Bone marrow is the preferred source for isolation of rat neutrophils and the subsequent acquisition of neutrophil extracellular traps
Introduction
Neutrophils are characterized by granules that contain a wide range of proteases which play a vital role in the innate immune response. Neutrophils prevent microbial invasion via strategies such as phagocytosis and degranulation (1). In 2004, Brinkmann et al. first described the secretion of neutrophil extracellular traps (NETs) as an anti-microbial mechanism which could restrain the dissemination of pathogenic bacteria (2). Subsequently, NETs have been shown to not only participate in the acute innate response, but also contribute to the pathogenesis, persistence, and progression of many chronic inflammatory diseases (3-7). The construction of NETs is realized by the double stranded (ds)DNA skeleton scaffolding of cytosolic and granule proteins, resulting in toxicity to invading microorganisms and native organic cells (2,5,8). Indeed, NETs can directly induce the death of epithelial and endothelial cells (8). Vaso-occlusion is another mechanism by which NETs can deteriorate the perfusion and reperfusion of important organs, resulting in thrombogenesis and impaired revascularization (9-11).
Recently, we reported the pathogenic role of NETs in uncontrolled fibrosis after myocardium infarction and during ventricular aneurysm formation, through perivascular fibrosis expansion (12). The myocardium infarction model and the isolation of neutrophils from bone marrow in mice are both technically mature and widely accepted in the literature (13,14). To isolate mouse neutrophils, the femur and tibia are harvested and the bone marrow is then gently flushed out with culture medium, followed by density gradient centrifugation. Once the neutrophils are isolated, they are incubated with phorbol 12-myristate 13-acetate (PMA) for 4 h to induce NETosis and the secretion of NETs (15,16). After the incubation, micrococcal nuclease (MNase) or deoxyribonuclease (DNase) are added for 30 min to obtain smaller NET fragments for improved cellular activity, or completely degraded NETs as negative controls. In humans, since there is a greater volume of blood compared to mice, polymorphonuclear (PMN) leukocytes are a suitable source for the isolation of human neutrophils (16), negating the need for bone marrow acquisition. This, together with a simplified protocol for isolating polymorphonuclear granulocytes using Polymorphprep (ProteoGenix, Schiltigheim, France), makes the whole procedure less time and energy consuming.
Recently we discovered NETs a role in atrial fibrillation related to cardiac remodeling. However, mouse models of atrial fibrillation could not be constructed unless certain regular channels or signaling pathways are knocked-down or knocked-out. This may be partly due to its atrium being too small to form a re-entrant cycle and further induce atrial fibrillation (17-20). Therefore, atrial fibrillation models in other animals such as rats, dogs, and pigs are preferable (20). Although atrial fibrillation can be induced in rats, our research demonstrated that rat neutrophils isolated from peripheral blood appeared to be flawed compared to its mouse counterparts. Despite sufficient blood quantities of 10 mL per rat, the percentage and amount of neutrophils isolated from rat peripheral blood remains extremely low (21). After several unsatisfactory attempts involving different methods to isolate neutrophils from rat peripheral blood, we examined the feasibility of the bone marrow-oriented strategy. This current article describes the isolation of neutrophils from bone marrow in detail, compares the NETs serection capability between rat peripheral and bone-marrow neutrophils, and presents some unique features of rat neutrophils during NETosis. Two different methods were developed for different experimental objectives. Both methods could ensure the quantity of obtained rat bone marrow neutrophils with less rats consumed, while two-step method are along with better purification of neutrophils. Additionally, we further compared the NETosis and NET formation of rat bone-marrow neutrophil to its peripheral counterpart, verifying its equal potency with PMN. We present the following article in accordance with the ARRIVE reporting checklist (available at https://atm.amegroups.com/article/view/10.21037/atm-22-2890/rc).
Methods
Animals and ethical statement
Specific pathogen-free Sprague Dawley (SD) rats, weighing 250 g, were purchased from Dashuo Biomedical Technique Company (Chengdu, Sichuan, China). The animal experiments were performed under a project license (No. 20211404A) granted by Animal Ethics Committee of West China Hospital, Sichuan University, in compliance with the guidelines of Animal Ethics Committee of West China Hospital, Sichuan University for the care and use of animals. The protocol was prepared before the study without registration.
