PDX1 associated therapy in translational medicine
PDX1 and PDX1 involved diseases
Pancreatic and duodenal homeobox 1 (PDX1), also known as insulin promoter factor 1 (IPF1), is a homeodomain-containing transcription factor specific for pancreatic islet β cells. PDX1 is essential for differentiation and maturation of pancreatic precursor cells towards β cells in the developing pancreas, and it is also the key regulator of insulin gene expression for postnatal β cell functions (1,2). During mouse early embryonic development, PDX1 expression can be firstly detected at embryonic day 8.5 in the dorsal and ventral buds that form pancreas; while in the adult stage, PDX1 is mainly expressed and functions in the β cells of the islet. It is known that PDX1 maintains mature β cell function and glucose metabolism through regulating the expression of multiple key endocrine β-cell-specific genes including insulin, glucokinase, islet amyloid polypeptide and the glucose transporter type 2. Deletion or homozygous inactive mutations in PDX1 is lethal in mice due to whole pancreatic agenesis (2,3). Conditional knockout of PDX1 gene in β cells leads to overt diabetes, whereas knocking-down of PDX1 expression results in decreased insulin secretion in mice (4). PDX1 mutant zebrafish also exhibit several key diabetic features including reduced β cell mass, decreased insulin and elevated glucose levels. In humans, heterozygous mutations in PDX1 have been associated with diabetes, including type 4 maturity-onset diabetes of the young (MODY IV) and non-MODY type 2 diabetes. Overall, PDX1 has been demonstrated to directly regulate glucose-dependent insulin expression and secretion.
Moreover, aberrant elevated PDX1 expression in pancreatic ductal adenocarcinoma (PDAC) and pancreatic neuroendocrine tumors (PNETs), including insulinoma, strongly suggested that PDX1 as a fundamental transcriptional factor for pancreas development might also play an important role in promoting tumorigenesis (5-7). So far, PDX1 has been proved to promote KrasG12D oncogenic protein-induced development of PanIN, metaplasia and PDAC. Overexpression of PDX1 in benign human cells (HEK293 and Human pancreatic ductal cells), as well as PDAC cell lines (PANC1 and MiaPaca2) and insulinoma cell lines (Min6 and βTC6 cells) lead to significant increases in cell proliferation, invasion and colony formation in vitro, as well as promotion of tumor growth in xenograft severe combined immunodeficiency (SCID) mouse models (8-12). These studies suggest that PDX1 is involved in tumorigenesis of both PDAC and insulinoma.
PDX1 promotes PDAC tumorigenesis
PDX1 expression is essentially restricted to the islet β-cells in adult. However, over-expression of PDX1 has been detected in a number of human cancers. In PDAC, PDX1 expression level is markedly elevated in comparison to normal tissues and the expression level of PDX1 is significantly correlated with metastasis, TNM grading, and tumor cell proliferation of pancreatic cancer. The patients with positive PDX1 expression have a significantly worse prognosis than those patients with negative PDX1. Therefore, PDX1 expression can be used as an independent survival factor in PDAC. In addition to PDAC, PDX1 is also aberrantly over-expressed in other types of cancers, including prostate cancer, colorectal cancer, gastric cancer, kidney cancer, pediatric solid pseudopapillary tumors, PNETs and nesidioblastosis, a hyperinsulinemic hypoglycemia attributed to excessive function of pancreatic β cells.
During adulthood, PDX1 expression is mainly restricted to islet β-cells in the pancreas. However, the marked PDX1 overexpression in almost all solid tumors and the significant correlation of PDX1 expression levels with clinic parameters of cancers strongly suggest that the overexpressed PDX1 plays a role in tumorigenesis. Our previous data have shown that overexpression of PDX1 in benign HEK293 cells and normal human pancreatic ductal epithelial (HPDE) cells results in increased cell proliferation, invasion and colony formation in vitro and promotes tumor formation of implanted HEK293 and HPDE cells in SCID mice. These studies indicate that PDX1 is able to transform benign cells. Moreover, overexpression of PDX1 in malignant human pancreatic cancer MiaPaCa2 cells leads to significant increase of cell proliferation, invasion and colony formation in vitro and promotes tumor growth of implanted PDAC MiaPaca2 cells in SCID mice in comparison to parental MiaPaca2 cells. Forced expression of PDX1 in PANC1 cells results in a significant higher migration rate. Thus, PDX1 has been proved to enhance the tumorigenicity of malignant PDAC cells. Indeed, persistent expression of PDX1 induces metaplasia. Upregulated PDX1 expression is observed in premalignant metaplastic ductal epithelium during transforming growth factor (TGF)-α—induced pancreatic ductal metaplasia and neoplasia. PDX1 is also required for KRasG12D-induced development of PanIN, metaplasia and PDAC. All these findings strongly suggest that PDX1 is an oncogenic transcription factor for PDAC.
