Novel application of 3D printed microporous prosthesis to repair humeral nonunion with segmental bone defects: a case report
Introduction
Bone possesses a remarkable capacity for regeneration and remodeling. However, approximately 5–10% of fractures result in nonunion fractures, even with clinical intervention (1). After reduction and fixation, bone atrophy, delayed healing, or nonunion may occur in severely injured fractures (2). The rate of nonunion after different treatments for humeral shaft fracture ranges from 0.3% to 13% (3). Moreover, long-term bone nonunion can cause internal fixation failure, and the fracture area may leave a large segment of refractory bone defects.
Repairing bone defects has always presented a challenge in clinical practice. Currently available methods for treating humeral bone defects include bone graft, distraction osteogenesis (Ilizarov technique), Masquelet’s induced membrane technique, vascularized fibular graft, and titanium mesh (4-11). However, these methods have some disadvantages: (I) they usually require larger amounts of bone graft material for huge defect construction, which leads to pain in patients undergoing autogenous bone grafting; (II) they cannot achieve early mobilization and weight-bearing; (III) they are not patient-specific and may not be applied to atypia bone defects.
The application of 3D printed prostheses in the reconstruction of bone defects is expected to compensate for the drawbacks of traditional treatment (12). Our previous clinical trial has proved that 3D printed microporous prostheses are safe and effective for treating large metaphyseal segmental femoral bone defects (13). Herein, we presented a single case of a patient with a 9.5 cm humerus shaft bone defect treated with a 3D printed Ti6Al4V microporous prosthesis after internal fixation failure of a middle-inferior humerus fracture. We investigated how 3D printed prostheses can repair the abnormal humeral shaft defect and form effective mechanical stability. Because the biological force transmitted between the prosthesis and the bone stump is weaker than that in lower limbs, we further examined a biological fusion between the prosthesis and bone without bone grafting. We present the following case in accordance with the CARE reporting checklist (available at https://atm.amegroups.com/article/view/10.21037/atm-22-447/rc).
Case presentation
We have finished the registry for clinical trials in the United States National Library of Medicine (No. NCT03941028). The patient was recruited in the clinical trial approved by the Institutional Review Board (IRB) at our hospital. She was scheduled for the implantation of a 3D printed microporous prosthesis. All procedures performed in this study were in accordance with the ethical standards of the institutional and/or national research committee(s) and with the Helsinki Declaration (as revised in 2013). Written informed consent was obtained from the patient for publication of this case report and accompanying images. A copy of the written consent is available for review by the editorial office of this journal.
History
A 53-year-old female suffered a fall on her left upper limb and complained of an obvious swelling of the left arm accompanied by pain in February 2019 (Figure 1). After physical and radiography examination (Figure 1A,1B), she was diagnosed with a fracture of the left humeral shaft. No open soft tissue injury or vascular injury was found. The fracture was treated with open reduction and internal fixation. Two months after the surgery, radiography reexamination indicated humeral nonunion. Nine months postoperatively, no healing of the fracture was detected, and the patient was admitted to our hospital for further diagnosis and treatment.
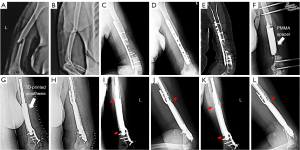
X-ray examination revealed a middle-inferior humeral nonunion with bone defects (Figure 1C,1D). An internal fixation image in position could be seen on the left humerus. A computerized tomography (CT) scan revealed that two screws at the distal end of the humerus were broken and that the middle part of the humerus lacked bone mass. In addition, osteosclerosis could be seen at the fractured end (Figure 1E).
First-stage surgery
The first-stage surgery involved withdrawing the broken internal fixation and debridement of all nonviable bone and interposed fibrous tissue. Two external fixation screws were placed into the humeral condyle, and two were implanted into the proximal end. The external fixed connecting rod was connected, and the polymethyl methacrylate (PMMA) cement spacer was planted in the bone defect areas and configured according to the original humeral bone shape (Figure 1F). Vacuum sealing drainage was used to cover the wound. The cement spacer was left in the defect areas for at least 6 weeks to ensure induced membrane formation and promote new bone regeneration. A 3D printed prosthesis design and virtual surgery were performed during this period.