Isolation of neutrophils
The protocol for the isolation of neutrophils from rat bone marrow is depicted in Figure 1, and consists of a one-step method and a two-step method, depending on the number of density gradient centrifugations. Commonly, two to three rats were used to isolate rat bone marrow deprived neutrophils. The femur and tibia of rats were detached from the connected muscle and other tissues and washed in Hank’s Balanced Salt Solution (HBSS) three times. To protect the femur head, the lower limbs were severed at the hip. The remaining irrelevant tissue was further trimmed with a small pair of scissors and bones were washed again in HBSS three times. After appendages were removed, a 5- or 10-mL syringe with needle was used to poke the marrow to loosen the marrow matrix. Another syringe was then used to rinse the bone marrow with Roswell Park Memorial Institute (RPMI) media. Lysis of red blood cells (RBCs) was performed to remove RBC contamination. The two-step method incorporated two density gradient centrifugations using different regents. The first isolation used a commercialized rat neutrophil isolation kit (P9200, Solarbio, China) and the second centrifugation was performed with percoll gradients of 55%, 65%, 70%, and 80%. Finally, the 70% percoll, including the cell layers at the boundaries, were collected and washed with HBSS for further use. By contrast, in the one-step method, bone marrow cells were only subjected to percoll gradients without the use of the rat neutrophil isolation kit.
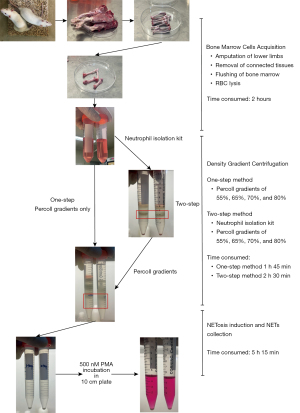
Acqusition of NETs
A total of 0.5×108–1×108 isolated neutrophils were resuspended in 4 mL RMPI media (supplemented with 10% fetal bovine serum (FBS) and 1% penicillin-streptomycin) in 10 cm plates and incubated with 500 nM PMA (Sigma, USA) for 3 hours to activate NETosis. For the negative control, DNase I (10 U/mL; Sigma, USA) was used to degrade the NETs. To harvest the NETs, the media was removed, and the NETs attached to the plate were washed gently with HBSS, followed by intense flushing with fresh media to detach the NETs from the plate. The washing medium was then collected and pipetted frequently for complete resuspension. The suspension was then centrifugated at 300 ×g for 10 min to remove any floating cells. Finally, the suspension containing the NETs was stored at −20 ℃ and used within 2 weeks.
Immunostaining and microscopy
Cells were fixed with 4% paraformaldehyde for 15 min and the NETs were fixed for 30 min to 1 h. Samples were then permeabilized with 0.5% Triton-X-100 (Biofroxx, German) for 10 min and sealed with the corresponding serum (Solarbio, China). Cells were then incubated with the primary antibodies anti-rat neutrophil elastase (Abcam, England, ab21595) and anti-rat myeloperoxidase (Abcam, England, ab208670, ab134132) overnight at 4 ℃, followed by the secondary antibody A488-conjugated donkey anti-rabbit IgG(H + L) (Invitrogen, USA, A32790), A594-conjugated donkey anti-mouse IgG(H + L) (Invitrogen, USA, A32744), and A594-conjugated goat anti-Mouse IgG1 (Invitrogen, USA, A21125) at room temperature for 1 h in the dark. All antibodies were diluted to the working concentration recommended by the manufacturer. Cell nuclei and NET skeletons were then stained with 10 mg/mL DAPI.
