Palbociclib regulates the expression of dihydrofolate reductase and the cell cycle to inhibit t (11;14) multiple myeloma
Introduction
Multiple myeloma (MM) is a malignancy of plasma cells (PCs) disease (1). It can produce monoclonal immunoglobulin in the blood and urine leading repeated infections, and impaired renal function (2). Moreover, immunodeficiency and osteolytic bone lesions are often found, which significantly compromises both their longevity and quality of life (2). MM with t (11;14) present in about 20% of patients with newly diagnosed multiple myeloma, but studies examining its prognostic ability have yielded divergent results, and data on outcomes from first-line therapy are lacking. New combinations of novel agents are needed to improve the prognosis of patients with t (11;14) MM.
As a selective inhibitor of cyclin-dependent kinases 4 and 6 (CDK4/6), palbociclib (PAL) has been used to treat various malignancies, including breast cancer, ovarian cancer, hepatocellular carcinoma, colorectal cancers, and so on (3). PAL has been shown to inhibit breast cancer cells by mediating the proteasome and Proteasome Adaptor and Scaffold (ECM29) protein (4). In 2015, PAL was approved by the US Food and Drug Administration (FDA) to treat breast cancer patients combined with other drugs (5)
As an important enzyme in the de novo pathway of purine and thymidine synthesis, dihydrofolate reductase (DHFR) plays an important role in many physiological activities, such as the growth of bacteria and parasites, anti-inflammatory activity, and cancer chemotherapy (6). The inhibition of DHFR can cause disruption of purine and thymidylate biosynthesis and DNA replication, leading to cell death (7). Our previous study demonstrated that PAL could inhibit the expression levels of DHFR and vascular endothelial growth factor (VEGF), thereby killing cancer cells in bladder cancer patients, which indicated that PAL could be considered a broad anticancer drug (8).
Two MM cell lines (U266 and KMS27) and a three-dimensional (3D) spheroid model (9) were used in this study to investigate the effects of PAL on MM. Paclitaxel (PTX) and methotrexate (MTX) were used as controls to evaluate the anticancer effects of PAL on MM. Mode-of-action (MOA) underlying effects of PAL on MM were also investigated. The findings of this investigation might provide useful information for the development of novel therapeutic strategies to treat MM. We present the following article in accordance with the ARRIVE reporting checklist (available at https://atm.amegroups.com/article/view/10.21037/atm-22-2830/rc).
Methods
Compliance with ethical standards
All animal experiments were approved by Shengjing Hospital of China Medical University Institutional Animal Care and Use Committee (No. SHCMU2020010) and complied with institutional guidelines for the care and use of animals. This article does not contain any studies with human participants performed by any of the authors. A protocol was prepared before the study without registration.
Reagents
PAL was purchased from InvivoChem (catalog number: PD-0332991, Libertyville, USA), PTX was purchased from Beyotime (catalog number: SC0213-10mM, Shanghai, China), and MTX was purchased from Selleck (catalog number: CL-14377, Houston, USA). BeyoRT™ complementary DNA (cDNA) kit was purchased from Beyotime (catalog number: D7166, Shanghai, China). Trizol was purchased from Beyotime (catalog number: R0016, Shanghai, China). U266 and KMS27 cell lines were obtained from the biological materials bank of the Shengjing Hospital of China Medical University. Fetal bovine serum (FBS) was purchased from Beyotime (catalog number: C0225, Shanghai, China). BeyoFast™ SYBR Green qPCR Mix (2X) was purchased from Beyotime (catalog number: D7260-25ml, Shanghai, China). The 3-(4,5-dimethylthiazol-2-yl)-2,5-diphenyltetrazolium bromide (MTT) Cell Proliferation and Cytotoxicity Assay Kit (catalog number: C0009S, Shanghai, China), Radioimmunoprecipitation (RIPA) buffer (strong) (catalog number: P0013B, Shanghai, China), and bicinchoninic acid (BCA) assay reagent (catalog number: P0010S, Shanghai, China) were purchased from Beyotime.
