GRSF1 predicts an unfavorable prognosis and promotes tumorigenesis in lung adenocarcinoma based on bioinformatics analysis and in vitro validation
Introduction
According to GLOBOCAN 2020 estimates, lung cancer is the leading cause of cancer-related death worldwide (and accounts for 18.0% of the estimated 1.8 million deaths), and accounts for twice as many deaths as colorectal cancer, which is the second most deadly cancer worldwide (1). Lung adenocarcinoma (LUAD) is the predominant pathological subtype of malignant lung cancers, for which radical resection, radiotherapy, and chemotherapy are available therapeutic options (2,3). However, the survival rates of lung cancer patients remain poor due to metastatic disease, and the 5-year relative survival ratio is only 5% in metastasized cancer, while that of localized stage disease is 57% (4). With high levels of heterogeneity, the initiating elements that determine the biological behaviors of carcinoma cells include multiple altered genes, cell signals caused by aberrant gene expression profiles, and the immune microenvironment (5-7). Until now, numerous genes have been reported to be associated with the tumorigenesis of LUAD, but only few biomarkers have been developed for clinical practice. Thus, identifying cancer-associated-genes with potential clinical implications and clarifying the biological mechanisms associated with tumor malignant transformation may be profitable for LUAD diagnosis and therapy.
There is accumulating evidence that ribonucleic acid (RNA)–binding proteins (RBPs) account for the complexity in the occurrence and malignancy of cancers (8-10). Guanine-rich RNA sequence binding factor 1 (GRSF1) is a cytosolic RBP that is initially synthetized by nuclear deoxyribonucleic acid (DNA), and then transfers to the mitochondria due to its mitochondria-localization signal (11,12). A series of studies have demonstrated that GRSF1 functioned in DNA damage regulated by oxidative stress (13), cell senescence (14), inflammatory program (15), and the stabilization of target messenger RNAs (mRNAs) (12). A recent study demonstrated that GRSF1 can serve as a prognostic biomarker in patients with sepsis (16). GRSF1 is characterized by 3 RNA recognition motif (RRM) domains (which are responsible for RNA-binding), and participates in cancer progression via the mediation of microRNA-mediated gene expression (17,18). Previous research has shown that patients suffering from estrogen receptor–positive breast cancer with high levels of GRSF1 are more resistant to tamoxifen than those patients with low levels of GRSF1 (19). Notably, GRSF1 has been observed to facilitate the malignant behavior of cervical cancer cells by stimulating cancer-related signal pathways (20,21). Additionally, GRSF1 has been reported to promote tumorigenesis and metastasis through the phosphatidylinositide 3-kinases/protein kinase B (PI3K/AKT) signal pathways in gastric cancer (22). GRSF1 has also been shown to drive the progression of hepatocellular carcinoma by binding to yin-yang 1 (YY1) (23). However, much is unknown about the contributions of GRSF1 to lung cancer.
In the current study, we investigated the expression profile of GRSF1 at both the RNA and protein levels, its clinical significance, its latent biological phenotypes, and the unconscious mechanisms of its dysregulation in lung cancer by mining large collections from The Cancer Genome Atlas (TCGA) data sets. Experiments were conducted to verify the biological behavioral changes induced by GRSF1 knockdown in vitro. Our data offered insight into the clinical significance and biological function of GRSF1 in LUAD, providing critical clues for developing prospective diagnostic and prognostic biomarkers in LUAD. We present the following article in accordance with the MDAR and REMARK reporting checklists (available at https://atm.amegroups.com/article/view/10.21037/atm-22-2798/rc).
Methods
In the current study, we evaluated the expression of GRSF1 in LUAD and normal samples by multiple online service portals. The relationships between GRSF1 expression and clinicopathological features in LUAD based on TCGA data sets were analyzed by R packages and UALCAN website. Besides, prognostic significance of GRSF1 in LUAD was identified by the Gene Expression Profiling Interactive Analysis 2 (GEPIA2) and Kaplan-Meier (K-M) Plotter. We next profiled the genetic mutations of GRSF1 using cBioPortal, compared GRSF1 expression in different genetic statuses, and analyzed the correlation between GRSF1 expression and methylation degree using R packages. The co-expressed genes of GRSF1 were obtained from LinkedOmics, and functional enrichments were then constructed by R clusterProfiler. Furthermore, cell counting kit 8 (CCK-8) and colony formation assays were applied to verify the proliferation effects of GRSF1 on LUAD cells. This study was conducted in accordance with the Declaration of Helsinki (as revised in 2013).
