Research progress on the mechanism of action of hesperetin in cerebral ischemia: a narrative review
Introduction
Ischemic cerebral vascular disease (ICVD) is one of the major diseases threatening human health and survival. ICVD is a common neurological disorder that leads to a high rate of disability among patients and requires therapeutic management and prevention of complications (1-3). The risk factors of cerebral ischemia are atherosclerosis, hypertension, hyperlipidemia, and blood viscosity caused by increased platelet levels (4). Treatment based on these risk factors can prevent the occurrence of cerebral ischemia. Following the occurrence of cerebral ischemia, reinstating the blood flow or enhancing the blood supply in the ischemic area is mandatory for repairing the damage to ischemic brain tissue; however, this may also result in reperfusion injury. The so-called ischemia-reperfusion injury refers to the gradually aggravated tissue injury caused by the restoration of blood perfusion after organ ischemia for a certain period of time. Recent research has reported that the pathogenesis of cerebral ischemia-reperfusion injury includes oxidative stress (OS), inflammatory reaction, nitric oxide injury, apoptosis, excitatory amino acid toxicity, calcium (Ca2+) overload, etc. (Figure 1) (5). Effective therapy can reduce brain tissue necrosis to alleviate the symptoms associated with cerebral ischemia-reperfusion injury.
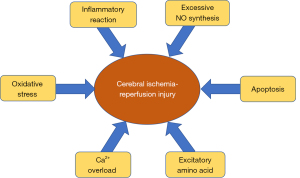
Traditional Chinese medicine (TCM) has a documented history of more than 2,000 years, and through ancient practice and continual understanding, a rich database of natural and traditional therapeutic compounds has been gradually accumulated (6). In recent years, studying the effective ingredients in TCM has become a particular focus of research attention, which aims to formulate new therapeutic measures for the treatment and prevention of many diseases. Hesperetin, an important natural and traditional medicinal ingredient, is reportedly present in quite high concentrations in numerous medicinal sources such as Pericarpium citri reticulatae, Poncirus trifoliate shell, Poncirus trifoliate fruit, and Pericarpium citri reticulatae viride (7). It has varied therapeutic effects and multiple mechanisms of action, including anti-atherosclerosis, anti-OS, anti-inflammatory reaction, anti-apoptosis, carbon monoxide regulation, anti-hyperlipidemia, and anti-thrombosis (8-12), which can be applied for the prevention and treatment of cerebral ischemia-reperfusion injury. Moreover, studies have shown that hesperetin is not accumulated in any organ, and is a very safe pharmacological component without obvious side effects (13,14). Khan et al. summarized the antioxidant and anti-inflammatory effects of hesperetin on neurological disorders (15); Ferreira de Oliveira et al. summarized the therapeutic potential of hesperidin in cancer cell cycle regulation and apoptosis induction (16); and Iranshahi et al. summarized the antibacterial effect of hesperidin both in vitro and in vivo (17). The current reviewed paper provides a deeper insight into the mechanisms of action and molecular targets of Hesperetin and shows the gaps in our knowledge about Hesperetin. However, there are currently no reports or relevant articles on the treatment of cerebral ischemia with hesperetin and its clinical application. We believe that hesperetin will be a new therapeutic drug with significant potential value and an effective remedy for cerebral ischemic injury. In this paper, we review the complex and multi-targeted mechanism of action of hesperetin to provide theoretical support for better and more reasonable use of hesperetin in the treatment of cerebral ischemia. We present the following article in accordance with the Narrative Review reporting checklist (available at https://atm.amegroups.com/article/view/10.21037/atm-22-3136/rc).
Methods
We conducted a literature search for published manuscripts hesperidin in ischemia/reperfusion up to December 2021 in common English databases (i.e., PubMed, EMBASE, Web of Science, SpringerLink, Wiley, Cochrane Library) and Chinese databases [Chinese BioMedical Literature Service System (CBM), WANFANG database, China Knowledge Resource Integrated Database (CNKI)]. The following search words and terms: “ischemia/reperfusion” OR “cerebral ischemia” OR “brain ischemia,”, and “hesperetin” were used in this review. The qualitative and quantitative analyses were conducted in parallel by interpreting each paper to avoid missing potentially valuable data (Table 1).
Table 1
Items | Specification |
---|---|
Date of search (specified to date, month and year) | December 30, 2021 |
Databases and other sources searched | English databases (i.e., PubMed, EMBASE, Web of Science, Cochrane Library) and Chinese databases [Chinese BioMedical Literature Service System (CBM), WANFANG database, China Knowledge Resource Integrated Database (CNKI)] |
Search terms used (including MeSH and free text search terms and filters) | “ischemia/reperfusion” OR “cerebral ischemiainjury” OR “brain ischemia” and “hesperetin” |
Timeframe | 1970–2021 |
Inclusion and exclusion criteria (study type, language restrictions etc.) | The inclusion criteria: (I) studies written in English and Chinese; (II) studies that assessed the correlation between hesperetin and ischemic stroke; III) studies that the mechanism of hesperetin in ischemic stroke |
The exclusion criteria: (I) publications with duplications or studies with overlapping data from the same author; (II) abstracts, case reports, proceedings, letters, reviews, and meta-analyses; (III) incomplete outcome data | |
Selection process (who conducted the selection, whether it was conducted independently, how consensus was obtained, etc.) | Two reviewers independently extracted the data from each included study using a standard criterion |
Any additional considerations, if applicable | No |
Discussion
Overview of ischemic stroke
Stroke can be broadly classified into two types: ischemic stroke and hemorrhagic stroke. Among these, ischemic stroke is the most common, accounting for nearly 80% of all stroke cases (18). The pathogenesis of ischemic stroke is very complicated, and given its high rates of morbidity, disability, mortality, and recurrence, as well as its considerable economic burden, it seriously threatens the safety and quality of human life. Ischemic stroke is a general term for brain tissue necrosis caused by the stenosis or occlusion of arterial blood supply (carotid and vertebral arteries) and insufficient blood supply to the brain. Tissue plasminogen activator, intravenous thrombolysis, and intravascular mechanical thrombectomy (19-21) are currently used for the treatment of ischemic stroke, but due to the reduced scope for widespread application and lower safety profiles, their clinical application is highly restricted. In recent years, a previous study has shown that the incidence of cerebral ischemia is the result of the interaction of multiple pathophysiological features, such as OS, apoptosis, inflammatory reaction, calcium overload, and blood-brain barrier damage (22). Due to the complexity and variability of its pathological process, a single drug component is inadequate to control and resolve the overall problem; hence, there is a pressing need for clinically useful drugs, which are significantly efficacious and have a reduced side effect profile, for the treatment of cerebral ischemia. TCM has been increasingly explored for the prevention and treatment of cerebral ischemia in recent years, owing to its complex composition and intervention in the pathological process of cerebral ischemia via multiple targets and pathways.