Quantification of NETs
To determine the concentration of NETs, the Picogreen dsDNA Assay Kit (Invitrogen, USA, P11496) was used in accordance with the manufacturer’s protocols. Briefly, the Tris-EDTA (TE) buffer and Picogreen regents were prepared with 19-fold volume of double distilled water or 199-fold volume of TE buffer, respectively. After preparing the standards (1 ng/mL, 10 ng/mL, 100 ng/mL, and 1 µg/mL), each 50 µL sample was added to 450 µL TE buffer. Subsequently, 100 µL Picogreen regent of was added to each well of a 96-well plate, followed by the same volume of prepared standards or diluted samples. After all samples were loaded, the plate was incubated at room temperature for 2–5 min, avoiding direct light. Samples were then read using a fluorescence microplate reader with emission spectrum around 530 nm and absorbance spectrum around 480 nm.
Analysis of NETs secretion by Celigo cytometry
The Celigo Image Cytometer instrument has been applied in high-throughput cell-based assays (22-24). In brief, a transmission and epifluorescence optical setup for one bright-field (BF) and four fluorescence (FL) imaging channels (blue, green, red, and far red) were included and optimized for analysis. The fluorescence filter was set for the corresponding colors as follows: blue (EX: 377/50 nm, EM: 470/22 nm), green (EX: 483/32 nm, EM: 536/40 nm), red (EX: 531/40 nm, EM: 629/53 nm), and far red (EX: 628/40 nm, EM: 688/31 nm). Auto and manual focus were provided, with the focus found in BF. After the focus was registered, highly uniform images of the entire plates (including 6-well, 12-well, 24-well, and 96-well plates) could be captured rapidly at different channels.
Before imaging, Hoechst (33342, Thermofisher, USA) and SytoxGreen (S7020, Thermofisher, USA) were added to reach final concentrations of 10 ng/mL and 300 nM, respectively, and incubated for 30 and 10 min, respectively. The fluorescent dyes were mixed by gentle pipetting. There was no need to discard the supernatant and wash the pellet. Sample plates were then scanned. The parameters for focus and exposure time for different channels were set, and the plates were scanned and images taken. Usually, if the Hoechst counts for different groups are similar, the NETs secretion could be determined by the SytoxGreen count. However, when embedded neutrophils varied sharply among different wells, ratio like SytoxGreen % or (Hoechst count – Hoechst-only count)/Hoechst count as Hoechst count indicates all cells and Hoechst-only count refers to living cells was recommended to detect the rate of neutrophils undergoing NETosis.
Statistical analysis
Results are presented as mean ± standard deviation. Groups were compared by analysis of variance and the Student’s t-test. All statistical analyses were performed using SPSS and Prism 7 software. P values less than 0.05 were considered statistically significant.
Results
Amount, purity, and viability of isolated neutrophils
Neutrophils were isolated from rat peripheral blood as previously described (21,25,26). About 10 mL peripheral blood could be obtained from the right ventricle of each rat. However, despite the extensive volume of peripheral blood, only 2×105–5×105 neutrophils could be isolated per rat (Figure 2A). Therefore, approximately 10–25 rats (5×106 neutrophils in total) would be required at each time point to acquire sufficient NETs (27). Additionally, the method involving both the isolation kit and the percoll gradients resulted in a great loss of neutrophils, while the dextran and Ficollpaque strategy resulted in too many red blood cell fragments that would interfere with the Celigo analysis (Figure 2B).
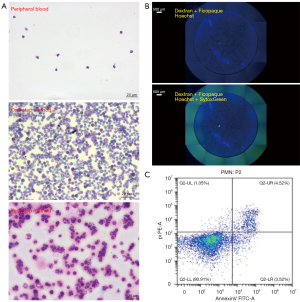
The isolation of neutrophils from rat bone marrow was adopted as a less consuming method. Taking into consideration both neutrophil density and lymphocyte contamination, a percoll gradient set including 55%, 65% 70%, and 80% was adopted, which was similar, but not identical that used in the peripheral blood method. Approximately 0.5×108–1×108 neutrophils could be obtained per rat, as demonstrated in Table 1. A comparison of the two methods revealed that the most evident differences were observed in purity and duration (Table 1). Using the one-step method, more than 75% of isolated cells were neutrophils, while the purity of the two-step method was greater than 90% (Figure 2A). However, compared to the one-step method, the two-step method required an additional 2–3 h on top of the 10–12 h required for the one-step method. Interestingly, although the density of neutrophils (1.0814–1.0919, with a peak at 1.0919) is significantly different from that of lymphocytes (1.0337–1.0765, with a peak at 1.0526), lymphocyte contamination appeared to be inevitable when isolating neutrophils from bone marrow, which may be partly due to the abundance of lymphocytes in the bone marrow and the increased density of immature lymphocytes (28). Flow cytometry analysis with the annexin V-FITC/PI apoptosis detection kit demonstrated that the viability of the isolated cells was above 90% (Figure 2C), which was comparable to its mouse and human counterparts (data not shown).