PDX1 and insulinoma
PNETs are a group of rare tumors that develop from specialized cells known as neuroendocrine cells within the pancreas, with approximately 1 in 100,000 people being affected per year in the general population. There are two broad categories of PNETs: functioning and non-functioning. Functioning tumors with secretes hormones are further subtyped according to the hormone that they secrete. These include insulinomas, gastrinomas, VIPomas, glucagonomas, and somatostatinoma. The symptoms associated with PNETs are highly variable and depend on whether the tumor is functioning or non-functioning. Individuals with functioning tumors are more likely to present with symptoms associated with excess hormone production (13-15). The special type of functioning PNET insulinoma primarily raised from islet β cells that produce insulin, occurring in only 3–4 per 1,000,000 people. However, these patients with insulinoma suffer horribly from uncontrollable hypoglycemia because the expanded β cell mass continues to secrete insulin and disrupt the glucose regulation, causing severe hyperinsulinemic hypoglycemia, for which there is no effective treatment. Nesidioblastosis in adults is a complication of Roux-en-Y gastric bypass surgery requiring recurrent hospitalizations for hyperinsulinemic hypoglycemia. Congenital nesidioblastosis is also commonly referred to as persistent hyperinsulinemic hypoglycemia of infancy. A number of genetic mutations have been associated with nesidioblastosis. The abnormal histologic aspects of the tissue included the presence of islet cell enlargement, islet cell dysplasia, β cells budding from ductal epithelium, and islets in apposition to ducts. Patients with severe cases can manifest as cyanosis or seizures, which can then lead to developmental delay if brain damage occurs. As a vital islet β cell specific transcription factor, PDX1 was found significantly overexpressed in both human insulinoma specimens and mouse insulinoma cell lines, suggesting a potential role of PDX1 in promoting insulinoma. To determine the role of PDX1 in insulinoma, a RNA interference (RNAi) therapy utilizing PDX1-shRNA has been developed and knocking down of PDX1 expression using bi-functional shRNA resulted in significant inhibition of insulin expression, PDX1 expression and glucose-stimulated insulin secretion, as well as cell proliferation in mouse βTC6 cells. Nanoparticle capsulated bishRNA-PDX1 prevented death from severe hyperinsulinemic hypoglycemia in insulinoma SCID mice. Therefore, PDX1 plays a significant role in regulating insulin synthesis and the expression level correlates with its insulinoma. A novel therapeutic strategy targeting PDX1 gene has been proved effective in preventing mortality for hyperinsulinemic hypoglycemia in an insulinoma mouse model.
PDX1 and diabetes mellitus
Diabetes mellitus has been cited as one of the most challenging health problems in the US. It is a group of metabolic disorders characterized by hyperglycemia, in which the patients have very high blood glucose over a prolonged period of time, because of either inadequate insulin secretion, or improper responses to insulin, or both. As the most important regulator for insulin regulation, it is known that PDX1 associated with diabetes and is a master regulator for insulin and multiple key endocrine β-cell-specific genes including glucokinase, islet amyloid polypeptide in glucose hemostasis. Animal study showed that complete or partial loss function of PDX1 in mice leads to whole pancreatic agenesis and embryonic lethal. Tissue specific gene targeting of PDX1 in β cells of mice leads to overt diabetes, whereas knocking-down of PDX1 expression using RNAi results in decreased insulin secretion (16). Moreover, PDX1 mutant zebrafish have the similar diabetic features of loss of β cells, decreased insulin and elevated glucose levels. While in humans, heterozygous mutations in PDX1 have been reported to associate with diabetes, including both type 4 MODY IV and non-MODY type 2 diabetes. In addition to diabetes, abnormal PDX1 expression is also involved in other pathophysiologic conditions, such as chronic pancreatitis with decreased PDX1 expression. Overall, PDX1 as one of the most important insulin gene regulators has been shown to directly regulate glucose-dependent insulin expression and secretion.