Cell culture
The U266 cell line (human MM cell line) was cultured with Roswell Park Memorial Institute (RPMI)-1640 medium supplemented with dialyzed 5% (v/v) FBS and 1% (v/v) non-essential amino acids at 37 ℃ in humidified air with 5% CO2. Another MM cell line, KMS27, was cultured with 85% RPMI 1640 + 15% FBS at 37 ℃ in humidified air with 5% CO2.
3D spheroid model
The 3D spheroid model using U266 and KMS27 was constructed according to previous reports (9). Briefly, the cell monolayer was dissociated when the confluency was 70–80%. Next, the cell suspension was dispensed 200 µL/well into ultra-low attachment 96-well round bottom plates (Cat#7007, Corning, Lawrenceville, USA). The medium was refreshed every other day.
Mouse model of MM
For in vivo assessment of the effects of PAL on MM, 5×105 myeloma U266 and KMS27 cells were injected subcutaneously into Non-Obese Diabetic (NOD) mice (4–6 weeks of age, male and female, purchased from Cyagen, Suzhou, China) to create the MM mouse models and randomly divided two groups. PAL [35 and 75 mg/kg in 50 mM sodium lactate buffer (pH 4.0)] was administered to U266 and KMS27 mice (six mice per group) orally 5 days per week for 3 weeks. Following treatment, the mice were continuously monitored until death or study cessation, and the mice were sacrificed by spine dislocation. The tumors were then harvested, the sizes of the tumors were measured, and the tumor volume was calculated using the formula V = 0.5 (L × W2), with the width being the shorter tumor diameter.
Real-time quantitative reverse transcription PCR (qRT-PCR)
For qRT-PCR, total Ribonucleic acid (RNA) was isolated using Trizol according to the manufacturer’s instructions. RNA was quantified using NanoDrop® ND-1000 (Thermo Scientific, Waltham, MA, USA) and the RNA content of the samples was normalized. The cDNA was synthesized using the BeyoRT™ cDNA kit following the manufacturer’s instructions. RT-PCR was performed using a BeyoFast™ SYBR Green qPCR Mix (2X) according to the manufacturer’s instructions. Amplification was carried out on a GeneAMP PCR System 9700 thermocycler (Applied Biosystems, Foster City, CA, USA). Thermocycling conditions consisted of a 30 min hold at 50 ℃ for reverse transcription, 15 min at 95 ℃, and 35 cycles of 30 s at 95 ℃, 30 s at 50 ℃ and 45 s at 72 ℃, with a final hold of 7 min at 72 ℃. The primer sequences for MCM2 (Minichromosome Maintenance Complex Component 2), MCM3 (Minichromosome Maintenance Complex Component 3), DHFR, and GAPDH (glyceraldehyde-3-phosphate dehydrogenase) were listed in Table 1.
Table 1
Gene name | Primer | Sequence | Tm |
---|---|---|---|
MCM2 | Sense | GCGAAACCTGGTTGTTGCTG | 60.59 |
Anti-sense | GAGGTGAGGGCATCAGTACG | 59.90 | |
MCM3 | Sense | TGGGGATTCATACGACCCCT | 60.03 |
Anti-sense | GTGAGTCTGCCGTCTTTGGA | 59.97 | |
DHFR | Sense | GTCGCTGTGTCCCAGAACAT | 60.32 |
Anti-sense | TCTGAATTCATTCCTGAGCGG | 58.09 | |
GAPDH | Sense | AATGGGCAGCCGTTAGGAAA | 59.96 |
Anti-sense | GCCCAATACGACCAAATCAGAG | 59.39 |
qRT-PCR, quantitative reverse transcription polymerase chain reaction.
Cell viability assay
The cell viability assay was performed using CCK-8 method and the CCK-8 Cell Proliferation and Cytotoxicity Assay Kit according to the manufacturer’s instructions.
Western blot analysis
Whole proteins were extracted from the U266 and KMS27 monolayers using RIPA buffer (strong) according to the manufacturer’s instructions. The protein concentrations were quantified using BCA assay reagent (Beyotime). MCM2 (D7G11) XP® Rabbit mAb (1:200, Cell Signaling Technology, #3619), MCM3 (D47B6) Rabbit mAb (#4003), DHFR (E6L1H) rabbit monoclonal antibody (mAb) (1:200, Cell Signaling Technology, #43497), and GAPDH monoclonal antibody (1:100, Proteintech, Cat No. 60004-1-Ig).