Comprehensive online mining
Tumor Immune Estimation Resource (TIMER; https://cistrome.shinyapps.io/timer/) is a bioinformatic portal tool used to perform extensive examinations of gene expression abundance (24). The “Diff exp” module was used to depict the gene expression profiles in tumors and non-tumors of multiple common types of cancer.
UALCAN (http://ualcan.path.uab.edu/index.html) is an integrated online service portal that contains gene expression profiles and clinicopathologic information from TCGA and the Clinical Proteomic Tumor Analysis Consortium (CPTAC) cohort databases (25). We used the TCGA module to assess the comparative correlation between the mRNA level of GRSF1 and clinicopathological features. Additionally, we compared the protein levels of GRSF1 in primary LUAD samples and control normal samples via the CPTAC module. A P value <0.05 was considered statistically significant.
The Human Protein Atlas (HPA; https://www.proteinatlas.org) is a visual bioinformatics service platform that provides immunohistochemical (IHC) images of around 20 typical normal and tumor tissues (26). IHC photographs of clinical-histological lung tumor specimens together with para-tumor samples were acquired from this website to examine the GRSF1 protein expression patterns in the 2 paired groups.
K-M Plotter (https://kmplot.com/analysis/) is an integrated service portal for determining cancer patients’ survival (27,28), which was engaged to evaluate the prognostic features of GRSF1 in LUAD by assessing the relevance between mRNA levels of GRSF1 and survival, including overall survival (OS) and recurrence-free survival (RFS). The optimal threshold was defined by the K-M Plotter algorithm, and a P value <0.05 was considered statistically significant.
GEPIA2 (http://gepia.cancer-pku.cn/), an enhanced bioinformatics portal for large-scale gene expression profiling and interactive analysis (29), was used to assess the prognostic potential of GRSF1 in LUAD, such as OS and disease-free survival (DFS). The “median” mRNA expression was used to stratify the patients into high and low GRSF1 groups. A P value <0.05 was considered statistically significant. The expression of GRSF1 in LUAD and para-tumor tissues was also visualized by the “Expression DIY” module with the option of log2 (TPM + 1) for log-scale and matching TCGA normal and the GTEx data.
cBioPortal (https://www.cbioportal.org/) is a free access platform for comprehensive searches of all-round tumor genomic data. Alterations of GRSF1 in LUAD were summarized by the “Cancer Types Summary” module (30).
The Cancer Cell Line Encyclopedia (CCLE; https://sites.broadinstitute.org/ccle/) is a simple and objective website that contains genomic information from over 1,000 tumor cell lines (31). The RNA-sequencing data of GRSF1 in different lung cancer cells were downloaded and visualized by heatmaps.
DNA alteration information of GRSF1
The DNA copy number alterations (CNAs) and methylations of GRSF1 from TCGA-LUAD were analyzed in R. GRSF1 CNA and mRNA expression information were downloaded, and the mRNA levels of GRSF1 in the indicated genetic status were assessed and visualized by box plots. Additionally, the correlations between the GRSF1 transcriptional levels and DNA methylation degrees were calculated by linear regression analyses. A P value <0.05 was considered statistically significant.
Associations between GRSF1 expression levels and the clinicopathologic parameters of LUAD
R package “cgdsr” was used to retrieve mRNA expression information and clinical data from TCGA-LUAD. There were 479 patients with complete information on age, gender, pathologic tumor (T), nodes (N) and stage. First, we constructed high and low GRSF1 groups using optimum cut-off points determined by X-Tile software. Next, the correlations between GRSF1 expression levels and the clinicopathological characteristics of LUAD from TCGA portal were specially analyzed using the chi-squared test or Fisher’s exact test in R. A P value <0.05 was considered statistically significant.
Signal pathway enrichment analysis of the genes co-expressed with GRSF1
The top 50 positively and negatively correlated GRSF1 genes were visualized as heatmaps by LinkedOmics (32) from TCGA data, and the top 1,000 positively and negatively correlated genes were tested with the Gene Ontology (GO) (33) and Kyoto Encyclopedia of Genes and Genomes (KEGG) pathway enrichment (34) tools by R clusterProfiler (35) to reveal the mechanism of GRSF1 in LUAD.
