cells expressed α-actin, which is a marker of SMC cells (Figure 1B), indicating that the SMC cells had been isolated from the rat colons successfully. To explore the role of EsA on colitis, the SMCs were pretreated with EsA and then treated with LPS to induce the colitis model in vitro. As the results demonstrated that EsA could effectively improve cell viability which reduced by LPS in a dose-dependent manner, especially for 24 and 48 hours (Figure 1C). Moreover, the SMC cells were treated with LPS or EsA alone or in combination to explore the cell proliferation and apoptosis. The data showed that co-treatment with EsA and LPS could effectively increase the cell viability reduced by LPS (Figure 1D). Furthermore, co-treatment with EsA and LPS could effectively decrease the cell apoptosis increased by LPS in SMCs (Figure 1E,1F). The EdU staining showed the opposite results in cell proliferation (Figure 1G). All the data indicated that the apoptosis and proliferation of SMCs induced by LPS were greatly reversed by EsA.
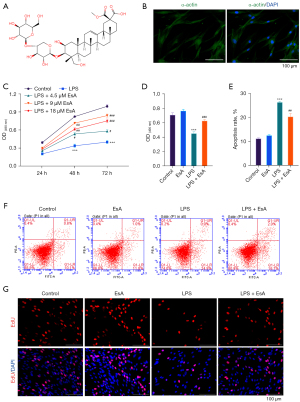
The apoptosis and inflammation induced by LPS were largely attenuated by EsA via inhibiting the nuclear translocation of NF-κB in vitro
To further explore the effect of EsA in apoptosis and inflammation induced by LPS, the cell apoptosis and inflammatory factors including IL-6 and TNF-ɑ were detected. As the results showed that the cell apoptosis induced by LPS were partly reduced by EsA (Figure 2A,2B). In addition, the expression of Bax and cleaved caspase-3 which was increased by LPS were partly decreased by EsA; however, the expression of Bcl-2 showed the opposite results (Figure 2C). Moreover, the secretion of IL-6 and TNF-ɑ promoted by LPS were largely inhibited by EsA (Figure 2D,2E). Furthermore, the western blot and immunofluorescence staining showed that LPS could activate NF-κB and promote its nuclear translocation, and that EsA could inhibit the nuclear translocation of NF-κB induced by LPS (Figure 2F,2G).
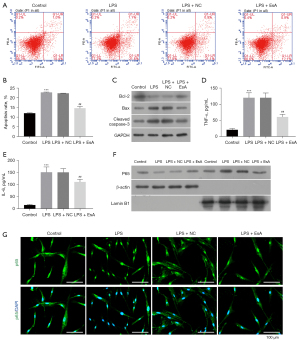
EsA treatment attenuated TNBS-induced UC in rats
The histological data showed that muscularis propria, mucosa, submucosal, and serosa in the colon of the control group were normal in morphology, without inflammatory cells (Figure 3A). Conversely, the TNBS group and TNBS + NC group displayed disrupted colonic architecture, and infiltration of inflammatory cells with depletion of crypt and thickened muscle layer. Nevertheless, the EsA treatment group reduced the severity of TNBS-induced intestinal damage (Figure 3A,3B). Furthermore, the inflammatory cytokines TNF-α and IL-6 in serum of TNBS-induced UC rats were markedly increased when compared with the control group. Moreover, EsA notably inhibited the secretion of TNF-α and IL-6 in TNBS induced UC rats (Figure 3C,3D).
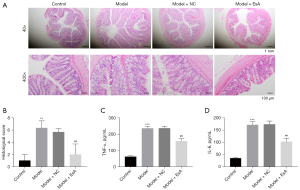
EsA treatment reduced TNBS-induced apoptosis and NF-κB p65 expression in colon tissue
The apoptosis and apoptosis-related proteins in colon tissues were detected via TUNEL staining and western blotting, respectively. The results demonstrated that apoptosis was increased in the TNBS group when compared with the control group, and EsA administration could reduce the apoptosis in the colon tissues of TNBS-induced UC rats (Figure 4A). Besides, the pro-apoptotic proteins including Bax and cleaved caspase-3 increased by TNBS were decreased by EsA in UC rats (Figure 4B), and the expression of anti-apoptotic protein Bcl-2 showed the opposite results (Figure 4B). Moreover, the expression of NF-κB p65 were detected via immunostaining on colonic tissues. The data showed that the expression of NF-κB p65 was up-regulated in the TNBS group when compared with the control group. However, EsA administration resulted in the reduction of the expression of NF-κB p65 compared with the model + NC group (Figure 4C).