Table 1
Method | Rats required for each experiment | Purity (%) | Viability (%) | Total number of isolated cells (×108) | Time required (h) | Applicable conditions |
---|---|---|---|---|---|---|
One-step | 2 | >75 | >90 | 0.5–1 | 9–11 | NETs required for analysis of NET effect on other cells |
Two-step | 2–3 | >90 | >90 | 0.5–1 | 10–13 | Analysis of inducive capability of different stimulants for NETosis |
NET, neutrophil extracellular trap.
Features of rat NETosis
Previously, Saito et al. described a method to isolate rat neutrophils from bone marrow and reported that bone marrow neutrophils with increased F-actin formation are stiffer than those isolated from peripheral blood. However, the latter study did not analyze and compare the NET secretion properties of bone marrow neutrophils and peripheral neutrophils (29). Considering the heterogeneity shift between peripheral blood and bone marrow neutrophils, we first analyzed whether different reactiveness exists between them under different circumstances (30,31). To examine the NETosis process, rat neutrophils isolated from peripheral blood and bone marrow were incubated with PMA. A similar trend of NETs secretion was observed in both peripheral neutrophils and bone marrow neutrophils (Figure 3A). Interestingly, spontaneous NETosis was common in both rat peripheral neutrophils and bone marrow neutrophils, even in the absence of any stimulants, as demonstrated by the high protein levels of citrullinated H3 both before and after PMA incubation (data not shown). Overall, the properties of NET secretion were comparable between neutrophils isolated from rat bone marrow and those isolated from peripheral blood.
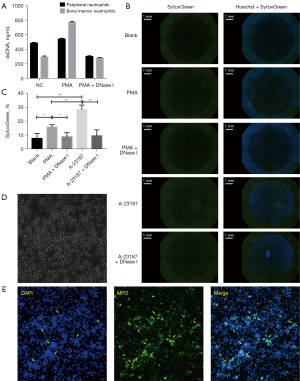
To further investigate the process of rat NETosis, PMA or calcimycin (A-23187) were used to induce NETosis. The detection of NETs was measured by cell-free DNA (cfDNA) staining with SytoxGreen and whole view scanning with Celigo. Incubation with PMA revealed that, in contrast to mouse and human neutrophils, rat neutrophils are more vulnerable to spontaneous NETosis, even in the absence of any stimulation, as shown by the 10% increased cfDNA content after 4 hours (Figure 3B). Additionally, despite the significant increase of cfDNA after PMA activation, NETs secreted by rat neutrophils are almost cloudy rather than spiky and showed weak cross-linking ability to form greater web-like structures. Compared to PMA, both cfDNA secretion and web-like structures were more significant when neutrophils were incubated with calcimycin. Despite this, PMA was used in the current study as it is widely accepted in the literature (Figure 3B,3C).
NETosis is a process involving DNA decondensation, autophagy, and intracellular matrix extrusion. In humans, the extrusion tends to be complete and only the remnants of the cell membrane remain. In contrast, much intracellular contents could be observed with rat neutrophils (Figure 3D). In addition, although neutrophils are cells in a suspension, the adhering capacity of neutrophils undergoing NETosis is enhanced. Therefore, from the macro perspective, NETs can form a film that sticks to the culture plate. While thick and well-structured human NETs tend to form a pasty, wax-like film, rat NET films have the appearance of gauze (data not shown).
Quantity of rat NETs
To obtain NETs, 0.5×108–1×108 neutrophils were incubated with 500 nM PMA. The final concentration of NET-DNA was 8–12 µg/mL. In some cases, MNase, as a restrictive endonuclease, was used to digest NETs into smaller, albeit more reactive, fragments. However, as mentioned above, the rat NETs had weak cross-link capability, and thus, mechanical disruption was sufficient to segment the rat NETs into smaller, active fragments (Figure 3E).