Cell cycle analysis
The effects of PAL, PTX, or MTX on cell cycle progression were tested by flow cytometry. Briefly, the U266 or KMS27 cells (3.0×105 per well) were seeded and then treated with different concentrations of drugs. Following treatment (24 h), the cells were collected, fixed, resuspended in 500 µL PBS containing 60 µg/mL DNase-free RNase A (Sigma Aldrich, St. Louis, MO, USA) and 50 µg/mL propidium iodide (PI).
The percentage of cells in the G1, S, and G2 phases was analyzed with a flow cytometer (Becton Dickinson, FACS Calibur, San Jose, CA, USA), with 10,000 cells analyzed for each sample. Data obtained from the flow cytometer were analyzed using the FlowJo Software (Tree Star, Inc., Ashland, OR, USA).
Statistical analysis
Statistical analysis was performed using GraphPad Prism 5 software (GraphPad Software, Inc., La Jolla, CA, USA). Between-group differences were analyzed using the two-tailed Student’s t-test or Tukey’s multiple comparison tests. Data were expressed as mean ± standard error of mean (SEM). P<0.05 was considered statistically significant.
Results
PAL significantly induced apoptosis of U266 and KMS27 cells
To investigate the effects of PAL on MM, U266 and KMS27 cells were treated with different concentrations (1, 10, 50, 100, and 200 nM) of PAL. The results showed that 10, 50, 100 and 200 nM PAL could significantly increase expression of apoptosis genes including caspase3 (Figure 1A) and caspase9 (Figure 1B) in U266 cells. Also, PAL significantly suppressed the cell viability of U266 cells (Figure 1C). Similarly, it was also found that PAL remarkably increased the expression level of caspase3 (Figure 1D) and caspase9 (Figure 1E) in KMS27 cells. Moreover, PAL significantly suppressed the cell viability of KMS27 cells (Figure 1F). Thus, it was confirmed that PAL could induce apoptosis in MM cell lines.
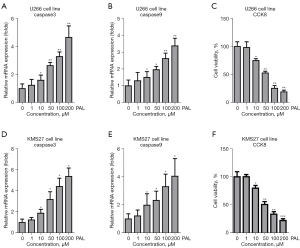
Co-treatment of PAL and PTX or MTX synergistically induced apoptosis of U266 and KMS27 cells
The effects of PTX alone and co-treatment of PAL and PTX on U266 and KMS27 cell lines were investigated to compare the combinatory effects of PAL and other common anticancer drugs (PTX and MTX) on MM cells. It was found that PTX alone could significantly increase the mRNA levels of apoptosis genes, including caspase3 (Figure 2A) and caspase9 (Figure 2B), in U266 cells.
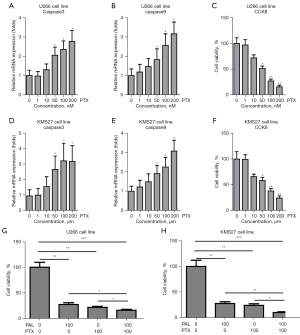
Moreover, PTX significantly suppressed the cell viability of U266 cells (Figure 2C), and remarkably increased the expression levels of caspase3 (Figure 2D) and caspase9 (Figure 2E) in KMS27 cells. PTX also substantially suppressed the cell viability of KMS27 cells (Figure 2F). Notably, we observed that co-treatment of PTX and PAL synergistically induced apoptosis in U266 (Figure 2G) and KMS27 (Figure 2H) cells.
To further probe this, the MM cell lines were treated with MTX. It was found that MTX alone could significantly increase the mRNA level of apoptosis genes, including caspase3 (Figure 3A) and caspase9 (Figure 3B), in U266 cells. Moreover, MTX markedly suppressed the cell viability of U266 cells (Figure 3C). Similarly, MTX remarkably increased the expression level of caspase3 (Figure 3D) and caspase9 (Figure 3E) in KMS27 cells. MTX substantially suppressed the cell viability of KMS27 cells (Figure 3F). Notably, co-treatment of MTX and PAL was found to synergistically induce apoptosis in U266 (Figure 3G) and KMS27 (Figure 3H) cells.