Cerebral ischemia-reperfusion injury refers to the phenomenon of brain cell damage following an ischemic response; in this condition, restoration of the normal function of tissues and organs is not achieved or improved to a certain extent and the ischemic injury is further aggravated after the recovery of blood reperfusion. The main mechanisms of cerebral ischemia-reperfusion injury are OS, apoptosis, excessive nitric oxide (NO) synthesis, inflammatory reaction, Ca2+ overload, and excitatory amino acids. Unraveling the complex mechanism of cerebral ischemia-reperfusion injury can help to establish effective therapeutic strategies and may provide a reference for the effective management of this condition.
Overview of hesperetin
Basic situation of hesperetin
Hesperetin is a flavanone compound. It is a kind of TCM extract that exists in the form of Hesperidin in nature (23), which is hydrolyzed to produce hesperetin under the action of human intestinal flora and exerts a pharmacodynamic effect (Figure 2). Hesperetin is a natural ingredient that is present in a variety of medicinal plants including clematis, citrus, chrysanthemum, and others (24) and is more abundantly available in citrus. Its concentration is quite high in various medicinal sources, such as Pericarpium citri reticulatae, Poncirus trifoliate shell, Poncirus trifoliate fruit, and Pericarpium citri reticulatae viride, in which it is a predominant ingredient (7). The relative molecular mass of hesperetin is 302.28, its molecular formula is C16H14O6, and its melting point is 227.5 °C. It has poor water solubility (273 mg/L) and is easy to dissolve in organic reagents. The structure of hesperetin contains multiple reactive radicals, such as ketone, carbonyl, ether, methoxy, as well as numerous phenol-hydroxyl groups, making it a potent biological molecule with a broad pharmacological effect (25). Hesperetin is not accumulated in any organ; it is safe to use and does not exhibit any obvious side effects (8-12). Moreover, it has multiple functions, including anti-OS, anti-platelet aggregation, vasodilation, anti-atherosclerosis, anti-inflammation, anti-apoptosis, anti-hyperlipidemia, anti-tumor, cardiovascular protection, and regulation of nitric oxide release (26-29). In recent years, reports have shown that hesperetin and its derivatives exert therapeutic effects such as anti-Alzheimer’s disease, anti-Parkinson’s disease, anti-hyperglycemia, anti-hemocoagulase, and anti-fibrosis of the lung, kidney, and liver, as well as inhibition of some new tumors (22,30-35).
There are several methods for extracting hesperetin from orange peel, including solvent extraction, aqueous two-phase extraction, alkaline extraction-acid precipitation, and ultrasound (36). The extracted material is a mixture, and the next step requires purification of hesperetin via macroporous resin adsorption, ion exchange, high-speed countercurrent chromatography, or high-performance liquid chromatography. Hesperetin is an important flavonoid; it is non-toxic, safe, and reliable. Furthermore, it also has spectral pharmacological functions, including antibacterial, antioxidant, anti-cancer, expectorant, immune regulation, cardiovascular protection, etc. (37,38). However, owing to its poor water solubility, the application of hesperetin is limited. Therefore, the chemical modification of hesperetin to improve its hydrophilicity is currently a research hotspot. Researchers have methylated the 7-position hydroxyl group of hesperetin, transformed hesperetin into its sulfonated sodium salt, or coordinated hesperetin with metal ions to improve its water solubility (39,40). At present, hesperetin is used clinically as an auxiliary drug for the treatment of cardiovascular diseases and diabetes. In addition, since hesperetin has a selective inhibitory effect on cancer cells and has no effect on normal human cells, it can be used as an auxiliary drug for the treatment of cancer patients who are resistant to chemotherapy and radiotherapy (41,42).
There are few published domestic research reports related to hesperetin but are instead predominantly focused on hesperidin, such as its drug efficacy, production technology, and so on. However, with the continuous development of clinical medicine, modern pharmaceutical research has revealed detectable levels of hesperetin in the blood, urine, and feces of mice following ingestion over a specified period. Surprisingly, no trace of hesperidin was detected in any of the mice samples tested. Further investigation has determined that when using intestinal microorganisms for the degradation of flavonoid glycosides in vitro, hesperidin will only change marginally after it is degraded to hesperetin. These experimental findings have confirmed that most of the hesperidin is hydrolyzed to hesperetin via intestinal microbial flora-induced metabolism, which is then absorbed into the blood through the intestinal tract and transported to various tissues and organs to exert its clinical effects (43). The basis of hesperidin’s effect in vivo is probably the effect of its metabolic decomposition into hesperetin, and thus, exploring the role of hesperetin in the human body is crucially important.
Advantages/disadvantages of hesperetin
Hesperetin exerts numerous pharmacological effects, but due to its poor water solubility and low biological activity, its clinical application is considerably limited (44). To improve the biological activity of hesperetin and enhance its clinical effects, some researchers have used the synthetic derivatives of hesperetin and its pharmaceutical preparations through the application of biological nanotechnology in recent years, which has improved the water solubility and bioavailability of hesperetin. Ding et al. and Wang et al. synthesized a series of hesperetin derivatives and evaluated their anti-inflammatory activity, and found that the derivatives exhibit higher anti-inflammatory activity than hesperetin (45,46). Mary Lazer et al. synthesized hesperetin chitosan folic acid nanoparticles (CHF) and evaluated the half maximal inhibitory concentration (IC50) values of hesperetin and CHF on human colon cancer HCTl5 cells (47). Their results showed that the IC50 value of hesperetin was 190 µmol/L, while that of CHF was only 28 µmol/L, which confirmed that the application of nanotechnology improved the water solubility of hesperetin and also greatly enhanced its bioavailability.