PDX1 targeted therapy in translational medicine
PDX1 has been shown to have oncogenic properties in PDAC and insulinoma with potential to be a promising therapeutic target for these types of tumors. Therefore, we developed three types of PDX1 targeted therapies (Figure 1) and tested their translational potential in various preclinical studies.
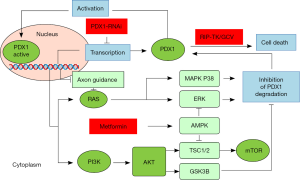
PDX1-RNAi effectively silences PDX1 expression and ablates human PDAC tumors in vivo
RNAi is a promising new form of targeted gene therapy with great potentials for translational medicine. RNAi has been uncovered as a naturally occurring regulatory mechanism in mammals. Conventional siRNA generated from double-stranded RNA molecules can bind target RNA sequences and interfere with their translation or target them for destruction (17). Having demonstrated PDX1 is a potential gene target for PDAC, we developed our first therapeutic platform targeting PDX1 using a novel RNAi technology bi-functional shRNA (11,18,19). The bi-functional shRNA consists of two stem-loop shRNA (short-hairpin RNA) structures: one cleavage-dependent unit with a perfectly matched passenger-strand and guide-strand, and one cleavage-independent unit composed of a mismatched double strand. It is able to induce both RNase-H like cleavage and non-cleavage mediated degradation of the target mRNA and inhibit translation concurrently, leading to more rapid onset of gene silencing, higher efficacy and greater durability (17,20-22). We designed and tested a series of bi-shRNAPDX1 vectors and demonstrated in vitro that bi-shRNAPDX1 was much more effective than conventional shRNAPDX1 in silencing PDX1 gene expression in PDAC cells. After that, we further tested the in vivo therapeutic effects of bi-shRNAPDX1 that three biweekly cycles of systemically delivered bi-shRNAPDX1 nanoplexes significantly ablated human PDAC tumors in SCID mice (5,7). Intravenous infusions of bi-shRNAPDX1 nanoplexes in a large bio-relevant Yucatan mini-pig model were associated minimal toxicity (23). Therefore, these preclinical studies using RNAi targeting PDX1 gene suggest that RNAi therapy holds great promise to serve as an adjuvant, targeted therapy for PDAC.
RIP-TK therapy specific targets PDX1 positive PDAC cells
Gene therapy treats disease cells at the molecular level by correcting the mutated gene or by suicide gene therapy, which terminates the disease cells. Despite its promise in numerous preclinical studies and clinical trials, it has seen only limited translational success. While there are a myriad of reasons limiting its translational potential, with the main limitation being the lack of an ideal delivery system, the field of gene therapy continues to move forward to develop effective targeted gene therapies for cancer. We have developed a gene therapy strategy using a synthetic gene promoter that is activated by transcription factors only in the target cancer cell using a novel DOTAP: cholesterol liposome system, which allows effective delivery and specific targeting to PDX1 in PDAC cells. We utilized a synthetic insulin promoter, which is activated only by PDX1, to drive the cytotoxic viral thymidine kinase (TK) gene. Once expressed in the PDAC cell, TK is coupled with its prodrug, ganciclovir, to induce cell death. Using this gene therapy strategy, we demonstrated that our synthetic cytotoxic gene construct RIP-TK could be given intravenously to significantly reduce the growth of human PDAC xenograft tumors in mice. The novel liposomal delivery system allows repeated intravenous infusions of our cytoxic gene and significantly enhances the therapeutic effects with minimal toxicity in vivo. We have since developed more powerful synthetic promoters that are specific for PDAC and delivered by viruses that are highly specific for PDAC cells and are testing these advances in gene therapy in mouse models. Thus, these preclinical studies suggest that RIP-RK gene therapy targeting PDX1 expressing cells, followed by ganciclovir, holds promise to serve as an adjuvant, targeted therapy for PDAC.