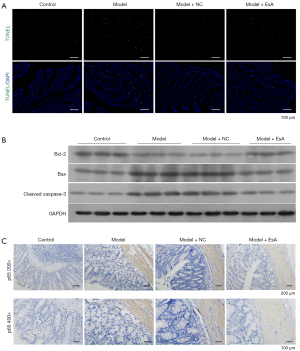
Discussion
The bowel disease UC is a chronic, non-specific, inflammatory disease occurring in the colon and rectum with an extended duration and high recurrence rate (3). To date, studies have shown that the occurrence of UC is mainly caused by environmental deterioration, genetics, immunity, and other factors, especially the abnormal immune response of the body (3,4). Moreover, long-term unhealed UC can increase the risk of colon cancer. Inflammatory response-resulted DNA damage, oxidative stress, microsatellite and chromosomal instabilities can contribute to the UC-related carcinogenesis (25). It is of great significance to seek anti-inflammatory drugs for the treatment of UC. In this study, firstly, we used EsA to treat the LPS-induced SMC cell inflammatory damage and demonstrated that EsA can significantly alleviate the SMC cell inflammatory injury. The symptoms and histological changes of the TNBS/ethanol model were similar to those of human UC, which could simulate the chronic recurrence process of UC, and could be used to evaluate the therapeutic effect of new treatment methods on human UC (26,27). The TNBS-induced UC model has the advantages of simple operation, good reproducibility, short induction time, and long duration of inflammation, which can reflect the complex process of the transformation from acute inflammation to chronic inflammation, and create conditions for the study of inflammation chronization and the exploration of drug therapy (28). Therefore, consistent with the earlier study (29), we also constructed rat UC model via TNBS/ethanol method to explore the therapeutic effect of EsA on UC. After treatment with 20 mg/kg EsA for 10 days, the mucosal inflammatory cell infiltration was significantly decreased, ulcers were healed, necrotic mucosal epithelium was repaired, crypt abscess was alleviated or disappeared, and histological score was significantly reduced, indicating that EsA had a good therapeutic effect on TNBS-induced UC.
Reports have demonstrated that the imbalance between pro-inflammatory cytokines and anti-inflammatory cytokines disrupts the relief of inflammation in the colon which further aggravates the pathogenesis of UC (30). Among these cytokines, TNF-α and IL-6 are considered key pro-inflammatory cytokines that cause intestinal mucosal damage and lead to inflammation (31). Notably, TNF-α could stimulate monocyte macrophages and neutrophils to synthesize IL-6, IL-8, and other cytokines (32). Meanwhile, IL-6, as a pro-inflammatory cytokine with a wide range of effects, is involved in the inflammatory process and can regulate the immune response (33,34). The excessive release of IL-6 often leads to the inflammatory lesions in UC. Therefore, its level could reflect the condition change of UC and be used as a biological indicator of efficacy and prognosis. Ju et al. (21) discovered that EsA could reduce LPS-caused TNF-α, IL-1 and IL-6 production of peritoneal macrophages in mice. Liu et al. (35) reported that EsA inhibited breast cancer progression via suppressing IL-6/signal transducer and activator of transcription 3 (STAT3) pathway. In this study, we also showed that the levels of TNF-α and IL-6 were effectively suppressed after treatment with EsA in LPS-treated SMCs and TNBS-induced UC rat model, indicating that EsA could significantly improve the inflammatory response of mice with UC and the inflammatory response of SMCs induced by LPS.
As a transcription factor regulates the expression of inflammatory factors such as IL-6 and TNF-α, NF-κB is closely related to the pathogenesis and progression of various types of enteritis (36). The main component of NF-κB signaling pathway, p65/p50 dimer, is in a suppressed state when it combines with IκB in cytoplasm (37). Under the stimulus of external signals, IκB is phosphorylated and degraded, and the p65/p50 dimer is released and migrated to the nucleus, thus activating the NF-κB signaling pathway (17). Earlier literature reported that EsA treatment inhibited the activation of NF-κB caused by LPS in kidney tissue of mice (38). Moreover, EsA was discovered to participated in NF-κB nuclear translocation in experimental acute liver injury of mice (17). Here, we also showed that LPS-promoted p65 nucleation was significantly suppressed when treated with EsA, highlighting that EsA played its protective role in UC via regulating NF-κB pathway. In the in vivo experiments, we demonstrated that the up-regulated p65 expression, which was mainly found in the muscularis externa section in the UC group, was also greatly decreased under the treatment of EsA, further confirming that EsA might treat UC by regulating the NF-κB pathway.