Mechanism of hesperetin in the treatment of cerebral ischemia
Anti-OS and apoptosis
OS is a pathophysiologic phenomenon that is caused by the excessive production of reactive oxygen species (ROS) due to the external influence of cells in vivo. Thus, OS leads to an imbalance between oxidation and the antioxidant system and makes the system tend toward oxidization, resulting in cell damage. This concept was first proposed by Paniker et al. (48). In cerebral ischemia-reperfusion injury, OS can damage nerve cells via direct ROS damage and the activation of other signaling pathways. The direct ROS damage includes damage to membranes caused by lipid peroxidation (LPO), oxidation and deactivation of enzymes, and the modification of nitrogenous bases of chromosomal deoxyribonucleic acid (DNA), which all may directly affect the survival and development of cells and lead to cell damage. Indirect ROS damage to cells is mainly activated through the mitochondrial-mediated apoptosis pathway regulated by altering mitochondrial pore permeability (49). ROS can also damage nerves during cerebral ischemia-reperfusion injury by affecting transcription factors (50).
ROS is related to the pathogenesis of neurodegenerative diseases. Similarly, the transcription factors, nuclear factor erythroid 2-related factor 2 (Nrf-2) and heme oxygenase-1 (HO-1), are the main cellular mechanisms regulating antioxidant and cytoprotective genes, which are down-regulated in neurodegenerative diseases. Lipopolysaccharide (LPS) induces ROS production, resulting in oxidant/antioxidant imbalance and OS (51-54). The results reported by Muhammad et al. showed that LPS promotes OS by inducing the production of LPO and ROS in mouse brains and HT-22 cells (55). Their western blotting results showed that LPS also down-regulated the expression of Nrf-2 and HO-1 in the mouse brain. In the hesperetin-treated group, the increased expression of LPO and ROS and the decreased expression of Nrf-2 and HO-1 were significantly regulated, indicating that hesperetin exerts an antioxidant effect on mouse brain cells (Figure 3).
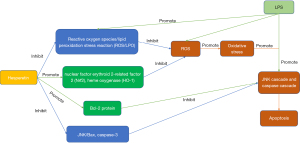
Apoptosis refers to the spontaneous and orderly cell death controlled by genes to maintain the stability of the internal environment. In the apoptotic system, the B cell lymphoma-2 (Bcl-2) and Caspase families play a key role in receiving, transmitting, or expanding apoptotic signals inside or outside the cell or when the cell executes apoptosis commands, and are crucial to the regulation of apoptosis (56). The Bcl-2 family can be divided into two categories: anti-apoptosis proteins and pro-apoptotic proteins, and Bcl-2 and Bcl-2 associated X protein (Bax) have been extensively investigated. Studies have shown that the expression levels of the two regulatory proteins directly determine the apoptosis and survival of cells following external stimulus (57,58). The Caspase family comprises 14 subtypes:
Type I (Inflammation) includes Caspase-1, Caspase-4, Caspase-5, Caspase-11, Type II (apoptosis initiators) includes Caspase-8, Caspase-9, Caspase-10; Type II (apoptosis executors) includes Caspase-3, Caspase-6, Caspase-7; Type III (response regulators) includes Caspase-2, Caspase-12, Caspase-14, etc. (59). Liang et al. found that the Bcl2 protein levels decreased, while those of Bax increased, in an animal model of cerebral ischemia-reperfusion (60). Liu et al. also found that the Caspase-3 protein levels increased in an animal model of cerebral ischemia-reperfusion (61). Therefore, apoptosis is involved in the occurrence and development of cerebral ischemia-reperfusion injury.
c-Jun N-terminal kinase (JNK), a stress kinase, is involved in the apoptotic process. OS induced by cerebral ischemia triggers the JNK cascade (62). The anti- and pro-apoptotic protein markers, Bcl-2 and Bax, play an important role in the regulation of the apoptotic cell death pathway at the mitochondrial level (63,64). Another factor leading to apoptotic neurodegeneration is the Caspase cascade, especially C-3, which is the main effector of the apoptosis cascade leading to neurodegeneration (65). Muhammad et al. showed that the expression of JNK/Bax and cleaved Caspase-3 increased significantly in LPS-treated mice, while the level of anti-apoptotic Bcl-2 decreased markedly (55). Hesperetin also promoted Bcl-2 protein levels and prevents LPS-induced neuron apoptosis by reducing the expression of JNK/Bax and Caspase-3 (Figure 3).
Anti-atherosclerosis
Intracranial atherosclerosis (ICAS) is a common cause of ischemic stroke. The study of Chinese intracranial atherosclerosis (CICAS) has confirmed that ICAS is the most common vascular disease in Chinese patients with cerebrovascular disease, which is closely related to the high risk of stroke recurrence. The risk factors of ICAS mainly include age, race, hypertension, hyperlipidemia, metabolic syndrome, smoking, etc., which are also risk factors for ischemic stroke. Although ICAS is the main vascular disease in patients with ischemic stroke in Asia, 20–30% of Asian patients with ischemic stroke are caused by extracranial atherosclerosis (ECAS) (66).
Atherosclerosis and its resulting cerebrovascular disease seriously endanger the lives and health of people in China and around the world. After diffuse atherosclerosis of the cerebral artery, the lumen is narrowed, which reduces the blood supply to the brain parenchyma and causes ischemic changes in the brain. Macrophages play an important role in the mechanism of atherosclerosis. Hesperetin can enhance the expression of macrophage lipid transporter adenosine triphosphate binding transporter A1, inhibit macrophage infiltration in the plaque site, and reduce foam cell formation in the plaque area. Wang et al. (67) labeled CD68 macrophages by immunofluorescence staining, which showed that hesperetin can significantly reduce macrophage infiltration into aortic sinus plaques and foam cell formation, and inhibit the expression and secretion of tumor necrosis factor-α (TNF-α) and monocyte chemoattractant protein-1 (MCP-1) inflammatory factors in apoE-/mice, thereby reducing or delaying the progression of atherosclerosis and inhibiting the inflammatory reaction (Figure 4). Hesperetin also exerts an anti-atherosclerotic effect by inhibiting the proliferation and migration of smooth muscle cells. Jin et al. (27) studied the effect of hesperetin on the proliferation of primary cultured rat aortic vascular smooth muscle cells (VSMCs) induced by platelet-derived growth factor (PDGF)-bb. Their results illustrated that hesperetin inhibited PDGF-bb-induced proliferation of rat aortic VSMCs through G(0)/G(1) blockade (Figure 4).