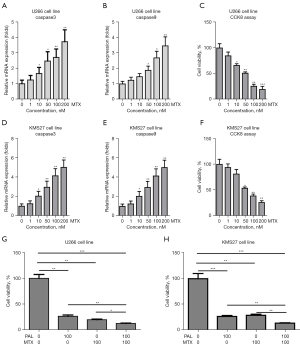
PAL significantly increased the expression level of cell cycle genes including MCM2 and MCM3 in MM cell lines
The cell cycle plays an important role in growth of cancer cells. Thus, it is necessary to explore the effects of PAL on cell cycle-related genes. It was found that PAL could increase the mRNA expression of MCM2 (Figure 4A) and MCM3 (Figure 4B) in a dose-dependent manner in the U266 cell line. To further confirm this, western blot (WB) was used to demonstrate the effects of PAL on cell cycle genes. It was found that PAL could increase the protein levels of MCM2 and MCM3 (Figure 4C) in the U266 cell line. Similarly, it was found that PAL increased the mRNA expressions of MCM2 (Figure 4D) and MCM3 (Figure 4E) in KMS27 cells, and also increased the protein levels of MCM2 and MCM3 (Figure 4F) in these cells.
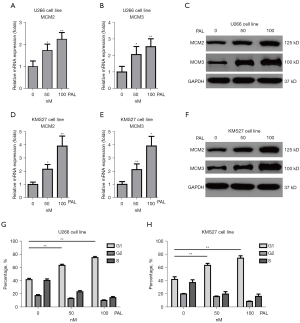
In order to further demonstrate, FACS was used to check effects of PAL on cell cycle, which indicated PAL remarkably increased number of G1-phage cells in both U266 cell line (Figure 4G) and KMS27 cell line (Figure 4H). However, other two anticancer drugs including PTX and MTX showed very minor effects on cell cycle (Figure S1). Collectively, it was confirmed that PAL significantly regulates cell cycle of MM cell lines.
PAL significantly decreased expression of DHFR in MM cell lines
Our previous study confirmed that PAL could decrease the DHFR level in bladder cancer cells (8). Thus, it was checked effects of expression of DHFR in MM cell lines. In this study, we found that PAL could significantly decrease the mRNA expression of DHFR in U266 cells in a dose-dependent manner (Figure 5A). PAL could also reduce protein level of DHFR in U266 cells (Figure 5B). Similarly, we also observed that PAL significantly decreased the mRNA expression (Figure 5C) and protein level (Figure 5D) of DHFR in KMS27 cells in a dose-dependent manner.
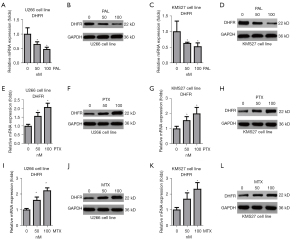
However, PTX exerted different effects on the expression of DHFR; it increased the mRNA expression (Figure 5E) and protein level (Figure 5F) of DHFR in the U266 cell line, and also increased the mRNA expression (Figure 5G) and protein level (Figure 5H) of DHFR in the KMS27 cell line. Similarly, MTX increased the mRNA expression (Figure 5I) and protein level (Figure 5J) of DHFR in the U266 cell line, and also increased the mRNA expression (Figure 5K) and protein level (Figure 5L) of DHFR in the KMS27 cell line. Taken together, these results confirmed that PAL decreases the expression of DHFR, while PTX and MTX increase the expression of DHFR.