Metformin targets PDX1 via inhibiting ERK signaling
Having developed bi-shRNAPDX1 and RIP-TK PDX1 targeted therapeutic platforms that effectively inhibit PDX1, we sought to identify novel small molecular compounds for PDX1 using high-throughput drug screening technology on PDAC cell lines. To achieve this goal, we designed and engineered powerful synthetic human insulin promoter (SHIP) with excellent dynamic range and sensitivity to drive firefly luciferase reporter gene to reflect inhibition or activation of the transcription factor-promoter complex of the target gene (PDX1). Small-molecule libraries including an FDA approved drug library were then applied to the stably transfected cells plated on 384-well plates to search for small molecules suppressing the transcription factor-promoter complex of the target gene (PDX1) within the PDAC cell. The FDA approved drug library consists of 2,100 drugs from the NIH clinical collection (I and II; version 5) that could be repurposed for PDAC therapy. Repurposing of drugs is of great interest, as it reduces costs of drug research and development and saving years of drug development time. We have successfully identified a list of small molecular inhibitor targeting PDX1, one of which is metformin. Metformin is the most widely prescribed diabetes drug worldwide and it has been associated with a substantially lower risk of all-cancer mortality in multiple studies and meta-epidemiological analyses and is emerging as a promising anticancer drug in clinical trials at various stages (24). Our team demonstrated that metformin suppresses growth of PDAC cells via inhibition of PDX1 via a variety of adenosine monophosphate (AMP)-activated protein kinase (AMPK)-dependent and/or AMPK-independent mechanisms. We thus identified a potential novel mechanism by which metformin exerts its anticancer action through down-regulating PDX1 (25,26).
Future perspectives
PDX1 targeted therapies have been proved to effectively ablate PDX1 positive insulinoma and PDAC cancer cells both in vitro and in vivo. Our team has been translating novel targeted therapies for cancers and has identified PDX1 as a promising therapeutic target for both PDAC and insulinoma. To do so, we are developing effective PDX1 targeted therapeutic platforms utilizing RNAi, RIP-TK gene therapy and small inhibitory compounds. The preclinical studies are promising and we have embarked on the arduous path to translate these studies via clinical trials. We hope that the development of translational therapies will bring more effective, targeted adjuvant therapies and thus prolong survival for pancreatic cancer and insulinoma.
Acknowledgements
Funding: The study was funded by the National Institutes of Health grants NIDDK R01-DK46441 and NCI R01-CA095731 and the Ann and Jerry Moss Foundation.
Footnote
Conflicts of Interest: The authors have no conflicts of interest to declare.
References
- Ashizawa S, Brunicardi FC, Wang XP. PDX-1 and the pancreas. Pancreas 2004;28:109-20. [Crossref] [PubMed]
- Feanny MA, Fagan SP, Ballian N, et al. PDX-1 expression is associated with islet proliferation in vitro and in vivo. J Surg Res 2008;144:8-16. [Crossref] [PubMed]
- Liu T, Wang CY, Gou SM, et al. PDX-1 expression and proliferation of duct epithelial cells after partial pancreatectomy in rats. Hepatobiliary Pancreat Dis Int 2007;6:424-9. [PubMed]
- Gidekel Friedlander SY, Chu GC, Snyder EL, et al. Context-dependent transformation of adult pancreatic cells by oncogenic K-Ras. Cancer Cell 2009;16:379-89. [Crossref] [PubMed]
- Liu S, Ballian N, Belaguli NS, et al. PDX-1 acts as a potential molecular target for treatment of human pancreatic cancer. Pancreas 2008;37:210-20. [Crossref] [PubMed]