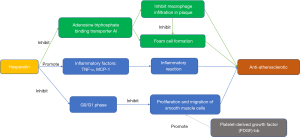
Anti-inflammatory reaction
The inflammatory reaction is an important cause of continuous brain tissue injury following cerebral ischemia, especially in cerebral ischemia-reperfusion. Therefore, controlling a series of cascade inflammatory reactions after cerebral ischemia is an important strategy to reduce cerebral ischemia-reperfusion injury (68). After cerebral ischemia, microglia and astrocytes are activated rapidly, and the activated and proliferated microglia and astrocytes release a large number of pro-inflammatory mediators and neurotoxic molecules, which participate in the cerebral ischemia-induced inflammatory reaction. These inflammatory mediators can in turn further stimulate the activation and proliferation of microglia and astrocytes, thereby aggravating the inflammatory reaction and forming a vicious circle.
Nuclear factor-kappaB (NF-κB) is a multidirectional transcription factor that regulates several other genes involved in the inflammatory reaction and stimulates a variety of cell signaling pathways, leading to increased production of inflammatory cell factors. Thus, the inhibition of NF-κB-mediated cellular and molecular processes may provide a potential target for neuroinflammatory-related neurological diseases. LPS treatment leads to the activation of microglia and increased astrocytes, and also increases the expression of inflammatory mediators, such as phosphorylation nuclear factor κB (p-NF-κB), TNF-α, and interleukin-1β (IL-1β), which are involved in various kinds of psychogenic diseases including cerebral ischemia (69-70). The findings of Muhammad et al. demonstrated that hesperetin significantly reduces the expression level of phosphorylated-nuclear factor-kappaB (p-NF-κB) in LPS-stimulated mice brains, and markedly reduces the expression levels of TNF-α and IL-1β cytokines (55). The effect of hesperetin on neuroinflammation is notably mediated by the inhibition of NF-κB signaling (Figure 5).
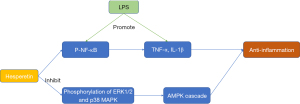
Mitogen activated protein kinases (MAPKs) are important upstream regulators of inflammatory cytokines in various biological systems. MAPKs, including the extracellular signal-regulated kinase 1/2 (ERK1/2), JNK, and p38 MAPK, are involved in the activation of BV-2 microglia cells stimulated by LPS. Jo et al. reported that hesperetin can significantly inhibit the phosphorylation of ERK1/2 and p38 MAPK in LPS-stimulated BV-2 microglia (Figure 5) (9).
Anti-thrombosis
After a cerebral ischemic attack, the blood remains in a hypercoagulable state, which is conducive to the formation of platelet thrombi and is directly involved in the occurrence and development of repeated cerebral ischemia (71). By constructing an middle cerebral artery occlusion (MCAO) model, Okada et al. confirmed that platelets are activated after focal cerebral ischemia, and platelet activation plays a key role in the natural course of the disease (72). Thrombosis is one of the important factors in secondary brain injury. Therefore, inhibiting platelet aggregation and subsequent thrombosis can play a protective role in brain injury following ischemic episodes.
According to Jin et al., hesperetin can inhibit platelet aggregation induced by collagen and arachidonic acid, and the cellular mechanism of hesperetin’s antiplatelet activity is mainly mediated by inhibition of phospholipase C-2 (PLC-2) phosphorylation and cyclooxygenase-1 activity (27). This beneficial characteristic of hesperetin is critical in thrombosis (Figure 6).
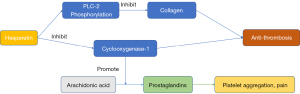
Anti-hyperlipidemia
Hyperlipidemia is diagnosed in patients with total cholesterol over 220 mg/dL, triglycerides over 160 mg/dL, high-density lipoprotein (HDL) below 40 mg/dL, and low-density lipoprotein (LDL) above 160 mg/dL, or those with a history of using lipid-lowering drugs. In a clinical study of the risk factors for stroke recurrence, Kang et al. confirmed that hyperlipidemia is correlated with the recurrence of ischemic stroke within 5 years, especially in cases of large atherosclerotic stroke, which may be attributable to the more severe baseline atherosclerotic stenosis in such patients (73). Also, Zhao et al. showed that patients with atherosclerotic dyslipidemia have a higher risk of recurrence of ischemic stroke (74). Atherosclerotic dyslipidemia is defined as the decrease of HDL and the increase of triglycerides. Lee et al. reported that elevated triglyceride levels and decreased HDL levels in a fasting state increase the risk of coronary heart disease and ischemic stroke in diabetes patients (75). Therefore, high cholesterol, high triglycerides, decreased HDL, increased LDL, and a history of treatment with lipid-lowering drugs are all risk factors for ischemic stroke.
The experimental study reported by Choi et al. showed that hesperetin could inhibit the activity of cholesterol-producing and esterifying enzymes, thereby achieving an anti-hyperlipidemia effect (76). 3-hydroxy-3-methylglutaryl-coenzyme A (HMG CoA) reductase and acyl coenzyme cholesterol acyltransferase (ACAT) are the main rate-limiting enzymes for cholesterol synthesis and esterification in animals. An experiment using high-fat model mice showed that the synthesis and esterification of cholesterol are affected by hesperetin and its metabolites, which is represented by reducing the activities of HMG-CoA reductase and ACAT enzymes. The carrier protein is the protein part of lipoproteins, which are involved in the transport of cholesterol and triglycerides in the body. Hesperetin reduces the bioavailability of assembled lipids for carrier protein-bound lipoproteins, thereby improving the transport capacity of cholesterol in the blood. Hesperetin reduces blood lipid by reducing the transcription of ACAT-2 messenger ribonucleic acid (mRNA) in Hep (hepatocyte) G2 cells and reducing the synthesis carrier protein. The above effects are achieved in three ways: (I) reducing the activity of ATAC1 and ATAC2; (II) selectively reducing the expression of ATAC2; and (III) reducing the activity of the triglyceride transfer protein (Figure 7).