The imbalance of Bcl-2 (an anti-apoptotic protein) and Bax (a pro-apoptotic protein) often leads to changes in the apoptotic ability of cells (39,40). The caspase protein family is involved in the initiation and regulation of apoptosis, and caspase-3 is a key enzyme in the activation of various apoptosis-stimulating factors (1). In this study, we showed that EsA could decrease the apoptosis rate of LPS-treated SMCs, suppress the Bax protein level, and increase the protein levels of Bcl-2 and caspase-3, indicating that EsA could greatly inhibit the apoptosis of LPS-induced SMCs. Besides, the similar trends were observed in the in vivo experiments, further confirming that EsA could significantly reduce the apoptosis in UC.
Intestinal mucosal immune is an important part of human immune system (41). There are diffuse lymphoid tissue, isolated lymphoid nodules, collective lymphoid nodules, lymphocytes, macrophages and plasma cells in intestinal digestive tract, which work together to form intestinal mucosal immune system (42). Previous literature reported that the defect or damage of intestinal mucosal immune might be associated with UC (43). Considering that EsA had excellent anti-inflammatory activity, we think that EsA might also have a beneficial effect on inflammation in intestinal mucosal immunity. In the future, we will further explore the possible beneficial influence of EsA in intestinal mucosal immune.
Conclusions
Our study showed that EsA can inhibit the inflammatory response and cell apoptosis by regulating the NF-κB signaling pathway thereby inhibiting inflammatory factors TNF-α and IL-6 expressions in LPS-induced inflammatory cells and the rat UC model, which provided evidence that EsA might be used as a novel therapeutic drug for UC treatment. Due to the pathogenesis of UC is very complex, which involves in multiple inflammatory-related factors, intestinal cell apoptosis and autophagy, as well as intestinal mucosal immune (44), how EsA modulates the nuclear translocation of NF-κB is still unclear, more animal and clinical experiments are still needed to further explore the efficacy and safety of EsA on UC treatment in the future.
Acknowledgments
Funding: The study was funded by the National Natural Science Foundation of China (No. 81960106).
Footnote
Reporting Checklist: The authors have completed the ARRIVE reporting checklist. Available at https://atm.amegroups.com/article/view/10.21037/atm-22-2675/rc
Data Sharing Statement: Available at https://atm.amegroups.com/article/view/10.21037/atm-22-2675/dss
Conflicts of Interest: All authors have completed the ICMJE uniform disclosure form (available at https://atm.amegroups.com/article/view/10.21037/atm-22-2675/coif). The authors have no conflicts of interest to declare.
Ethical Statement: The authors are accountable for all aspects of the work in ensuring that questions related to the accuracy or integrity of any part of the work are appropriately investigated and resolved. The animal experiments were approved by the Institutional Animal Ethics Committee of Guilin Medical University (No. GLMC202203270), in compliance with Guide for the Care and Use of Laboratory Animals, 8th edition.
Open Access Statement: This is an Open Access article distributed in accordance with the Creative Commons Attribution-NonCommercial-NoDerivs 4.0 International License (CC BY-NC-ND 4.0), which permits the non-commercial replication and distribution of the article with the strict proviso that no changes or edits are made and the original work is properly cited (including links to both the formal publication through the relevant DOI and the license). See: https://creativecommons.org/licenses/by-nc-nd/4.0/.
References
- Matsuo J, Haga S, Hashimoto K, et al. Activation of caspase-3 during Chlamydia trachomatis-induced apoptosis at a late stage. Can J Microbiol 2019;65:135-43. [Crossref] [PubMed]
- Baker DM, Folan AM, Lee MJ, et al. A systematic review and meta-analysis of outcomes after elective surgery for ulcerative colitis. Colorectal Dis 2021;23:18-33. [Crossref] [PubMed]
- Kobayashi T, Siegmund B, Le Berre C, et al. Ulcerative colitis. Nat Rev Dis Primers 2020;6:74. [Crossref] [PubMed]
- Kaenkumchorn T, Wahbeh G. Ulcerative Colitis: Making the Diagnosis. Gastroenterol Clin North Am 2020;49:655-69. [Crossref] [PubMed]
- Rowan CR, Boland K, Harewood GC. Ustekinumab as Induction and Maintenance Therapy for Ulcerative Colitis. N Engl J Med 2020;382:91. [Crossref] [PubMed]
- Wu D, Yang Z, Zhao C, et al. Infliximab versus cyclosporine for severe ulcerative colitis refractory to steroids: A protocol for systematic review and meta-analysis. Medicine (Baltimore) 2018;97:e12657. [Crossref] [PubMed]
- Wu B, Tong J, Ran Z. Tacrolimus Therapy in Steroid-Refractory Ulcerative Colitis: A Review. Inflamm Bowel Dis 2020;26:24-32. [Crossref] [PubMed]
- Wehkamp J, Stange EF. Recent advances and emerging therapies in the non-surgical management of ulcerative colitis. F1000Res 2018;7:eF1000 Faculty Rev-1207.