Cell cultures and GRSF1 gene silencing
The human lung cancer cell lines of A549 and NCI-H23 were procured from the National Infrastructure of Cell Line Resource (Beijing, China) and grown in 10% fetal bovine serum (Ausbian, Australia)-supplemented Roswell Park Memorial Institute (RPMI)-1640 medium (Gibco, Waltham, MA, USA) with 1× penicillin/streptomycin. The cells were incubated at 37 °C in a humid 5% carbon dioxide incubator. After thawing, the cells were used for 15 passages. The small-interfering RNA (siRNA) sequences targeting GRSF1 used in this system are set out in the supplementary file (see Table S1) and were synthesized at RiboBio Co., Ltd., China. The wild-type A549 and NCI-H23 cells were transfected with pooled siRNAs (siGRSF1 1#: 2#: 3# = 1: 1: 1) using an RNAiMAX kit (Invitrogen, Carlsbad, CA, USA) in accordance with the manual provided. The transfected cells were obtained and subjected to viability and colony formation assays after 24 hours. The cells that were transfected for 48 hours were collected to detect both the mRNA and protein expression of GRSF1 by real-time quantitative polymerase chain reaction (RT-qPCR) and western blot.
RNA extraction and RT-qPCR
The total RNA was extracted from the cells using Trizol (Invitrogen, MA, USA), after which a complementary DNA (cDNA) synthesis was performed using 2 µg of RNA, and the RevertAid First Strand cDNA Synthesis Kit (Thermo Fisher Scientific, MA, USA). For each target sequence, RT-qPCR was conducted in triplicate using the Power Up SYBR Green Master Mix (Applied Biosystems, CA, USA) on a CFX Connect Real-Time System (Bio-Rad, CA, USA). The expression levels of the target genes were determined using the 2-ΔΔCT approach, and the internal control was human β-actin. The primer sequences used in the assay are set out in the supplementary file (see Table S1).
Western blot
The indicated cells were completely lysed and immediately quantified using a bicinchoninic acid assay kit (Pierce Biotechnology, IL, USA). Proteins with 40 µg per lane were subsequently resolved on 10% sodium dodecyl-sulfate polyacrylamide gel electrophoresis and shifted onto polyvinylidene fluoride membranes (Millipore, Boston, USA). The membranes were subsequently blocked with 5% skimmed milk diluted with Tris-buffered saline Tween at 37 °C for 2 hours, and then incubated with primary antibodies, including anti-glyceraldehyde 3-phosphate dehydrogenase (1:3,000, Proteintech, Chicago, USA) and anti-GRSF1 (1:1,000, Abclonal, Wuhan, China) overnight at 4 °C. Thereafter, secondary antibodies, such as anti-rabbit or anti-mouse immunoglobulin G (1:10,000, ZSGB-BIO, Beijing, China), were reacted with the membranes for 1 hour at 37 °C. The protein bands were visualized with an enhanced chemiluminescent detection kit (Millipore, Boston, USA).
Cell proliferation assays
To perform the CCK-8 assays, 1,500 transfected cells were seeded into 1 well of the 96-well plates (Corning-Costar, NY, USA) and cultured as usual. At 24, 48, 72, and 96 hours, the supernatants were removed, and 100 µL of CCK-8 diluent (CCK-8: RPMI medium =1:9) (Dojindo Laboratories, Kumamoto, Japan) was added to each well of the 96-well plate. The cells were then kept at 37 °C for 1 hour, and the 450 nm absorbance value was then collected via a microplate reader. To conduct the colony formation experiments, 500 transfected cells were evenly seeded into 1 well of the 12-well plates and then cultured for another 2 weeks. Next, the colonies were immobilized with methanol and immediately incubated with crystal violet. Each experiment was performed in triplicate.
Statistical analyses
The in-vitro experimental data are shown as the mean and standard deviation. The statistical analyses were all summarized through Prism 7.0. An unpaired t-test was used to compare the differences between the 2 groups. A P value <0.05 (2-tailed) was considered statistically significant.
Results
The expression of GRSF1 was significantly increased in LUAD samples compared to normal lung samples
Using the TIMER data sets, we analyzed the GRSF1 expression profiles in different human cancers and normal organs in TCGA. The tumor tissues displayed a higher expression of GRSF1 than the normal tissues in most cancer types, including LUAD. Compared to other cancers and normal organs, the liver hepatocellular carcinoma (LIHC) samples and its normal samples showed particularly low GRSF1 mRNA levels. While the GRSF1 mRNA level was lower in the kidney renal clear cell carcinoma (KIRC) samples than the normal samples (see Figure 1A). We also compared the GRSF1 expression levels using GEPIA2, and GRSF1 was also observed to be overexpressed in lung tumors (see Figure 1B). As shown by the UALCAN website, GRSF1 mRNA was significantly overexpressed in primary tumors (n=515) compared to normal lung controls (n=59) (see Figure 1C). Further, primary lung tumor groups showed higher protein expression levels of GRSF1 than normal lung groups in the CPTAC database (see Figure 1D). This was validated by the HPA database. The IHC staining of GRSF1 indicated that lung tumor tissues had medium levels of GRSF1 expression while normal lung tissues had low levels of GRSF1 (see Figure 1E). Taken together, the above data indicated that GRSF1 expression increased at both the mRNA and protein levels in the LUAD tissues.