Prosthesis design and fabrication
The customized patient-specific 3D printed Ti6Al4V implant and additional internal fixation were produced by Shandong Weigao Orthopaedic Device Company Limited. After debridement, the patient’s lesion area and the contralateral counterpart of the humerus were scanned by CT for further reconstruction planning. The manufacturer designed the product according to the contralateral mirror CT images. The surgeon was consulted for fixation requirements.
Materialise Mimics software was used to design the implant for functional, anatomical, and mechanical considerations. Prosthesis included a homogeneous porous structure with approximately 70% porosity, a pore size of (625±70) µm, an elastic modulus of (1,200±48) MPa, and compressive strength of (66±0.5) MPa (13). Since the defect area was 9.5 cm long and close to the humeral metaphysis, we designed the prosthesis as a “prosthesis-intramedullary nail-lateral plate” integrated implant (Figure 2). A schematic of the completed design dimensions was sent to the surgeon. At the same time, supplemental hardware was also designed, such as a trial implant and its replica. The implant was produced after the surgeon’s consent.
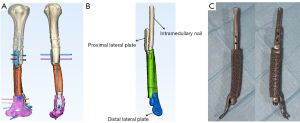
Second-stage surgery
Three months after the first-stage surgery, there was no local inflammation and no infection. The second-stage surgery was performed, including removal of external fixation and cement spacer as well as implantation of the designed 3D printed prosthesis. The original surgical incision was made, and the biceps and triceps were separated layer by layer to expose the cement spacer. After removing the cement spacer, the cicatricial tissue of the fracture end was debrided. Following reaming of the proximal ends, the microporous prosthesis was implanted into the induced membrane. The lateral plates were fixed with screws to promote the stability of the prosthesis. The process of the second-stage surgery is shown in Figure 3.
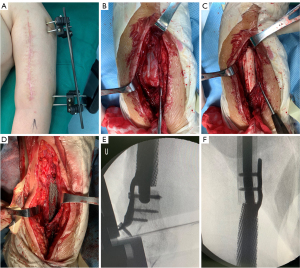
Outcomes and follow-up
In the early postoperative period, the patient was allowed partial weight training of the left upper limb. Physiotherapy, such as range of motion (ROM) exercises and full weight-bearing (2 months later), was performed for functional rehabilitation. Routine plain radiographs (Figure 1G-1L) and CT scans (Figure 4) were conducted to assess postoperative limb reconstruction and prosthesis incorporation. Calluses were found at the contact end of the prosthesis and bone at the 7-month follow-up. The callus was still growing around the surface of the prosthesis at the 18-month follow-up. At the latest follow-up, the upper limb functions were normal (Figure 5). The Mayo Elbow Performance Score (MEPS) was 85 points, and the Disabilities of the Arm, Shoulder, and Hand (DASH) score was 17.5 points. No complications were found.
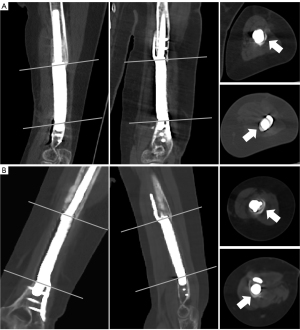
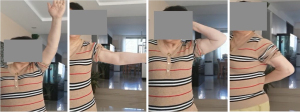
Discussion
Currently, there is no consensus on the optimal treatment for humeral shaft defects (3,14). The autologous or allogeneic bone graft was previously considered a gold standard for treating humeral reconstruction and functional restoration (4,5). Yet, when bone defects are severe, the traditional supply of bone graft material is insufficient. A vascularized fibular graft is another efficient treatment for large segmental bone defects (6,7). Nevertheless, it is a challenging technique associated with a high risk of insufficient vascularization and donor-site morbidity (15). Ilizarov’s external fixation method may also be applied to treat the bone defects in the humerus (8,9), but this technique is somehow complex with frequent surgeon participation during the treatment process and high costs. Additionally, wearing an external fixator for a long time may be uncomfortable for the patients.