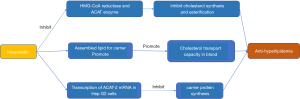
Anti-nitric oxide damage
Research results in recent years have shown that NO, a nitrogen oxide compound with free radicals, uses arginine as a substrate and produces the dual effects of neuroprotection and neurotoxin catalyzed by nitric oxide synthase (NOS). There are three isoforms of the NOS isoenzyme, namely, nNOS, iNOS, and eNOS. The NO that is produced is neurotoxic, which is generally due to the overexpression of iNOS and nNOS, while the NO produced by eNOS has a neuroprotective effect. Previous studies have shown that nNOS plays a key role in the process of ischemia and initiates the primary effects in the early phase of excitotoxic injury. Firstly, iNOS protein and catalytic activity can be detected 12 hours after cerebral ischemia. Next, the iNOS inhibitor can reduce the infarct area in the focal cerebral infarction model 24 hours after cerebral ischemia. Finally, iNOS reaches a peak within 48 hours after cerebral ischemia and recovers to the basic level after 7 days. Experimental results have demonstrated that endothelial NOS has a neuroprotective effect, and NO mediated by neurogenic NOS participates in brain injury in the early stage of ischemia and has a neurotoxic effect (77,78). NO has the dual functions of neuroprotection and neurotoxicity (Figure 8) (9). All the mechanisms of hesperetin in the treatment of cerebral ischemia were summarized in Table 2. Such a comprehension of the recent progress of hesperetin will help understand the progress of the effect of hesperetin intervention on the evolution of cerebral ischemia.
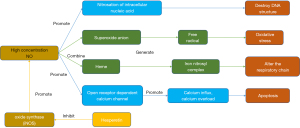
Table 2
Effect | Mechanism | References |
---|---|---|
Anti-oxidative stress and apoptosis | Inhibit ROS, LPO, and JNK/Bax, caspase-3 | (46-50) |
Promote Nrf2, HO-1, and Bcl-2 | ||
Anti-atherosclerosis | Inhibit TNF-α, MCP-1, and the G0/G1 phase | (56) |
Promote adenosine triphosphate binding transporter A1 | ||
Anti-inflammatory reaction | Inhibit P-NF-κB, ERK1/2, and p38 MAPK | (58-60) |
Anti-thrombosis | Inhibit PCL-2, phosphorylation, and cyclooxygenase-1 | (24) |
Anti-hyperlipidemia | Inhibit HMG-CoA reductase and ACAT enzymes | (65) |
Assembled lipid for carrier protein binding | ||
Transcription of ACAT-2 mRNA | ||
Anti-nitric oxide damage | Inhibit iNOS | (66,68) |
ROS, reactive oxygen species; LPO, lipid peroxidation; JNK, c-Jun N-terminal kinase; BAX, Bcl-2 associated X protein; Nrf-2, nuclear factor erythroid 2-related factor 2; HO-1, heme oxygenase-1; Bcl-2, B cell lymphoma-2; TNF-α, tumor necrosis factor-α; MCP-1, monocyte chemoattractant protein-1; p-NF-κB, phosphorylated -nuclear factor-kappaB; ERK1/2, extracellular signal-regulated kinase 1/2; p38 MAPK, p38 mitogen-activated protein kinase; PLC-2, phospholipase C-2; HMG CoA, 3-hydroxy-3-methylglutaryl-coenzyme A; ACAT, acyl coenzyme cholesterol acyltransferase; iNOS, inducible nitric oxide synthase.
Conclusions and future prospects
In summary, hesperetin are widely involved in various regulatory pathways and play an important role, such as anti-oxidative stress and apoptosis, anti-atherosclerosis, anti-inflammatory reaction, anti-thrombosis, anti-hyperlipidemia, anti-nitric oxide damage. The mechanism of hesperetin in the treatment of cerebral ischemia was summarized in Table 2. At present, there are few studies on hesperetin in the treatment of cerebral ischemia, and most of these are experiments that use nerve cells to construct a certain model of cerebral ischemia in vitro, excluding a few in vivo studies. More research is required to further explore this topic. However, hesperetin exhibits numerous pharmacological effects that can be modulated to treat cerebral ischemia, and thus, its overall pathogenic mechanism of action is worth studying. At the same time, a study has shown that hesperetin inhibits Ca2+ overload in cardiomyocytes (79). We believe that hesperetin may also have the same effect on brain cells.
The advantages of hesperetin are its abundance, large output, low production cost, accurate biological activity, and minimal toxic side effects. China is a large citrus-producing country, with a citrus output of 41.318 million tons in 2018, and therefore has extremely rich hesperetin resources. As a key component of citrus fruits, hesperetin has broad development prospects. The main disadvantage of hesperetin is that despite its many pharmacological effects, its clinical application is greatly limited due to its poor water solubility and low biological activity. To improve the biological activity and enhance the therapeutic role of hesperetin, researchers have used synthetic derivatives of hesperetin, prepared through the application of bionanotechnology pharmaceutical preparations, to markedly improve its bioavailability in recent years. Collectively, these features indicate the considerable research significance of hesperetin.
Currently, the clinical application of hesperidin includes the treatment of paroxysmal tachycardia, the rescue of septic shock, and chronic gastritis (80). There are presently no reports on the treatment of cerebral ischemia using hesperetin, and the application of hesperetin in cerebral ischemia requires further study. The pharmacological mechanism of hesperetin has not been fully elucidated; however, with further research on its mechanism of action and the development of related derivatives, the potential pharmacological effects and value of hesperetin will be further explored, which will contribute to the development and synthesis of more efficient hesperetin analogues or novel drugs.
Acknowledgments
Funding: This work was supported by the Medical Scientific Research Foundation of Guangdong Province, China (No. B2021398) and the Maoming Science and Technology Project (No. 2020KJZX021).
Footnote
Reporting Checklist: The authors have completed the Narrative Review reporting checklist. Available at https://atm.amegroups.com/article/view/10.21037/atm-22-3136/rc
Conflicts of Interest: All authors have completed the ICMJE uniform disclosure form (available at https://atm.amegroups.com/article/view/10.21037/atm-22-3136/coif). The authors have no conflicts of interest to declare.
Ethical Statement: The authors are accountable for all aspects of the work in ensuring that questions related to the accuracy or integrity of any part of the work are appropriately investigated and resolved.
Open Access Statement: This is an Open Access article distributed in accordance with the Creative Commons Attribution-NonCommercial-NoDerivs 4.0 International License (CC BY-NC-ND 4.0), which permits the non-commercial replication and distribution of the article with the strict proviso that no changes or edits are made and the original work is properly cited (including links to both the formal publication through the relevant DOI and the license). See: https://creativecommons.org/licenses/by-nc-nd/4.0/.