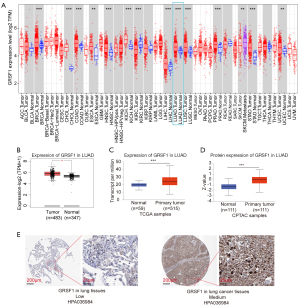
Overexpression of GRSF1 was correlated with clinicopathological features and indicated unfavorable survival in LUAD
We analyzed the relationship between GRSF1 and clinicopathological features based on the best performing threshold in LUAD via TCGA database. GRSF1 transcriptional level was found to have a significant correlation with gender (P=0.009) and T classification (P=0.003; see Table 1). Additionally, GRSF1 expression was more increased in tumor protein 53 (TP53)-mutant patients than TP53 non-mutant patients (see Figure 2A), smokers than non-smokers (see Figure 2B), and males than females (see Figure 2C). Further investigations showed that patients with a higher level of GRSF1 tended to have a more advanced histologic grade based on the CPTAC data sets (see Figure 2D). Next, the prognostic significance of GRSF1 mRNA in patients was identified by both the GEPIA2 website and K-M Plotter, which showed that higher GRSF1 expression indicated worse OS [hazards ratio (HR) =1.6] and DFS (HR =1.4) in LUAD (see Figure 3A,3B). Further, higher GRSF1 expression was associated with worse OS even in male patients (HR =1.79), stage 3 patients (HR =1.94), and mutation burden-high patients (HR =1.91) (see Figure 3C-3E). All of these above results revealed a significant relationship between GRSF1 level and LUAD patients’ progression and prognosis.
Table 1
Characteristics | LUAD (n=479) | χ2 | P value | |
---|---|---|---|---|
GRSF1 low (n=341) | GRSF1 high (n=138) | |||
Age (years) | 0.196 | 0.658 | ||
≥60 | 246 | 103 | ||
<60 | 95 | 35 | ||
Gender | 6.873 | 0.009* | ||
Male | 146 | 78 | ||
Female | 195 | 60 | ||
Nodes_pathologic_pN | 0.596 | |||
N0 | 229 | 87 | ||
N1 | 64 | 27 | ||
N2 | 46 | 24 | ||
N3 | 2 | 0 | ||
Tumor_pathologic_pT | 13.62 | 0.003* | ||
T1 | 131 | 33 | ||
T2 | 175 | 78 | ||
T3 | 26 | 18 | ||
T3 | 9 | 9 | ||
Pathologic_tumor_stage | 3.775 | 0.287 | ||
I | 193 | 65 | ||
II | 81 | 38 | ||
III | 53 | 27 | ||
IV | 14 | 8 |
*, statistically significant P values, P<0.05. GRSF1, guanine-rich RNA sequence binding factor 1; LUAD, lung adenocarcinoma.
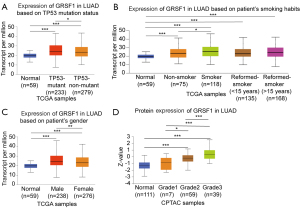
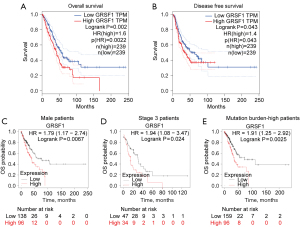
Copy number gain/amplification and DNA hypomethylation favor GRSF1 upregulation in patients suffering from LUAD
To investigate the potential mechanism induced by GRSF1 dysregulation in LUAD, we identified its genetic and epigenetic mutations in TCGA-LUAD. As the cBioPortal showed, the alteration frequency of GRSF1 in patients with LUAD was approximately 4% (see Figure 4A). Based on the transcriptome data and classic CNA data (n=512) in the tumor samples, 1 patient had GRSF1 homozygously deleted (Homdel), 139 had GRSF1 heterozygously deleted (Hetloss), 301 had GRSF1 diploid, 61 had GRSF1 gain, and 10 had GRSF1 amplification (Amp). GRSF1 copy number gain and amplification were significantly associated with increased GRSF1 transcriptional level, while copy number Hetloss was correlated with a decreased GRSF1 level (P<0.05) (see Figure 4B). Further, linear regression analysis of the correlation between GRSF1 transcriptional level and methylation suggested that the total methylation degree of GRSF1 was negatively correlated with its transcriptional level (Pearson’s r=–0.14, P<0.01; see Figure 4C). Together, these results indicated that genetic and epigenetic alterations of GRSF1 were related to its mRNA regulation in LUAD.