- Guo CG, Leung WK. Potential Strategies in the Prevention of Nonsteroidal Anti-inflammatory Drugs-Associated Adverse Effects in the Lower Gastrointestinal Tract. Gut Liver 2020;14:179-89. [Crossref] [PubMed]
- Oray M, Abu Samra K, Ebrahimiadib N, et al. Long-term side effects of glucocorticoids. Expert Opin Drug Saf 2016;15:457-65. [Crossref] [PubMed]
- Sartor RB. Mechanisms of disease: pathogenesis of Crohn's disease and ulcerative colitis. Nat Clin Pract Gastroenterol Hepatol 2006;3:390-407. [Crossref] [PubMed]
- Habtemariam S, Belai A. Natural Therapies of the Inflammatory Bowel Disease: The Case of Rutin and its Aglycone, Quercetin. Mini Rev Med Chem 2018;18:234-43. [Crossref] [PubMed]
- Zhang C, Jiang M, Lu A. Considerations of traditional Chinese medicine as adjunct therapy in the management of ulcerative colitis. Clin Rev Allergy Immunol 2013;44:274-83. [Crossref] [PubMed]
- Hempen CH, Hummelsberger J. Traditional Chinese medicine (TCM)-what is myth and what is the state of evidence today? Bundesgesundheitsblatt Gesundheitsforschung Gesundheitsschutz 2020;63:570-6. [Crossref] [PubMed]
- Ma H, Zhang X, Zhang X, et al. The effect of esculentoside A on lupus nephritis-prone BXSB mice. Arch Med Sci 2013;9:354-60. [Crossref] [PubMed]
- Ci X, Zhong W, Ren H, et al. Esculentoside A Attenuates Allergic Airway Inflammation via Activation of the Nrf-2 Pathway. Int Arch Allergy Immunol 2015;167:280-90. [Crossref] [PubMed]
- Zhang F, Wang X, Qiu X, et al. The protective effect of Esculentoside A on experimental acute liver injury in mice. PLoS One 2014;9:e113107. [Crossref] [PubMed]
- Yang H, Wang S, Yu L, et al. Esculentoside A suppresses Aβ(1-42)-induced neuroinflammation by down-regulating MAPKs pathways in vivo. Neurol Res 2015;37:859-66. [Crossref] [PubMed]
- Lenti MV, Di Sabatino A. Intestinal fibrosis. Mol Aspects Med 2019;65:100-9. [Crossref] [PubMed]
- Ren MT, Gu ML, Zhou XX, et al. Sirtuin 1 alleviates endoplasmic reticulum stress-mediated apoptosis of intestinal epithelial cells in ulcerative colitis. World J Gastroenterol 2019;25:5800-13. [Crossref] [PubMed]
- Ju DW, Zheng QY, Cao X, et al. Esculentoside A inhibits tumor necrosis factor, interleukin-1, and interleukin-6 production induced by lipopolysaccharide in mice. Pharmacology 1998;56:187-95. [Crossref] [PubMed]
- Xiao HT, Lin CY, Ho DH, et al. Inhibitory effect of the gallotannin corilagin on dextran sulfate sodium-induced murine ulcerative colitis. J Nat Prod 2013;76:2120-5. [Crossref] [PubMed]
- Fattahi N, Abdolahi A, Vahabzadeh Z, et al. Topical phenytoin administration accelerates the healing of acetic acid-induced colitis in rats: evaluation of transforming growth factor-beta, platelet-derived growth factor, and vascular endothelial growth factor. Inflammopharmacology 2022;30:283-90. [Crossref] [PubMed]
- Li Y, Cao Y, Xu J, et al. Esculentoside A suppresses lipopolysaccharide-induced pro-inflammatory molecule production partially by casein kinase 2. J Ethnopharmacol 2017;198:15-23. [Crossref] [PubMed]
- Thorsteinsdottir S, Gudjonsson T, Nielsen OH, et al. Pathogenesis and biomarkers of carcinogenesis in ulcerative colitis. Nat Rev Gastroenterol Hepatol 2011;8:395-404. [Crossref] [PubMed]
- Li Y, Liu Q, Tang JH, et al. Regulatory mechanism of mesalazine on TLR4/MyD88-dependent pathway in mouse ulcerative colitis model. Eur Rev Med Pharmacol Sci 2019;23:6637-44. [PubMed]