- Liu SH, Patel S, Gingras MC, et al. PDX-1: demonstration of oncogenic properties in pancreatic cancer. Cancer 2011;117:723-33. [Crossref] [PubMed]
- Liu SH, Rao DD, Nemunaitis J, et al. PDX-1 is a therapeutic target for pancreatic cancer, insulinoma and islet neoplasia using a novel RNA interference platform. PLoS One 2012;7:e40452. [Crossref] [PubMed]
- Liu SH, Davis A, Li Z, et al. Effective ablation of pancreatic cancer cells in SCID mice using systemic adenoviral RIP-TK/GCV gene therapy. J Surg Res 2007;141:45-52. [Crossref] [PubMed]
- Tirone TA, Wang XP, Templeton NS, et al. Cell-specific cytotoxicity of human pancreatic adenocarcinoma cells using rat insulin promoter thymidine kinase-directed gene therapy. World J Surg 2004;28:826-33. [Crossref] [PubMed]
- Wang XP, Yazawa K, Yang J, et al. Specific gene expression and therapy for pancreatic cancer using the cytosine deaminase gene directed by the rat insulin promoter. J Gastrointest Surg 2004;8:98-108; discussion 106-8. [Crossref] [PubMed]
- Wu J, Liu S, Yu J, et al. Vertically integrated translational studies of PDX1 as a therapeutic target for pancreatic cancer via a novel bifunctional RNAi platform. Cancer Gene Ther 2014;21:48-53. [Crossref] [PubMed]
- Wu JX, Liu SH, Nemunaitis JJ, et al. Liposomal insulin promoter-thymidine kinase gene therapy followed by ganciclovir effectively ablates human pancreatic cancer in mice. Cancer Lett 2015;359:206-10. [Crossref] [PubMed]
- Batcher E, Madaj P, Gianoukakis AG. Pancreatic neuroendocrine tumors. Endocr Res 2011;36:35-43. [Crossref] [PubMed]
- Dadan J, Wojskowicz P, Wojskowicz A. Neuroendocrine tumors of the pancreas. Wiad Lek 2008;61:43-7. [PubMed]
- Ito T, Igarashi H, Jensen RT. Pancreatic neuroendocrine tumors: clinical features, diagnosis and medical treatment: advances. Best Pract Res Clin Gastroenterol 2012;26:737-53. [Crossref] [PubMed]
- Jonsson J, Carlsson L, Edlund T, Edlund H. Insulin-promoter-factor 1 is required for pancreas development in mice. Nature 1994;371:606-9. [Crossref] [PubMed]
- Phalon C, Rao DD, Nemunaitis J. Potential use of RNA interference in cancer therapy. Expert Rev Mol Med 2010;12:e26. [Crossref] [PubMed]
- Nemunaitis J, Rao DD, Liu SH, et al. Personalized cancer approach: using RNA interference technology. World J Surg 2011;35:1700-14. [Crossref] [PubMed]
- Senzer N, Barve M, Kuhn J, et al. Phase I trial of "bi-shRNAi(furin)/GMCSF DNA/autologous tumor cell" vaccine (FANG) in advanced cancer. Mol Ther 2012;20:679-86. [Crossref] [PubMed]
- Rao DD, Senzer N, Cleary MA, et al. Comparative assessment of siRNA and shRNA off target effects: what is slowing clinical development. Cancer Gene Ther 2009;16:807-9. [Crossref] [PubMed]
- Rao DD, Maples PB, Senzer N, et al. Enhanced target gene knockdown by a bifunctional shRNA: a novel approach of RNA interference. Cancer Gene Ther 2010;17:780-91. [Crossref] [PubMed]
- Rao DD, Vorhies JS, Senzer N, et al. siRNA vs. shRNA: similarities and differences. Adv Drug Deliv Rev 2009;61:746-59. [Crossref] [PubMed]
- Jay CM, Ruoff C, Kumar P, et al. Assessment of intravenous pbi-shRNA PDX1 nanoparticle (OFHIRNA-PDX1) in yucatan swine. Cancer Gene Ther 2013;20:683-9. [Crossref] [PubMed]
- Gong J, Robbins LA, Lugea A, et al. Diabetes, pancreatic cancer, and metformin therapy. Front Physiol 2014;5:426. [Crossref] [PubMed]
- Sinnett-Smith J, Kisfalvi K, Kui R, et al. Metformin inhibition of mTORC1 activation, DNA synthesis and proliferation in pancreatic cancer cells: dependence on glucose concentration and role of AMPK. Biochem Biophys Res Commun 2013;430:352-7. [Crossref] [PubMed]
- Zhou G, Yu J, Wang A, et al. Metformin Restrains Pancreatic Duodenal Homeobox-1 (PDX-1) Function by Inhibiting ERK Signaling in Pancreatic Ductal Adenocarcinoma. Curr Mol Med 2016;16:83-90. [Crossref] [PubMed]