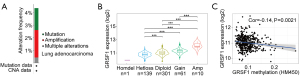
Signal pathway enrichment analysis based on GRSF1–co-expressed genes in LUAD
To determine which genes were co-expressed with GRSF1, the online tool (LinkedOmics) was applied to analyze the transcriptome data of LUAD from TCGA data set. The top 50 significantly positively and negatively correlated genes of GRSF1 were visualized in heatmaps (see Figure 5A,5B). To further examine the underlying biological process (BP) of GRSF1 regulation in LUAD, a total of 2,000 genes (the top 1,000 genes positively related to GRSF1 and the top 1,000 genes negatively related to GRSF1) were used for the GO and KEGG analyses. The top 10 significant terms of molecular function (MF), cellular component (CC), and BP are presented. Notably, in terms of MF, GRSF1 was focused on guanosine triphosphatases (GTPase) regulator activity, ubiquitin-like protein ligase binding, ribonucleoprotein complex binding, and adenosine triphosphate (ATP) hydrolysis activity. The significantly enriched CCs included the mitochondrial matrix, mitochondrial inner membrane, mitochondrial protein-containing complex, and proteasome complex. GRSF1 affected the following BPs: ribonucleoprotein complex biogenesis, protein folding, chromosome segregation, and rRNA metabolic process. Among the KEGG terms, human T-cell leukemia virus 1 infection, protein processing in endoplasmic reticulum, nucleocytoplasmic transport, and the cell cycle were obviously enriched (see Figure 5C-5F).
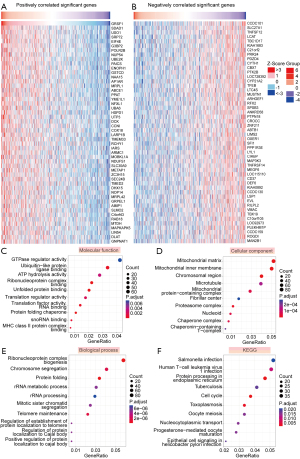
Relationship between GRSF1 expression and pathway status
We compared GRSF1 protein expression in different tumor-associated pathway statuses according to the UALCAN data set. The present data indicated that GRSF1 expression was higher in terms of the Wnt pathway status (see Figure 6A), Hippo pathway status (see Figure 6B), mammalian target of rapamycin (mTOR) pathway status (see Figure 6C), p53/Rb-related pathway status (see Figure 6D), chromatin-modifier status (see Figure 6E), receptor tyrosine kinase (RTK) pathway status (see Figure 6F), switch-sucrose nonfermentable (SWI-SNF) complex status (see Figure 6G), and c-Myc (MYC)/N-Myc (MYCN) status (see Figure 6H). These findings suggested that GRSF1 probably has a constructive effect on regulating lung cancer occurrence and malignant progression due in part to the tumor-associated signaling pathways.
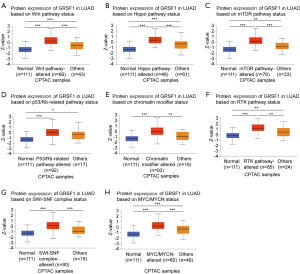
GRSF1 knockdown inhibits lung cancer cell proliferation in vitro
We further verified the critical role of GRSF1 in lung cancer cell lines in vitro. Based on GRSF1 mRNA expression in different cell lines in the CCLE website, A549 and NCI-H23 were selected for the subsequent functional analysis (see Figure S1). Notably, RT-qPCR and western blot detection demonstrated that the interference efficiency of GRSF1 knockdown by siRNA reached approximately 80% (see Figure 7A,7B). Further, the cell viability and colony formation assays showed that GRSF1 knockdown significantly restrained the growth of A549 and NCI-H23 cells (see Figure 7C,7D). In summary, GRSF1 was shown to be an oncogene, and decreased GRSF1 expression weakened the proliferative ability of lung cancer cell lines in vitro.