PAL inhibits the growth of MM cells by targeting DHFR
To explore whether PAL exerts its effect on the growth of MM cells by targeting DHFR, we performed knockdown of DHFR using small interfering RNA (siRNA). If was found that siRNA against DHFR could significantly knock down the mRNA expression and protein level of DHFR in the U266 cell line (Figure 6A,6B). Interestingly, we observed that siDHFR alone could increase the mRNA expression of MCM2 in the U266 cell line, while PAL could not further increase the expression level of MCM2 in this cell line under DHFR knockdown (Figure 6C). Similarly, it was found that siDHFR alone could decrease the cell viability of the U266 cell line, while PAL could not further decrease the cell viability of U266 cell line under DHFR knockdown (Figure 6D). To further confirm this, DHFR was knocked down at the mRNA (Figure 6E) and protein (Figure 6F) levels in the KMS27 cell line. It was found that siDHFR alone could increase the mRNA expression of MCM2 in the KMS27 cell line, while PAL could not further increase the expression level of MCM2 in the KMS27 cell line under DHFR knockdown (Figure 6G). It was found that siDHFR alone could decrease the cell viability of the KMS27 cell line, while PAL could not further decrease the cell viability of the KMS27 cell line under DHFR knockdown (Figure 6H). Taken together, these results confirmed that PAL regulates the cell cycle and inhibits the growth of MM cells by targeting DHFR.
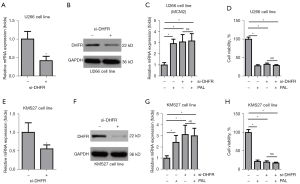
PAL induced apoptosis via DHFR in the 3D cancer spheroids
To further investigate effects of PAL on the apoptosis of MM cancer cells and relative MOA. 3D cancer spheroids, fabricated using the U266 MM cell line (Figure 7A), were knocked down by siDHFR, and it was found that DHFR was successfully knocked down in the U266 spheroid (Figure 7B). Importantly, we observed that PAL could induce increase the apoptosis gene caspase3 and DHFR depletion also increased the expression level of caspase3, while PAL treatment could not further increase the expression level of caspase3 in the U266 spheroids (Figure 7C). Similarly, 3D spheroids were cultured using the KMS27 MM cell line (Figure 7D), and it was found DHFR was successfully knocked down in the KMS27 spheroid (Figure 7E). Furthermore, it was also found that PAL could induce increase the apoptosis gene caspase3 and DHFR depletion also increased the expression level of caspase3, while PAL treatment could not further increase the expression level of caspase3 in the KMS27 spheroids (Figure 7F).
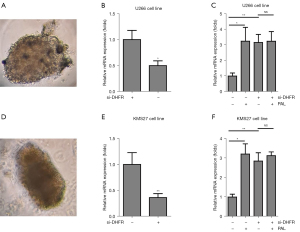
PAL reduced MM tumor in mouse mode
To further explore the effects of PAL on MM, naked mice carrying U266 and KMS27 cells were administered different doses of PAL (35 and 75 mg/kg) and saline. It was found that PAL could significantly decrease the expression of DHFR in U266 mice (Figure 8A) and markedly increased the expression level of the apoptosis marker, caspase3, in U266 mice (Figure 8B). Notably, PAL (35 and 75 mg/kg) remarkably decreased the tumor sizes in U266 mice (Figure 8C,8D). Moreover, it was observed that PAL could substantially decrease the expression of DHFR in the KMS27 mice (Figure 8E). PAL significantly increased the expression level of the apoptosis marker, caspase9, in the KMS27 mice (Figure 8F), and remarkably decreased the tumor sizes in KMS27 mice (Figure 8G,8H). Taken together, these results confirmed that PAL could reduce MM tumors in the mouse model.
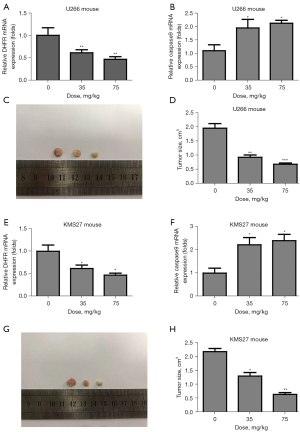
Discussion
MM is the second most commonly diagnosed hematological malignancy, with nearly 160,000 new cases reported worldwide in 2018. Although the outcomes of MM have improved dramatically over the past two decades, our understanding of MM still remains very limited (10). In the present study, it was found that PAL could robustly inhibit the viability of the t (11;14) MM cell line, including U266 and KMS27. Furthermore, PAL could synergistically inhibit the viability of MM cells together with commonly used anticancer drugs, including PTX and MTX. Interestingly, PAL significantly increased G1 phase cells in the U266 and KMS27 cell lines. Finally, it was confirmed that PAL inhibited the viability of MM cells by targeting DHFR in both cell lines and the 3D spheroids models. Our findings could enhance our understanding of MM and aid in the development of novel therapeutic strategies.