In our case, a customized 3D printed prosthesis was applied to reconstruct the humeral shaft bone defect (9.5 cm in size). The major advantages of this method are personalized shape compatibility, sufficient mechanical strength, early mobilization and weight-bearing, and the prevention of bone donor-site morbidity. For large defects >5 cm, a bone graft is subject to resorption, and grating materials are limited (14). Our patient was 53 years old, and this age is often associated with osteoporosis, which puts the effectiveness of bone fusion to the test. Moreover, when developing bone reconstruction strategies, the alignment of the bone and the anatomic characteristics of the original limb must also be assessed. The patient’s bone defect was located in the middle and distal humerus. The middle part of the humerus is cylindrical, while the distal part is relatively broad and flat, which makes it harder to achieve anatomic morphological union of both ends of the bone defect. The 3D printed prosthetic can be customized to match the local anatomical morphology and biomechanical environment of the patient’s humerus.
Accurate reconstruction of bone defects through customized prostheses could achieve immediate stability and length recovery of limbs. Previous research has confirmed the intercalary prosthesis’s excellent ability to reconstruct humeral shaft bone defects through a biomechanical analysis (16). Zhang et al. (17) used an intercalary prosthesis to reconstruct humeral shaft defects following tumor resection, and the prosthesis was designed and manufactured without microporous structures and 3D printing technology. Moreover, Luenam et al. (18) used 3D printing technology to repair severe distal humerus fractures with complete loss of the lateral column; however, this was structural rather than segmental repair. Hence, we sought to introduce a more suitable individualized prosthesis aimed at the reconstruction of humeral defects in this case.
Our prosthesis was an all-in-one design fabricated as a “prosthesis-intramedullary nail-lateral plate” integrated structure implant. Considering the particularity of the humeral defect, for prosthesis design, we adopted the combination of the intramedullary nail and lateral plate at the proximal end. The intramedullary part was longer, which is conducive to stress dispersion. The distal part of the humerus was flat without much medullary cavity; thus, the distal end of the prosthesis slightly protruded into the medullary cavity. At the same time, the lateral plate was extended and fixed to the humeral condyle to provide the stability of local fixation. The integral design makes the structural connection and force conduction between the prosthesis and the fixations more stable and, at the same time, makes it more convenient for prosthesis implantation in surgery. Additionally, the microporous surface of the prosthesis added friction to stabilize the interface and promoted new bone trabeculae to grow into the implant. The compressive strength is significantly lower than that of cortical bone and close to that of cancellous bone, which is beneficial to reducing the local stress shielding effect after prosthesis implantation. Our animal experiment (12) revealed that new bone could grow into the micropores and with time, a stable implant-bone complex was formed without bone grafting. In this patient, at a 7-month follow-up, callus formation was found at the end of the prosthesis and bone stump. At the last follow-up, the proximal callus was enlarged and crawled around the surface of the prosthesis. The cortical thickness of the bone stump at the contact position between the prosthesis and bone showed that under the action of stress, the reactive growth of bone structure could adapt to the new mechanical environment. Relatively, the gap between the distal prosthesis and the remaining bone continuously narrowed during follow-up, suggesting osteogenesis at this site.
For this patient, Masquelet’s induced membrane technique was applied to our therapeutic strategy at the first stage for achieving osteointegration of the implant-bone. Previous studies reported that the Masquelet technique could be used to treat humeral nonunion with satisfying outcomes (11). Other studies found that the autologous foreign-body membrane created by the immune response to the cement spacer could promote vascularity and bone regeneration by secreting osteogenic growth factors for 4–8 weeks after spacer implantation (19,20). In this case, the induced membrane was wrapped around the prosthesis and could grow into the prosthesis in the subsequent growth process to form a tight connection. With new bone integrating into the micropores of the prosthesis, a stable biological anchor was formed.