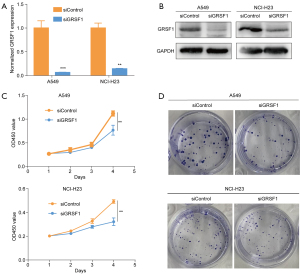
Discussion
Lung cancer still seriously harms human health due to its unusually high morbidity and mortality (1). Due to rapid progresses in genetic research and molecular biology, the identification of novel biomarkers for certain cancers has recently led to the personalized treatment of tumors. Previous reports have shown that GRSF1 acts as an oncogenic gene to promote tumor growth in cervical cancer, gastric cancer, and hepatocarcinoma (20,21,23). However, no previous study had investigated the tumorigenesis role of GRSF1 in LUAD. The current research initially revealed that GRSF1 was significantly more increased in LUAD tissues than control lung tissues at both the mRNA and protein levels. Additionally, the expression of GRSF1 was found to be significantly correlated with TP53-mutant status, smoking habits, gender, and T classification. Patients who expressed a high level of GRSF1 also tended to have a more advanced histologic grade. A further analysis found that a high level of GRSF1 expression predicted unfavorable survival. Thus, GRSF1 could be a prospective diagnostic and prognostic indicator of LUAD.
The possible mechanism underlying GRSF1 aberrant expression in LUAD has not yet been fully elucidated. Located on chromosome 4q13.2, the genetic alteration frequency of GRSF1 occurs in approximately 4% of LUAD patients (30). We found that GRSF1 DNA copy number gain and amplification were significantly associated with increased GRSF1 at the transcriptional level while copy number Hetloss patients had decreased GRSF1 levels. Notably, DNA methylation has commonly been observed in various malignant cancers, and hypomethylation is usually involved in chromosomal instability and gene upregulation (36). We further examined the relationship between the degree of GRSF1 DNA methylation and its transcriptional expression. The data suggested that hypomethylation is positively correlated with GRSF1 upregulation.
To comprehensively elucidate the pronounced function of GRSF1 in LUAD, we also assessed the genes that were significantly positively and negatively correlated to GRSF1 expression and performed signal pathway enrichment analyses in LUAD. The key categories of GO and KEGG were enriched in mitochondrial matrix, ATP activity, ribonucleoprotein complex biogenesis, rRNA processing, and the cell cycle. Due to its RNA-binding properties, GRSF1 plays critical roles in mitochondrial respiration, reactive oxygen species, DNA damage, senescence, and cell growth (13). Further investigations indicated that GRSF1 expression was positively correlated with tumorigenesis pathways, including the Wnt, Hippo, mTOR, RTK, MYC/MYCN, and p53/Rb-related pathways in LUAD. GRSF1 may play a pro-proliferative role in LUAD by driving these signaling pathways.
In vitro experiments were conducted to confirm the predictive effects of GRSF1 on LUAD. We silenced GRSF1 by transfecting siRNAs in 2 lung cancer cell lines (i.e., A549 and NCI-H23) and confirmed that the knockdown of GRSF1 impaired cell proliferation, which in turn validated the oncogenic role of GRSF1 in LUAD. Until now, very little research has investigated the detailed function of GRSF1 in LUAD. Thus, it would be of great value to further explain the accurate molecular regulatory network by which GRSF1 dominates cell abnormal proliferation and to develop targeted inhibitors to suppress tumor cell growth induced by GRSF1 in the future.
In summary, the current research provides substantial evidence of the upregulation of GRSF1 and supports the possibility of GRSF1 being used as an indicator for disease progression and survival in LUAD. DNA gain/amplification and hypomethylation could be mechanisms associated with GRSF1 dysregulation in LUAD. Interference with GRSF1 can significantly suppress the growth of lung cancer cells in vitro. Based on these findings, GRSF1 may be a valuable target for the further development of anti-cancer strategies in LUAD.
Conclusions
The upregulation of GRSF1 predicted poor disease progression and survival in LUAD. Our current study investigated the role of GRSF1 in LUAD and proposed a valuable prognostic biomarker and therapeutic target for LUAD.
Acknowledgments
Funding: This research was funded by Shenzhen High-Level Hospital Construction Fund and Sanming Project of Medicine in Shenzhen (No. SZSM202011010).
Footnote
Reporting Checklist: The authors have completed the MDAR and REMARK reporting checklists. Available at https://atm.amegroups.com/article/view/10.21037/atm-22-2798/rc
Data Sharing Statement: Available at https://atm.amegroups.com/article/view/10.21037/atm-22-2798/dss
Conflicts of Interest: All authors have completed the ICMJE uniform disclosure form (available at https://atm.amegroups.com/article/view/10.21037/atm-22-2798/coif). HZ serves as unpaid Associate Editor-in-Chief of Annals of Translational Medicine from June 2019 to May 2024. The other authors have no conflicts of interest to declare.