The t (11;14) (q13;32) translocation [t (11;14)] is found in about 20% of patients with MM, but studies examining its prognostic ability is different, and data on outcomes from first-line therapy are lacking (11). The resent study from retrospective reviews have shown that t (11;14) is associated with intermediate outcomes in patients treated with novel agents, as compared with patients who have standard- or high-risk cytogenetic aberrations (12). However, the underlying etiology of t (11;14) MM remains unclear (13). At present, there are no effective therapeutic strategies to treat this disease (14). In the present study, we investigated the pathogenesis of t (11;14) MM in depth using cell lines and a 3D spheroids model, and found for the first time that DHFR is involved in the pathogenesis of t (11;14) MM.t(11; 14) leads to the abnormality of cell cycle related gene CCND1. Therefore, we try to explore the treatment of t (11; 14) myeloma from the combination of new agents that can affect the cell cycle.
As a small-molecule inhibitor of CDK4 and CDK6, PAL has been demonstrated to exert significant anticancer effects against estrogen-receptor (ER)-positive breast cancer cells (15). It can also suppress ovarian cancer and has shown synergistic effects against c-my (MYC) overexpressing ovarian cancer cells together with Olaparib (16). As a class of taxeme with microtubule-stabilizing ability, PTX is considered to be the standard care for the management of primary ovarian cancer (17). As a synthetic organic compound, MTX has been broadly used to treat multiple neoplasms including acute lymphoblastic leukemia, acute myeloid leukemia, meningeal leukemia and lymphoma, osteosarcomas, non-Hodgkin’s lymphoma, as well as breast, bladder, and number of other cancers (18). Co-treatment with anticancer drugs has been confirmed as an effective strategy to treat various cancers. In the present study, it was demonstrated that co-treatment of PAL and PTX or MTX could synergistically inhibit the growth of MM cells (Figures 2,3).
The cell cycle plays an important role in cancer pathogenesis. It comprises precise duplication of the genome throughout the DNA synthesis stage (S phase) and separation of whole sets of chromosomes to one of the daughter cells in the mitosis stage (M phase) (19). The G1 phase links the accomplishment of the M phase to the commencement of the S phase in the succeeding cycle (20). In fact, cell cycle proteins such as CDK4 and CDK6 are often dysregulated in malignant cells, and are potential targets for anticancer drug development (21). Previous studies have demonstrated that CDK4/6 is the key regulator of G1-S transition (22). As an FDA approved drug, PAL can effectively inhibit CDK4/6 (23). It exerts anticancer effects on breast cancer by targeting the cell cycle machinery. Huang et al. reported that PAL can induce cell cycle arrest and senescence in human renal tubular epithelial cells (24). In patients with hormone-receptor-positive metastatic breast cancer, Turner et al. found that PAL combined with fulvestrant resulted in longer progression-free survival than fulvestrant alone (25). In the present study, we found that PAL could significantly increase the expression level of cell cycle genes and the G1 phase in MM cells (Figure 4). However, it was demonstrated that anticancer drugs including PTX and MTX did not alter the cell cycle in MM cells (Figure S1). Interestingly, we found that PAL could remarkably reduce the expression level of DHFR, while PTX and MTX increased the expression level of DHFR in MM cells (Figure 5). Importantly, it was found effects of PAL on cell cycle and growth of MM cells was through targeting DHFR (Figure 6).
Accumulating evidence has shown that the 3D model is a promising in vitro model for disease modeling and drug discovery (26-29). New cancer drug approval rates are ≤5%, which is significantly lower than that of other therapeutic areas due to lack of effective in vitro screening models (30). In the present study, two kinds of spheroids were constructed using the U266 and KMS27 MM cell lines. It was observed that PAL and DHFR depletion could induce apoptosis in MM cancer spheroids. Mechanistically, it was found that PAL failed to further induce the expression of apoptosis genes after DHFR depletion (Figure 7). In summary, it was demonstrated that PAL could induce MM cancer cell apoptosis by targeting DHFR in the 3D MM cancer spheroids.