To acquire NETs for further incubation with other cells, the one-step method was less time and energy consuming, and is recommended as contaminating lymphocytes had no substantial impact on NET secretion and could be removed during the final centrifugation. On the contrary, to isolate neutrophils for subsequent NETosis and NET secretion experiments, the two-step method is recommended.
Discussion
Different strategies to isolate rat neutrophils from peripheral blood have been extensively reported with use of percoll, dextran or Ficollpaque (21,25,26). These methods were developed from isolation method of human PMNs. However, the scenario of rat peripheral blood is significantly different from human as monocytes rather than neutrophils are the most nucleated cells in rat peripheral blood. From peripheral blood of one rat, only 2×105–5×105 neutrophils could be isolated. In order to acquire enough neutrophils, we established a percoll-based method to deprive neutrophils from rat bone marrow, and we also demonstrated that bone marrow neutrophils and peripheral blood neutrophils are of equally capability in secreting NETs under different circumstances.
NETosis is the specialized cell death of neutrophils charactered by the secretion of NETs, which involves DNA de-condensation, autophagy, and intracellular matrix extrusion. As the neutrophil populations are different among different species, tissues or physiological states, neutrophils of different sources or subpopulations may be distinct in releasing NETs (32). And different from human, rat NETosis has several unique characteristics. Firstly, the extrusion of intracellular contents is incomplete in rat neutrophils. After incubation with PMA for 4 hours, much intracellular contents could be still observed within rat neutrophils. On the contrary in human neutrophil the extrusion tends to be complete and only the remnants of the cell membrane remain. Secondly, rat NETs are cloudy rather than spiky and are weak in cross-linking ability to form greater web-like structures. Instead of interweaving an extensive network, rat NETs fuse and fill in the gaps between neutrophils aggregating neutrophils together. Possibly due to the decreased cross-link capability and loss of extensive networks formation, rat NETs are more gauze-like macroscopically, while human NETs form a thick and wax-like film. At last, spontaneous NETosis is common in rat neutrophils. Rat neutrophils are more vulnerable to NETosis, and spontaneous NETosis takes place even without stimulation, which could be proved by elevated cfDNA of cultured rat neutrophils compared to human and shrunken cfDNA gap between rat neutrophils incubated with PMA or not.
Besides bone marrow, neutrophils can be isolated from peripheral blood, spleen, bronchoalveolar lavage and peritoneal lavage. Compared with human neutrophils, it is very difficult to isolate mouse neutrophils from blood, as blood volume of mouse is low. And it is hard to isolate neutrophils enough for the following experiments. Under normal circumstances, the abdominal or thoracic cavity does not contain a large quantity of neutrophils, both need artificial creation of an inflammatory environment to increase the infiltrated neutrophils. However neutrophil isolated through this way is lack of purity and quite aged. Compared with peripheral blood, spleen, abdominal and thoracic cavity, the bone marrow is an accessible neutrophil pool. Whether infected or not, a lot of neutrophils can be isolated from bone marrow. It has been reported that the heterogeneity is more complex in bone marrow, however most bone marrow neutrophils are mature which indicates the similar potencies of neutrophils deprived from bone marrow and peripheral blood (33).
Acknowledgments
We are grateful to Hongying Chen, Yan Wang, Huifang Li, Xiangyi Ren, Li Fu, Cong Li, Beibei Liu, Jiangrong Deng, and Mengli Zhu from the Core Facilities, West China Hospital, Sichuan University, for their assistance and guidance.
Funding: This work was supported by the National Natural Science Foundation of China (Nos. 81900311, 82100336 and 81970345).
Footnote
Reporting Checklist: The authors have completed the ARRIVE reporting checklist Available at https://atm.amegroups.com/article/view/10.21037/atm-22-2890/rc
Data Sharing Statement: Available at https://atm.amegroups.com/article/view/10.21037/atm-22-2890/dss
Conflicts of Interest: All authors have completed the ICMJE uniform disclosure form (available at https://atm.amegroups.com/article/view/10.21037/atm-22-2890/coif). The authors have no conflicts of interest to declare.