Ethical Statement: The authors are accountable for all aspects of the work, including ensuring that any questions related to the accuracy or integrity of any part of the work have been appropriately investigated and resolved. The study was conducted in accordance with the Declaration of Helsinki (as revised in 2013).
Open Access Statement: This is an Open Access article distributed in accordance with the Creative Commons Attribution-NonCommercial-NoDerivs 4.0 International License (CC BY-NC-ND 4.0), which permits the non-commercial replication and distribution of the article with the strict proviso that no changes or edits are made and the original work is properly cited (including links to both the formal publication through the relevant DOI and the license). See: https://creativecommons.org/licenses/by-nc-nd/4.0/.
References
- Sung H, Ferlay J, Siegel RL, et al. Global Cancer Statistics 2020: GLOBOCAN Estimates of Incidence and Mortality Worldwide for 36 Cancers in 185 Countries. CA Cancer J Clin 2021;71:209-49. [Crossref] [PubMed]
- Testa U, Castelli G, Pelosi E. Lung Cancers: Molecular Characterization, Clonal Heterogeneity and Evolution, and Cancer Stem Cells. Cancers (Basel) 2018;10:248. [Crossref] [PubMed]
- Hanaoka J, Yoden M, Hayashi K, et al. Dynamic perfusion digital radiography for predicting pulmonary function after lung cancer resection. World J Surg Oncol 2021;19:43. [Crossref] [PubMed]
- Siegel RL, Miller KD, Jemal A. Cancer statistics, 2020. CA Cancer J Clin 2020;70:7-30. [Crossref] [PubMed]
- de Sousa VML, Carvalho L. Heterogeneity in Lung Cancer. Pathobiology 2018;85:96-107. [Crossref] [PubMed]
- Dai X, Lu L, Deng S, et al. USP7 targeting modulates anti-tumor immune response by reprogramming Tumor-associated Macrophages in Lung Cancer. Theranostics 2020;10:9332-47. [Crossref] [PubMed]
- Fan Y, Liu B, Chen F, et al. Hepcidin Upregulation in Lung Cancer: A Potential Therapeutic Target Associated With Immune Infiltration. Front Immunol 2021;12:612144. [Crossref] [PubMed]
- Wang ZL, Li B, Luo YX, et al. Comprehensive Genomic Characterization of RNA-Binding Proteins across Human Cancers. Cell Rep 2018;22:286-98. [Crossref] [PubMed]
- Masuda K, Kuwano Y. Diverse roles of RNA-binding proteins in cancer traits and their implications in gastrointestinal cancers. Wiley Interdiscip Rev RNA 2019;10:e1520. [Crossref] [PubMed]
- Pereira B, Billaud M, Almeida R. RNA-Binding Proteins in Cancer: Old Players and New Actors. Trends Cancer 2017;3:506-28. [Crossref] [PubMed]
- Jourdain AA, Koppen M, Wydro M, et al. GRSF1 regulates RNA processing in mitochondrial RNA granules. Cell Metab 2013;17:399-410. [Crossref] [PubMed]
- Antonicka H, Sasarman F, Nishimura T, et al. The mitochondrial RNA-binding protein GRSF1 localizes to RNA granules and is required for posttranscriptional mitochondrial gene expression. Cell Metab 2013;17:386-98. [Crossref] [PubMed]
- Noh JH, Kim KM, Pandey PR, et al. Loss of RNA-binding protein GRSF1 activates mTOR to elicit a proinflammatory transcriptional program. Nucleic Acids Res 2019;47:2472-86. [Crossref] [PubMed]
- Kim SJ, Chun M, Wan J, et al. GRSF1 is an age-related regulator of senescence. Sci Rep 2019;9:5546. [Crossref] [PubMed]
- Zou YH, Zhao L, Xu YK, et al. Anti-inflammatory sesquiterpenoids from the Traditional Chinese Medicine Salvia plebeia: Regulates pro-inflammatory mediators through inhibition of NF-κB and Erk1/2 signaling pathways in LPS-induced Raw264.7 cells. J Ethnopharmacol 2018;210:95-106. [Crossref] [PubMed]
- Qi L, Wu Y, Li M, et al. G-rich sequence factor 1 serves as a prognostic biomarker in septic patients. Ann Transl Med 2021;9:691. [Crossref] [PubMed]