References
- Yang G, Wang Y, Zeng Y, et al. Rapid health transition in China, 1990-2010: findings from the Global Burden of Disease Study 2010. Lancet 2013;381:1987-2015. [Crossref] [PubMed]
- Zhou M, Wang H, Zeng X, et al. Mortality, morbidity, and risk factors in China and its provinces, 1990-2017: a systematic analysis for the Global Burden of Disease Study 2017. Lancet 2019;394:1145-58. [Crossref] [PubMed]
- Zhou M, Wang H, Zhu J, et al. Cause-specific mortality for 240 causes in China during 1990-2013: a systematic subnational analysis for the Global Burden of Disease Study 2013. Lancet 2016;387:251-72. [Crossref] [PubMed]
- Xie YP, Li YJ. Research progress on the risk factors of ischemic stroke Chinese Journal of Integrative Medicine on Cardio-Cerebrovascular Disease 2019;17:1493-5.
- Turley KR, Toledo-Pereyra LH, Kothari RU. Molecular mechanisms in the pathogenesis and treatment of acute ischemic stroke. J Invest Surg 2005;18:207-18. [Crossref] [PubMed]
- Liang Z, Zhang W, Zhu T, et al. Ischemia-Reperfusion Injury in Peripheral Artery Disease and Traditional Chinese Medicine Treatment. Evid Based Complement Alternat Med. 2021;2021:4954070. [Crossref] [PubMed]
- Tong HM. The medicinal value and application of citrus hesperetin Gansu Agricultural Science and Technology 2011;38-40.
- Ikram M, Muhammad T, Rehman SU, et al. Hesperetin Confers Neuroprotection by Regulating Nrf2/TLR4/NF-κB Signaling in an Aβ Mouse Model. Mol Neurobiol 2019;56:6293-309. [Crossref] [PubMed]
- Jo SH, Kim ME, Cho JH, et al. Hesperetin inhibits neuroinflammation on microglia by suppressing inflammatory cytokines and MAPK pathways. Arch Pharm Res 2019;42:695-703. [Crossref] [PubMed]
- Kim HJ, Jeon SM, Lee MK, et al. Comparison of hesperetin and its metabolites for cholesterol-lowering and antioxidative efficacy in hypercholesterolemic hamsters. J Med Food 2010;13:808-14. [Crossref] [PubMed]
- Liu HM, Liu W, Liang NC. Effect of Hesperetin Monosulfate Sodium Salt on Thrombosis and Platelet Aggregation Lishizhen Medicine and Materia Medica Research 2011;22:1898-9.
- Parhiz H, Roohbakhsh A, Soltani F, et al. Antioxidant and anti-inflammatory properties of the citrus flavonoids hesperidin and hesperetin: an updated review of their molecular mechanisms and experimental models. Phytother Res 2015;29:323-31. [Crossref] [PubMed]
- Ameer B, Weintraub RA, Johnson JV, et al. Flavanone absorption after naringin, hesperidin, and citrus administration. Clin Pharmacol Ther 1996;60:34-40. [Crossref] [PubMed]
- Erlund I, Meririnne E, Alfthan G, et al. Plasma kinetics and urinary excretion of the flavanones naringenin and hesperetin in humans after ingestion of orange juice and grapefruit juice. J Nutr 2001;131:235-41. [Crossref] [PubMed]
- Khan A, Ikram M, Hahm JR, et al. Antioxidant and Anti-Inflammatory Effects of Citrus Flavonoid Hesperetin: Special Focus on Neurological Disorders. Antioxidants (Basel) 2020;9:609. [Crossref] [PubMed]
- Ferreira de Oliveira JMP, Santos C, Fernandes E. Therapeutic potential of hesperidin and its aglycone hesperetin: Cell cycle regulation and apoptosis induction in cancer models. Phytomedicine 2020;73:152887. [Crossref] [PubMed]
- Iranshahi M, Rezaee R, Parhiz H, et al. Protective effects of flavonoids against microbes and toxins: The cases of hesperidin and hesperetin. Life Sci 2015;137:125-32. [Crossref] [PubMed]
- Fan J, Li Y, Fu X, et al. Nonhuman primate models of focal cerebral ischemia. Neural Regen Res 2017;12:321-8. [Crossref] [PubMed]
- Goyal M, Menon BK, van Zwam WH, et al. Endovascular thrombectomy after large-vessel ischaemic stroke: a meta-analysis of individual patient data from five randomised trials. Lancet 2016;387:1723-31. [Crossref] [PubMed]
- Hankey GJ. Thrombolysis for acute ischaemic stroke. J Clin Neurosci 2001;8:103-5. [Crossref] [PubMed]
- Röther J, Ford GA, Thijs VN. Thrombolytics in acute ischaemic stroke: historical perspective and future opportunities. Cerebrovasc Dis 2013;35:313-9. [Crossref] [PubMed]
- Chen XN, Zhu JB, Wang YN, et al. Research progress on pathogenesis of ischemic stroke and traditional Chinese medicine commonly used for treatment of ischemic stroke China Journal of Chinese Materia Medica 2019;44:20-30. [PubMed]
- Kanaze FI, Bounartzi MI, Georgarakis M, et al. Pharmacokinetics of the citrus flavanone aglycones hesperetin and naringenin after single oral administration in human subjects. Eur J Clin Nutr 2007;61:472-7. [Crossref] [PubMed]
- Deng QS, Su LQ. Synthesis and Properties Study of Hesperetin Molecular Imprinted Polymer Chemical Industry Times 2009;23:28-30.
- Chao TL, Wang QQ. New advances in research of pharmacological effects of hesperetin and its derivatives Chinese Traditional and Herbal Drugs 2018;625:259-64.