- Cai B, Zhou MH, Huang HL, et al. Protective effects of citrulline supplementation in ulcerative colitis rats. PLoS One 2020;15:e0240883. [Crossref] [PubMed]
- Motaghi E, Hajhashemi V, Mahzouni P, et al. Protective Effect of Dizocilpine (MK-801) On TNBS-Induced Experimental Colitis in Mice. Iran J Pharm Res 2019;18:1341-850. [PubMed]
- Tozaki H, Odoriba T, Okada N, et al. Chitosan capsules for colon-specific drug delivery: enhanced localization of 5-aminosalicylic acid in the large intestine accelerates healing of TNBS-induced colitis in rats. J Control Release 2002;82:51-61. [Crossref] [PubMed]
- Yao D, Dong M, Dai C, et al. Inflammation and Inflammatory Cytokine Contribute to the Initiation and Development of Ulcerative Colitis and Its Associated Cancer. Inflamm Bowel Dis 2019;25:1595-602. [Crossref] [PubMed]
- Wang C, Li W, Wang H, et al. Saccharomyces boulardii alleviates ulcerative colitis carcinogenesis in mice by reducing TNF-α and IL-6 levels and functions and by rebalancing intestinal microbiota. BMC Microbiol 2019;19:246. [Crossref] [PubMed]
- Pérez D, Muñoz MC, Molina JM, et al. Eimeria ninakohlyakimovae induces NADPH oxidase-dependent monocyte extracellular trap formation and upregulates IL-12 and TNF-α, IL-6 and CCL2 gene transcription. Vet Parasitol 2016;227:143-50. [Crossref] [PubMed]
- Tanaka T, Narazaki M, Kishimoto T. Interleukin (IL-6) Immunotherapy. Cold Spring Harb Perspect Biol 2018;10:a028456. [Crossref] [PubMed]
- Juchem KW, Gounder AP, Gao JP, et al. NFAM1 Promotes Pro-Inflammatory Cytokine Production in Mouse and Human Monocytes. Front Immunol 2021;12:773445. [Crossref] [PubMed]
- Liu C, Dong L, Sun Z, et al. Esculentoside A suppresses breast cancer stem cell growth through stemness attenuation and apoptosis induction by blocking IL-6/STAT3 signaling pathway. Phytother Res 2018;32:2299-311. [Crossref] [PubMed]
- Sha H, Gu Y, Shen W, et al. Rheinic acid ameliorates radiation-induced acute enteritis in rats through PPAR-γ/NF-κB. Genes Genomics 2019;41:909-17. [Crossref] [PubMed]
- Cai M, Liao Z, Zou X, et al. Herpes Simplex Virus 1 UL2 Inhibits the TNF-α-Mediated NF-κB Activity by Interacting With p65/p50. Front Immunol 2020;11:549. [Crossref] [PubMed]
- Chen DZ, Chen LQ, Lin MX, et al. Esculentoside A inhibits LPS-induced acute kidney injury by activating PPAR-γ. Microb Pathog 2017;110:208-13. [Crossref] [PubMed]
- Zhang Y, Yang X, Ge X, et al. Puerarin attenuates neurological deficits via Bcl-2/Bax/cleaved caspase-3 and Sirt3/SOD2 apoptotic pathways in subarachnoid hemorrhage mice. Biomed Pharmacother 2019;109:726-33. [Crossref] [PubMed]
- Vasquez-Montes V, Rodnin MV, Kyrychenko A, et al. Lipids modulate the BH3-independent membrane targeting and activation of BAX and Bcl-xL. Proc Natl Acad Sci U S A 2021;118:e2025834118. [Crossref] [PubMed]
- Allaire JM, Crowley SM, Law HT, et al. The Intestinal Epithelium: Central Coordinator of Mucosal Immunity. Trends Immunol 2018;39:677-96. [Crossref] [PubMed]
- Xu AT, Lu JT, Ran ZH, et al. Exosome in intestinal mucosal immunity. J Gastroenterol Hepatol 2016;31:1694-9. [Crossref] [PubMed]
- Targan SR, Karp LC. Defects in mucosal immunity leading to ulcerative colitis. Immunol Rev 2005;206:296-305. [Crossref] [PubMed]
- Du L, Ha C. Epidemiology and Pathogenesis of Ulcerative Colitis. Gastroenterol Clin North Am 2020;49:643-54. [Crossref] [PubMed]
(English Language Editor: J. Jones)