Extracorporeal stimulation positively affects nonunion healing (2). When applying 3D printed prosthesis for reconstructing bone defects, it is not necessary to wait for bone healing before carrying out weight-bearing exercises for limbs. The Ti6Al4V prosthesis provided sufficient mechanical strength and force conduction for bones with stable fixation so that the upper limb could be restored to a biomechanical environment close to the natural state. On the second day after surgery, the patient began left upper limb mobility and weight-bearing exercises under the guidance of the rehabilitation department. Early and regular functional exercise may promote axial stress stimulation and micromotion at the implant-bone interface and thus be conducive to osseointegration. At the last follow-up, the patient had a normal range of elbow motion with a satisfactory MEPS score and DASH score. The prosthesis remained stable in the original implantation area without significant loosening, displacement, or fracture.
This case report has a few limitations. First, a longer follow-up duration is needed to assess possible complications after the implantation. Second, personalized customization of 3D printed prostheses is currently more reflected in morphology than internal structure. 3D printed prostheses that match the uneven biomechanical properties of the initial bone itself need to be further developed to restore the inherent biomechanical conduction of the limb. Finally, we only observed the bone ingrowth in the contact area between the bone stump and the prosthesis. Whether bone ingrowth occurs in the middle of the prosthesis needs to be assessed during long-term follow-up. Despite the aforementioned limitations, this case report provides a valuable direction for further research.
To summarize, reconstruction with a 3D printed microporous prosthesis is an effective option for humeral shaft defects. Compared to other treatments for bone defects, this novel method can achieve immediate stability and early mobilization. Without bone grafting, the bone structure can form biological stability between the bone stump and the prosthesis. Therefore, it might be used as an alternative to repair large segmental bone defects of limbs.
Acknowledgments
Funding: This work was supported by Beijing Municipal Science & Technology Commission (Project No. Z181100001718195).
Footnote
Reporting Checklist: The authors have completed the CARE reporting checklist. Available at https://atm.amegroups.com/article/view/10.21037/atm-22-447/rc
Conflicts of Interest: All authors have completed the ICMJE uniform disclosure form (available at https://atm.amegroups.com/article/view/10.21037/atm-22-447/coif). The authors have no conflicts of interest to declare.
Ethical Statement: The authors are accountable for all aspects of the work in ensuring that questions related to the accuracy or integrity of any part of the work are appropriately investigated and resolved. All procedures performed in this study were in accordance with the ethical standards of the institutional and/or national research committee(s) and with the Helsinki Declaration (as revised in 2013). Written informed consent was obtained from the patient for publication of this case report and accompanying images. A copy of the written consent is available for review by the editorial office of this journal.
Open Access Statement: This is an Open Access article distributed in accordance with the Creative Commons Attribution-NonCommercial-NoDerivs 4.0 International License (CC BY-NC-ND 4.0), which permits the non-commercial replication and distribution of the article with the strict proviso that no changes or edits are made and the original work is properly cited (including links to both the formal publication through the relevant DOI and the license). See: https://creativecommons.org/licenses/by-nc-nd/4.0/.