- Choi EJ. Antioxidative effects of hesperetin against 7,12-dimethylbenz(a)anthracene-induced oxidative stress in mice. Life Sci 2008;82:1059-64. [Crossref] [PubMed]
- Jin YR, Han XH, Zhang YH, et al. Hesperetin, a bioflavonoid, inhibits rat aortic vascular smooth muscle cells proliferation by arresting cell cycle. J Cell Biochem 2008;104:1-14. [Crossref] [PubMed]
- Li F, Chow S, Cheung WH, et al. The citrus flavonone hesperetin prevents letrozole-induced bone loss in a mouse model of breast cancer. J Nutr Biochem 2013;24:1112-6. [Crossref] [PubMed]
- Orallo F, Alvarez E, Basaran H, et al. Comparative study of the vasorelaxant activity, superoxide-scavenging ability and cyclic nucleotide phosphodiesterase-inhibitory effects of hesperetin and hesperidin. Naunyn Schmiedebergs Arch Pharmacol 2004;370:452-63. [Crossref] [PubMed]
- Chen X, Li XF, Chen Y, et al. Hesperetin derivative attenuates CCl4-induced hepatic fibrosis and inflammation by Gli-1-dependent mechanisms. Int Immunopharmacol 2019;76:105838. [Crossref] [PubMed]
- Jayaraman R, Subramani S, Sheik Abdullah SH, et al. Antihyperglycemic effect of hesperetin, a citrus flavonoid, extenuates hyperglycemia and exploring the potential role in antioxidant and antihyperlipidemic in streptozotocin-induced diabetic rats. Biomed Pharmacother 2018;97:98-106. [Crossref] [PubMed]
- Kiasalari Z, Khalili M, Baluchnejadmojarad T, et al. Protective Effect of Oral Hesperetin Against Unilateral Striatal 6-Hydroxydopamine Damage in the Rat. Neurochem Res 2016;41:1065-72. [Crossref] [PubMed]
- Luo W, Wang T, Hong C, et al. Design, synthesis and evaluation of 4-dimethylamine flavonoid derivatives as potential multifunctional anti-Alzheimer agents. Eur J Med Chem 2016;122:17-26. [Crossref] [PubMed]
- Vander Dos Santos R, Villalta-Romero F, Stanisic D, et al. Citrus bioflavonoid, hesperetin, as inhibitor of two thrombin-like snake venom serine proteases isolated from Crotalus simus. Toxicon 2018;143:36-43. [Crossref] [PubMed]
- Wang HW, Shi L, Xu YP, et al. Hesperetin alleviates renal interstitial fibrosis by inhibiting tubular epithelial-mesenchymal transition in vivo and in vitro. Exp Ther Med 2017;14:3713-9. [Crossref] [PubMed]
- Wang DD, Gao D, Xu WJ, et al. Magnetic molecularly imprinted polymer for the selective extraction of hesperetin from the dried pericarp of Citrus reticulata Blanco. Talanta 2018;184:307-15. [Crossref] [PubMed]
- Velusamy P, Mohan T, Ravi DB, et al. Targeting the Nrf2/ARE Signalling Pathway to Mitigate Isoproterenol-Induced Cardiac Hypertrophy: Plausible Role of Hesperetin in Redox Homeostasis. Oxid Med Cell Longev 2020;2020:9568278. [Crossref] [PubMed]
- Wang DD, Gao D, Huang YK, et al. Preparation of restricted access molecularly imprinted polymers based fiber for selective solid-phase microextraction of hesperetin and its metabolites in vivo. Talanta 2019;202:392-401. [Crossref] [PubMed]
- Zheng Y, Zhang YL, Li Z, et al. Design and synthesis of 7-O-1,2,3-triazole hesperetin derivatives to relieve inflammation of acute liver injury in mice. Eur J Med Chem 2021;213:113162. [Crossref] [PubMed]
- Actis-Goretta L, Dew TP, Lévèques A, et al. Gastrointestinal absorption and metabolism of hesperetin-7-O-rutinoside and hesperetin-7-O-glucoside in healthy humans. Mol Nutr Food Res 2015;59:1651-62. [Crossref] [PubMed]
- Liu H, Dong Y, Gao Y, et al. Hesperetin suppresses RANKL-induced osteoclastogenesis and ameliorates lipopolysaccharide-induced bone loss. J Cell Physiol 2019;234:11009-22. [Crossref] [PubMed]
- Menezes PD, Frank LA, Lima BD, et al. Hesperetin-loaded lipid-core nanocapsules in polyamide: a new textile formulation for topical drug delivery. Int J Nanomedicine 2017;12:2069-79. [Crossref] [PubMed]
- Roohbakhsh A, Parhiz H, Soltani F, et al. Molecular mechanisms behind the biological effects of hesperidin and hesperetin for the prevention of cancer and cardiovascular diseases. Life Sci. 2015;124:64-74. [Crossref] [PubMed]
- Wei L, Deng W, Cheng Z, et al. Effects of hesperetin on platelet-derived growth factor-BB-induced pulmonary artery smooth muscle cell proliferation. Mol Med Rep 2016;13:955-60. [Crossref] [PubMed]
- Ding HW, Huang AL, Zhang YL, et al. Design, synthesis and biological evaluation of hesperetin derivatives as potent anti-inflammatory agent. Fitoterapia 2017;121:212-22. [Crossref] [PubMed]
- Wang QQ, Shi JB, Chen C, et al. Hesperetin derivatives: Synthesis and anti-inflammatory activity. Bioorg Med Chem Lett 2016;26:1460-5. [Crossref] [PubMed]
- Mary Lazer L, Sadhasivam B, Palaniyandi K, et al. Chitosan-based nano-formulation enhances the anticancer efficacy of hesperetin. Int J Biol Macromol 2018;107:1988-98. [Crossref] [PubMed]
- Paniker NV, Srivastava SK, Beutler E. Glutathione metabolism of the red cells. Effect of glutathione reductase deficiency on the stimulation of hexose monophosphate shunt under oxidative stress. Biochim Biophys Acta 1970;215:456-60. [Crossref] [PubMed]
- Frantseva MV, Carlen PL, Perez Velazquez JL. Dynamics of intracellular calcium and free radical production during ischemia in pyramidal neurons. Free Radic Biol Med 2001;31:1216-27. [Crossref] [PubMed]
- Yrjänheikki J, Koistinaho J, Copin JC, et al. Spreading depression-induced expression of c-fos and cyclooxygenase-2 in transgenic mice that overexpress human copper/zinc-superoxide dismutase. J Neurotrauma 2000;17:713-8. [Crossref] [PubMed]
- He M, Zhao L, Wei MJ, et al. Neuroprotective effects of (-)-epigallocatechin-3-gallate on aging mice induced by D-galactose. Biol Pharm Bull 2009;32:55-60. [Crossref] [PubMed]
- Ishii T, Itoh K, Takahashi S, et al. Transcription factor Nrf2 coordinately regulates a group of oxidative stress-inducible genes in macrophages. J Biol Chem 2000;275:16023-9. [Crossref] [PubMed]
- Liu Z, Zhou T, Ziegler AC, et al. Oxidative Stress in Neurodegenerative Diseases: From Molecular Mechanisms to Clinical Applications. Oxid Med Cell Longev 2017;2017:2525967. [Crossref] [PubMed]
- Nuzzo D, Amato A, Picone P, et al. A Natural Dietary Supplement with a Combination of Nutrients Prevents Neurodegeneration Induced by a High Fat Diet in Mice. Nutrients 2018;10:1130. [Crossref] [PubMed]
- Muhammad T, Ikram M, Ullah R, et al. Hesperetin, a Citrus Flavonoid, Attenuates LPS-Induced Neuroinflammation, Apoptosis and Memory Impairments by Modulating TLR4/NF-κB Signaling. Nutrients 2019;11:648. [Crossref]
- Dong YJ, Gao WJ. The role and relationship of bcl-2, bax and caspase-3 in cell apoptosis. Chinese Journal of Gerontology 2012;32:4828-30.