In conclusion, the present study found that PAL exerted significant anticancer effects on MM cells and had synergistic effects together with PTX and MTX. PAL could promote cell cycle genes and the G1 phase of MM cells, and also reduced the expression level of DHFR. PAL exerted its anticancer effects on MM cells by targeting DHFR. The results of the present study might help to understand the pathogenesis of MM and aid in the development of novel therapies.
Acknowledgments
Funding: None.
Footnote
Reporting Checklist: The authors have completed the ARRIVE reporting checklist. Available at https://atm.amegroups.com/article/view/10.21037/atm-22-2830/rc
Data Sharing Statement: Available at https://atm.amegroups.com/article/view/10.21037/atm-22-2830/dss
Conflicts of Interest: All authors have completed the ICMJE uniform disclosure form (available at https://atm.amegroups.com/article/view/10.21037/atm-22-2830/coif). The authors have no conflicts of interest to declare.
Ethical Statement: The authors are accountable for all aspects of the work in ensuring that questions related to the accuracy or integrity of any part of the work are appropriately investigated and resolved. All animal experiments were approved by Shengjing Hospital of China Medical University Institutional Animal Care and Use Committee (No. SHCMU2020010) and complied with institutional guidelines for the care and use of animals. This article does not contain any studies with human participants performed by any of the authors. A protocol was prepared before the study without registration.
Open Access Statement: This is an Open Access article distributed in accordance with the Creative Commons Attribution-NonCommercial-NoDerivs 4.0 International License (CC BY-NC-ND 4.0), which permits the non-commercial replication and distribution of the article with the strict proviso that no changes or edits are made and the original work is properly cited (including links to both the formal publication through the relevant DOI and the license). See: https://creativecommons.org/licenses/by-nc-nd/4.0/.
References
- Touzeau C, Maciag P, Amiot M, et al. Targeting Bcl-2 for the treatment of multiple myeloma. Leukemia 2018;32:1899-907. [Crossref] [PubMed]
- Walker BA, Mavrommatis K, Wardell CP, et al. Identification of novel mutational drivers reveals oncogene dependencies in multiple myeloma. Blood 2018;132:587-97. [Crossref] [PubMed]
- Llanos S, Megias D, Blanco-Aparicio C, et al. Lysosomal trapping of palbociclib and its functional implications. Oncogene 2019;38:3886-902. [Crossref] [PubMed]
- Miettinen TP, Peltier J, Härtlova A, et al. Thermal proteome profiling of breast cancer cells reveals proteasomal activation by CDK4/6 inhibitor palbociclib. EMBO J 2018;37:e98359. [Crossref] [PubMed]
- Diéras V, Harbeck N, Joy AA, et al. Palbociclib with Letrozole in Postmenopausal Women with ER+/HER2- Advanced Breast Cancer: Hematologic Safety Analysis of the Randomized PALOMA-2 Trial. Oncologist 2019;24:1514-25. [Crossref] [PubMed]
- Kalogris C, Garulli C, Pietrella L, et al. Sanguinarine suppresses basal-like breast cancer growth through dihydrofolate reductase inhibition. Biochem Pharmacol 2014;90:226-34. [Crossref] [PubMed]
- Abali EE, Skacel NE, Celikkaya H, et al. Regulation of human dihydrofolate reductase activity and expression. Vitam Horm 2008;79:267-92. [Crossref] [PubMed]
- Wang X, Wang H, Song Y. Clinical efficacy and mechanism of Pralatrexate combined with Palbociclib Isethionate in treatment of bladder cancer patients. Oncol Lett 2019;17:201-8. [PubMed]