References
- Ball AN, Donahue SW, Wojda SJ, et al. The challenges of promoting osteogenesis in segmental bone defects and osteoporosis. J Orthop Res 2018;36:1559-72. [Crossref] [PubMed]
- Wildemann B, Ignatius A, Leung F, et al. Non-union bone fractures. Nat Rev Dis Primers 2021;7:57. [Crossref] [PubMed]
- Peters RM, Claessen FM, Doornberg JN, et al. Union rate after operative treatment of humeral shaft nonunion--A systematic review. Injury 2015;46:2314-24. [Crossref] [PubMed]
- De Carolis O, Mori CM, Vicenti G, et al. A lifelong story: Case report of a humeral shaft nonunion successfully treated after 30 years. Injury 2018;49:S43-7. [Crossref] [PubMed]
- Stevens NM, Schultz BJ, Lowe DT, et al. Repair of Humeral Shaft Nonunion With Plate and Screw Fixation and Iliac Crest Bone Graft. J Orthop Trauma 2021;35:S7-8. [Crossref] [PubMed]
- George PK, Dasgupta B, Reddy B, et al. A Case Report of Post-traumatic Osteomyelitis with Gap Non-union of Humerus with Segmental Bone Defect and Scarred Skin Treated with Open Reduction Internal Fixation with Osteomyocutaneous Fibula. J Orthop Case Rep 2021;11:52-4. [Crossref] [PubMed]
- Bigoni M, Turati M, Arnoldi M, et al. Distal humeral septic non-union treated with debridement and vascularized fibular transfer: case report and review of the literature. Eur Rev Med Pharmacol Sci 2019;23:12-8. [PubMed]
- Kiran M, Jee R. Ilizarov's method for treatment of nonunion of diaphyseal fractures of the humerus. Indian J Orthop 2010;44:444-7. [Crossref] [PubMed]
- Tomić S, Bumbasirević M, Lesić A, et al. Ilizarov frame fixation without bone graft for atrophic humeral shaft nonunion: 28 patients with a minimum 2-year follow-up. J Orthop Trauma 2007;21:549-56. [Crossref] [PubMed]
- Attias N, Thabet AM, Prabhakar G, et al. Management of extra-articular segmental defects in long bone using a titanium mesh cage as an adjunct to other methods of fixation: a multicentre report of 17 cases. Bone Joint J 2018;100-B:646-51. [Crossref] [PubMed]
- Gaillard J, Masquelet AC, Boutroux P, et al. Induced-membrane treatment of refractory humeral non-union with or without bone defect. Orthop Traumatol Surg Res 2020;106:803-11. [Crossref] [PubMed]
- Zhang T, Wei Q, Zhou H, et al. Three-dimensional-printed individualized porous implants: A new "implant-bone" interface fusion concept for large bone defect treatment. Bioact Mater 2021;6:3659-70. [Crossref] [PubMed]
- Hou G, Liu B, Tian Y, et al. An innovative strategy to treat large metaphyseal segmental femoral bone defect using customized design and 3D printed micro-porous prosthesis: a prospective clinical study. J Mater Sci Mater Med 2020;31:66. [Crossref] [PubMed]
- Mauffrey C, Barlow BT, Smith W. Management of segmental bone defects. J Am Acad Orthop Surg 2015;23:143-53. [PubMed]
- Bumbasirevic M, Stevanovic M, Bumbasirevic V, et al. Free vascularised fibular grafts in orthopaedics. Int Orthop 2014;38:1277-82. [Crossref] [PubMed]
- Zhao LM, Tian DM, Wei Y, et al. Biomechanical Analysis of a Novel Intercalary Prosthesis for Humeral Diaphyseal Segmental Defect Reconstruction. Orthop Surg 2018;10:23-31. [Crossref] [PubMed]
- Zhang JF, Wang F, Hu YC. Reconstruction of Humeral Shaft Defect with an Intercalary Endoprosthesis Following Resection of Tumor. Orthop Surg 2018;10:281-4. [Crossref] [PubMed]
- Luenam S, Kosiyatrakul A, Phakdeewisetkul K, et al. The patient-specific implant created with 3D printing technology in treatment of a severe open distal humerus fracture with complete loss of the lateral column. J Orthop Surg (Hong Kong) 2020;28:2309499020960251. [Crossref] [PubMed]
- Alford AI, Nicolaou D, Hake M, et al. Masquelet's induced membrane technique: Review of current concepts and future directions. J Orthop Res 2021;39:707-18. [Crossref] [PubMed]
- Klein C, Monet M, Barbier V, et al. The Masquelet technique: Current concepts, animal models, and perspectives. J Tissue Eng Regen Med 2020;14:1349-59. [Crossref] [PubMed]