Ethical Statement: The authors are accountable for all aspects of the work in ensuring that questions related to the accuracy or integrity of any part of the work are appropriately investigated and resolved. The animal experiments were performed under a project license (No. 20211404A) granted by Animal Ethics Committee of West China Hospital, Sichuan University, in compliance with the guidelines of Animal Ethics Committee of West China Hospital, Sichuan University for the care and use of animals.
Open Access Statement: This is an Open Access article distributed in accordance with the Creative Commons Attribution-NonCommercial-NoDerivs 4.0 International License (CC BY-NC-ND 4.0), which permits the non-commercial replication and distribution of the article with the strict proviso that no changes or edits are made and the original work is properly cited (including links to both the formal publication through the relevant DOI and the license). See: https://creativecommons.org/licenses/by-nc-nd/4.0/.
References
- Papayannopoulos V. Neutrophil extracellular traps in immunity and disease. Nat Rev Immunol 2018;18:134-47. [Crossref] [PubMed]
- Brinkmann V, Reichard U, Goosmann C, et al. Neutrophil extracellular traps kill bacteria. Science 2004;303:1532-5. [Crossref] [PubMed]
- Dicker AJ, Crichton ML, Pumphrey EG, et al. Neutrophil extracellular traps are associated with disease severity and microbiota diversity in patients with chronic obstructive pulmonary disease. J Allergy Clin Immunol 2018;141:117-27. [Crossref] [PubMed]
- Franck G, Mawson TL, Folco EJ, et al. Roles of PAD4 and NETosis in Experimental Atherosclerosis and Arterial Injury: Implications for Superficial Erosion. Circ Res 2018;123:33-42. [Crossref] [PubMed]
- Marin-Esteban V, Turbica I, Dufour G, et al. Afa/Dr diffusely adhering Escherichia coli strain C1845 induces neutrophil extracellular traps that kill bacteria and damage human enterocyte-like cells. Infect Immun 2012;80:1891-9. [Crossref] [PubMed]
- Huang H, Tohme S, Al-Khafaji AB, et al. Damage-associated molecular pattern-activated neutrophil extracellular trap exacerbates sterile inflammatory liver injury. Hepatology 2015;62:600-14. [Crossref] [PubMed]
- Jorch SK, Kubes P. An emerging role for neutrophil extracellular traps in noninfectious disease. Nat Med 2017;23:279-87. [Crossref] [PubMed]
- Saffarzadeh M, Juenemann C, Queisser MA, et al. Neutrophil extracellular traps directly induce epithelial and endothelial cell death: a predominant role of histones. PLoS One 2012;7:e32366. [Crossref] [PubMed]
- Sambrano GR, Huang W, Faruqi T, et al. Cathepsin G activates protease-activated receptor-4 in human platelets. J Biol Chem 2000;275:6819-23. [Crossref] [PubMed]
- Chen G, Zhang D, Fuchs TA, et al. Heme-induced neutrophil extracellular traps contribute to the pathogenesis of sickle cell disease. Blood 2014;123:3818-27. [Crossref] [PubMed]
- Kang L, Yu H, Yang X, et al. Neutrophil extracellular traps released by neutrophils impair revascularization and vascular remodeling after stroke. Nat Commun 2020;11:2488. [Crossref] [PubMed]
- He L, Liu R, Yue H, et al. NETs promote pathogenic cardiac fibrosis and participate in ventricular aneurysm formation after ischemia injury through the facilitation of perivascular fibrosis. Biochem Biophys Res Commun 2021;583:154-61. [Crossref] [PubMed]
- Ubags NDJ, Suratt BT. Isolation and Characterization of Mouse Neutrophils. Methods Mol Biol 2018;1809:45-57. [Crossref] [PubMed]