- Song G, Wang R, Guo J, et al. miR-346 and miR-138 competitively regulate hTERT in GRSF1- and AGO2-dependent manners, respectively. Sci Rep 2015;5:15793. [Crossref] [PubMed]
- Noh JH, Kim KM, Abdelmohsen K, et al. HuR and GRSF1 modulate the nuclear export and mitochondrial localization of the lncRNA RMRP. Genes Dev 2016;30:1224-39. [Crossref] [PubMed]
- Wang Y, Gong X, Zhang Y. Network-based approach to identify prognosis-related genes in tamoxifen-treated patients with estrogen receptor-positive breast cancer. Biosci Rep 2021;41:BSR20203020. [Crossref] [PubMed]
- Yang Z, Sun Q, Guo J, et al. GRSF1-mediated MIR-G-1 promotes malignant behavior and nuclear autophagy by directly upregulating TMED5 and LMNB1 in cervical cancer cells. Autophagy 2019;15:668-85. [Crossref] [PubMed]
- Sun Q, Yang Z, Li P, et al. A novel miRNA identified in GRSF1 complex drives the metastasis via the PIK3R3/AKT/NF-κB and TIMP3/MMP9 pathways in cervical cancer cells. Cell Death Dis 2019;10:636. [Crossref] [PubMed]
- Wang B, Wang L, Lu Y, et al. GRSF1 promotes tumorigenesis and EMT-mediated metastasis through PI3K/AKT pathway in gastric cancer. Biochem Biophys Res Commun 2021;555:61-6. [Crossref] [PubMed]
- Han L, Huang C, Wang X, et al. The RNA-binding protein GRSF1 promotes hepatocarcinogenesis via competitively binding to YY1 mRNA with miR-30e-5p. J Exp Clin Cancer Res 2022;41:17. [Crossref] [PubMed]
- Li T, Fan J, Wang B, et al. TIMER: A Web Server for Comprehensive Analysis of Tumor-Infiltrating Immune Cells. Cancer Res 2017;77:e108-10. [Crossref] [PubMed]
- Chandrashekar DS, Bashel B, Balasubramanya SAH, et al. UALCAN: A Portal for Facilitating Tumor Subgroup Gene Expression and Survival Analyses. Neoplasia 2017;19:649-58. [Crossref] [PubMed]
- Asplund A, Edqvist PH, Schwenk JM, et al. Antibodies for profiling the human proteome-The Human Protein Atlas as a resource for cancer research. Proteomics 2012;12:2067-77. [Crossref] [PubMed]
- Györffy B, Lanczky A, Eklund AC, et al. An online survival analysis tool to rapidly assess the effect of 22,277 genes on breast cancer prognosis using microarray data of 1,809 patients. Breast Cancer Res Treat 2010;123:725-31. [Crossref] [PubMed]
- Gyorffy B, Lánczky A, Szállási Z. Implementing an online tool for genome-wide validation of survival-associated biomarkers in ovarian-cancer using microarray data from 1287 patients. Endocr Relat Cancer 2012;19:197-208. [Crossref] [PubMed]
- Tang Z, Kang B, Li C, et al. GEPIA2: an enhanced web server for large-scale expression profiling and interactive analysis. Nucleic Acids Res 2019;47:W556-60. [Crossref] [PubMed]
- Cerami E, Gao J, Dogrusoz U, et al. The cBio cancer genomics portal: an open platform for exploring multidimensional cancer genomics data. Cancer Discov 2012;2:401-4. [Crossref] [PubMed]
- Ghandi M, Huang FW, Jané-Valbuena J, et al. Next-generation characterization of the Cancer Cell Line Encyclopedia. Nature 2019;569:503-8. [Crossref] [PubMed]
- Vasaikar SV, Straub P, Wang J, et al. LinkedOmics: analyzing multi-omics data within and across 32 cancer types. Nucleic Acids Res 2018;46:D956-63. [Crossref] [PubMed]
- Thomas PD. The Gene Ontology and the Meaning of Biological Function. Methods Mol Biol 2017;1446:15-24. [Crossref] [PubMed]
- Kanehisa M, Furumichi M, Tanabe M, et al. KEGG: new perspectives on genomes, pathways, diseases and drugs. Nucleic Acids Res 2017;45:D353-61. [Crossref] [PubMed]
- Yu G, Wang LG, Han Y, et al. clusterProfiler: an R package for comparing biological themes among gene clusters. OMICS 2012;16:284-7. [Crossref] [PubMed]
- Eden A, Gaudet F, Waghmare A, et al. Chromosomal instability and tumors promoted by DNA hypomethylation. Science 2003;300:455. [Crossref] [PubMed]