- Rolver MG, Elingaard-Larsen LO, Pedersen SF. Assessing Cell Viability and Death in 3D Spheroid Cultures of Cancer Cells. J Vis Exp 2019; [Crossref] [PubMed]
- Brigle K, Rogers B. Pathobiology and Diagnosis of Multiple Myeloma. Semin Oncol Nurs 2017;33:225-36. [Crossref] [PubMed]
- Gasparetto C, Jagannath S, Rifkin RM, et al. Effect of t (11;14) Abnormality on Outcomes of Patients With Newly Diagnosed Multiple Myeloma in the Connect MM Registry. Clin Lymphoma Myeloma Leuk 2022;22:149-57. [Crossref] [PubMed]
- Szita VR, Mikala G, Kozma A, et al. Targeted Venetoclax Therapy in t(11;14) Multiple Myeloma: Real World Data From Seven Hungarian Centers. Pathol Oncol Res 2022;28:1610276. [Crossref] [PubMed]
- Pan D, Kaufman JL, Htut M, et al. Filanesib plus bortezomib and dexamethasone in relapsed/refractory t(11;14) and 1q21 gain multiple myeloma. Cancer Med 2022;11:358-70. [Crossref] [PubMed]
- Bi JY, Wen L, Duan WB, et al. Efficacy and safety analysis of BCL-2 inhibitor in relapsed/refractory multiple myeloma with t (11;14) in a single center. Zhonghua Xue Ye Xue Za Zhi 2022;43:146-9. [PubMed]
- Finn RS, Martin M, Rugo HS, et al. Palbociclib and Letrozole in Advanced Breast Cancer. N Engl J Med 2016;375:1925-36. [Crossref] [PubMed]
- Yi J, Liu C, Tao Z, et al. MYC status as a determinant of synergistic response to Olaparib and Palbociclib in ovarian cancer. EBioMedicine 2019;43:225-37. [Crossref] [PubMed]
- Meng Z, Lv Q, Lu J, et al. Prodrug Strategies for Paclitaxel. Int J Mol Sci 2016;17:796. [Crossref] [PubMed]
- Ramsey LB, Balis FM, O'Brien MM, et al. Consensus Guideline for Use of Glucarpidase in Patients with High-Dose Methotrexate Induced Acute Kidney Injury and Delayed Methotrexate Clearance. Oncologist 2018;23:52-61. [Crossref] [PubMed]
- Kastan MB, Bartek J. Cell-cycle checkpoints and cancer. Nature 2004;432:316-23. [Crossref] [PubMed]
- Kar S. Unraveling Cell-Cycle Dynamics in Cancer. Cell Syst 2016;2:8-10. [Crossref] [PubMed]
- Petroni G, Formenti SC, Chen-Kiang S, et al. Immunomodulation by anticancer cell cycle inhibitors. Nat Rev Immunol 2020;20:669-79. [Crossref] [PubMed]
- O'Leary B, Finn RS, Turner NC. Treating cancer with selective CDK4/6 inhibitors. Nat Rev Clin Oncol 2016;13:417-30. [Crossref] [PubMed]
- Schmidt M, Sebastian M. Palbociclib-The First of a New Class of Cell Cycle Inhibitors. Recent Results Cancer Res 2018;211:153-75. [Crossref] [PubMed]
- Huang L, Shen Y, Liu C, et al. Palbociclib induces cell cycle arrest and senescence of human renal tubular epithelial cells in vitro. Nan Fang Yi Ke Da Xue Xue Bao 2020;40:1784-92. [PubMed]
- Turner NC, Ro J, André F, et al. Palbociclib in Hormone-Receptor-Positive Advanced Breast Cancer. N Engl J Med 2015;373:209-19. [Crossref] [PubMed]
- Drost J, Clevers H. Organoids in cancer research. Nat Rev Cancer 2018;18:407-18. [Crossref] [PubMed]
- Yin Y, Liu PY, Shi Y, et al. Single-Cell Sequencing and Organoids: A Powerful Combination for Modelling Organ Development and Diseases. Rev Physiol Biochem Pharmacol 2021;179:189-210. [Crossref] [PubMed]
- Han K, Pierce SE, Li A, et al. CRISPR screens in cancer spheroids identify 3D growth-specific vulnerabilities. Nature 2020;580:136-41. [Crossref] [PubMed]
- Yin YB, de Jonge HR, Wu X, et al. Mini-gut: a promising model for drug development. Drug Discov Today 2019;24:1784-94. [Crossref] [PubMed]
- Amirghasemi F, Adjei-Sowah E, Pockaj BA, et al. Microengineered 3D Tumor Models for Anti-Cancer Drug Discovery in Female-Related Cancers. Ann Biomed Eng 2021;49:1943-72. [Crossref] [PubMed]
(English Language Editor: A. Kassem)