- Cao G, Minami M, Pei W, et al. Intracellular Bax translocation after transient cerebral ischemia: implications for a role of the mitochondrial apoptotic signaling pathway in ischemic neuronal death. J Cereb Blood Flow Metab 2001;21:321-33. [Crossref] [PubMed]
- Degterev A, Lugovskoy A, Cardone M, et al. Identification of small-molecule inhibitors of interaction between the BH3 domain and Bcl-xL. Nat Cell Biol 2001;3:173-82. [Crossref] [PubMed]
- Van Opdenbosch N, Lamkanfi M. Caspases in cell death, inflammation, and disease. Immunity 2019;50:1352-64. [Crossref] [PubMed]
- Liang K, Ye Y, Wang Y, et al. Formononetin mediates neuroprotection against cerebral ischemia/reperfusion in rats via downregulation of the Bax/Bcl-2 ratio and upregulation PI3K/Akt signaling pathway. J Neurol Sci 2014;344:100-4. [Crossref] [PubMed]
- Liu ZR, Huo DS, Jia JX, et al. Protective effect and its mechanism of 5- (4-hydroxy-3-methoxybenzylidene) rhodanine on cerebral ischemia-reperfusion injury Chinese Journal of Comparative Medicine 2019;29:43-8.
- Li J, Xu B, Chen Z, et al. PI3K/AKT/JNK/p38 signalling pathway-mediated neural apoptosis in the prefrontal cortex of mice is involved in the antidepressant-like effect of pioglitazone. Clin Exp Pharmacol Physiol 2018;45:525-35. [Crossref] [PubMed]
- Chao DT, Korsmeyer SJ. Bcl-2 family: regulators of cell death. Annu Rev Immunol 1998;16:395-419. [Crossref] [PubMed]
- Sang Eun H, Seong Min K, Ho Jeong L, et al. Scutellarein Induces Fas-Mediated Extrinsic Apoptosis and G2/M Cell Cycle Arrest in Hep3B Hepatocellular Carcinoma Cells. Nutrients 2019;11:263. [Crossref] [PubMed]
- Louneva N, Cohen JW, Han LY, et al. Caspase-3 is enriched in postsynaptic densities and increased in Alzheimer's disease. Am J Pathol 2008;173:1488-95. [Crossref] [PubMed]
- Mas-Capdevila A, Teichenne J, Domenech-Coca C, et al. Effect of Hesperidin on Cardiovascular Disease Risk Factors: The Role of Intestinal Microbiota on Hesperidin Bioavailability. Nutrients 2020;12:1488. [Crossref] [PubMed]
- Wang F, Zhao C, Yang M, et al. Four Citrus Flavanones Exert Atherosclerosis Alleviation Effects in ApoE-/- Mice via Different Metabolic and Signaling Pathways. J Agric Food Chem 2021;69:5226-37. [Crossref] [PubMed]
- Huang YN, Ho YJ, Lai CC, et al. 1, 25-Dihydroxyvitamin D3 attenuates endotoxin- induced production of inflammatory mediators by inhibiting MAPK activation in primary cortical neuron-glia cultures. J Neuroinflammation 2015;12:147. [Crossref] [PubMed]
- Gao HJ, Bai HH, Lei T, et al. Protective effect of ligustrazine on cerebral ischemia reperfusion injury Chinese Journal of Gerontology 2015;35:5019-23.
- Taki-Nakano N, Kotera J, Ohta H. 12-oxo-phytodienoic acid, a plant-derived oxylipin, attenuates lipopolysaccharide-induced inflammation in microglia. Biochem Biophys Res Commun 2016;473:1288-94. [Crossref] [PubMed]
- Siesjö BK, Katsura K, Zhao Q, et al. Mechanisms of secondary brain damage in global and focal ischemia: a speculative synthesis. J Neurotrauma 1995;12:943-56. [Crossref] [PubMed]
- Okada Y, Copeland BR, Mori E, et al. P-selectin and intercellular adhesion molecule-1 expression after focal brain ischemia and reperfusion. Stroke 1994;25:202-11. [Crossref] [PubMed]
- Kang K, Wang Y, Wu J, et al. Association Between Cumulative Exposure to Increased Low-Density Lipoprotein Cholesterol and the Prevalence of Asymptomatic Intracranial Atherosclerotic Stenosis. Front Neurol 2020;11:555274. [Crossref] [PubMed]
- Zhao L, Wang R, Song B, et al. Association between atherogenic dyslipidemia and recurrent stroke risk in patients with different subtypes of ischemic stroke. Int J Stroke 2015;10:752-8. [Crossref] [PubMed]
- Lee JS, Chang PY, Zhang Y, et al. Triglyceride and HDL-C Dyslipidemia and Risks of Coronary Heart Disease and Ischemic Stroke by Glycemic Dysregulation Status: The Strong Heart Study. Diabetes Care 2017;40:529-37. [Crossref] [PubMed]
- Choi GS, Lee S, Jeong TS, et al. Evaluation of hesperetin 7-O-lauryl ether as lipid-lowering agent in high-cholesterol-fed rats. Bioorg Med Chem 2004;12:3599-605. [Crossref] [PubMed]
- Jung JE, Kim GS, Chen H, et al. Reperfusion and neurovascular dysfunction in stroke: from basic mechanisms to potential strategies for neuroprotection. Mol Neurobiol 2010;41:172-9. [Crossref] [PubMed]
- Zhao ZQ. Postconditioning in reperfusion injury: a status report. Cardiovasc Drugs Ther 2010;24:265-79. [Crossref] [PubMed]
- Zeng XY, Zhang NN, Ji YN, et al. Hesperetin attenuates hypoxia/reoxygenation-induced apoptosis in H9c2 cells via inhibition of calcium overload Chinese Journal of Pathophysiology 2019;35:113-20.
- Wen JJ. The latest pharmacological research and clinical verification of tangerine peel Guangming Journal of Chinese Medicine 2007;35:63-4.
(English Language Editor: A. Kassem)