- Gao E, Lei YH, Shang X, et al. A novel and efficient model of coronary artery ligation and myocardial infarction in the mouse. Circ Res 2010;107:1445-53. [Crossref] [PubMed]
- Fuchs TA, Abed U, Goosmann C, et al. Novel cell death program leads to neutrophil extracellular traps. J Cell Biol 2007;176:231-41. [Crossref] [PubMed]
- Brinkmann V, Laube B, Abu Abed U, et al. Neutrophil extracellular traps: how to generate and visualize them. J Vis Exp 2010; [Crossref] [PubMed]
- Campbell HM, Quick AP, Abu-Taha I, et al. Loss of SPEG Inhibitory Phosphorylation of Ryanodine Receptor Type-2 Promotes Atrial Fibrillation. Circulation 2020;142:1159-72. [Crossref] [PubMed]
- Moreira LM, Takawale A, Hulsurkar M, et al. Paracrine signalling by cardiac calcitonin controls atrial fibrogenesis and arrhythmia. Nature 2020;587:460-5. [Crossref] [PubMed]
- Li N, Wang T, Wang W, et al. Inhibition of CaMKII phosphorylation of RyR2 prevents induction of atrial fibrillation in FKBP12.6 knockout mice. Circ Res 2012;110:465-70. [Crossref] [PubMed]
- Schuttler D, Bapat A, Kaab S, et al. Animal Models of Atrial Fibrillation. Circ Res 2020;127:91-110. [Crossref] [PubMed]
- Lindena J, Burkhardt H. Separation and chemiluminescence properties of human, canine and rat polymorphonuclear cells. J Immunol Methods 1988;115:141-7. [Crossref] [PubMed]
- Kessel S, Cribbes S, Dery O, et al. High-Throughput 3D Tumor Spheroid Screening Method for Cancer Drug Discovery Using Celigo Image Cytometry. SLAS Technol 2017;22:454-65. [Crossref] [PubMed]
- Chan LL, Smith T, Kumph KA, et al. A high-throughput AO/PI-based cell concentration and viability detection method using the Celigo image cytometry. Cytotechnology 2016;68:2015-25. [Crossref] [PubMed]
- Zhang H, Chan LL, Rice W, et al. Novel high-throughput cell-based hybridoma screening methodology using the Celigo Image Cytometer. J Immunol Methods 2017;447:23-30. [Crossref] [PubMed]
- Zhai TY, Cui BH, Zou L, et al. Expression and Role of the Calcium-Sensing Receptor in Rat Peripheral Blood Polymorphonuclear Neutrophils. Oxid Med Cell Longev 2017;2017:3869561. [Crossref] [PubMed]
- Szucs S, Varga C, Ember I, et al. The separation of the granulocytes from different rat strains. A comparative study. J Immunol Methods 1994;167:245-51. [Crossref] [PubMed]
- Najmeh S, Cools-Lartigue J, Giannias B, et al. Simplified Human Neutrophil Extracellular Traps (NETs) Isolation and Handling. J Vis Exp 2015; [Crossref] [PubMed]
- Freeman GE, Dalton CA, Brooks PM. A Nycodenz gradient method for the purification of neutrophils from the peripheral blood of rats. J Immunol Methods 1991;139:241-9. [Crossref] [PubMed]
- Saito H, Lai J, Rogers R, et al. Mechanical properties of rat bone marrow and circulating neutrophils and their responses to inflammatory mediators. Blood 2002;99:2207-13. [Crossref] [PubMed]
- Silvestre-Roig C, Hidalgo A, Soehnlein O. Neutrophil heterogeneity: implications for homeostasis and pathogenesis. Blood 2016;127:2173-81. [Crossref] [PubMed]
- Xie X, Shi Q, Wu P, et al. Single-cell transcriptome profiling reveals neutrophil heterogeneity in homeostasis and infection. Nat Immunol 2020;21:1119-33. [Crossref] [PubMed]
- Hidalgo A, Libby P, Soehnlein O, et al. Neutrophil extracellular traps: from physiology to pathology. Cardiovasc Res 2021; [Crossref] [PubMed]
- Evrard M, Kwok IWH, Chong SZ, et al. Developmental Analysis of Bone Marrow Neutrophils Reveals Populations Specialized in Expansion, Trafficking, and Effector Functions. Immunity 2018;48:364-79 e8. [Crossref] [PubMed]