TAK-242 ameliorates epileptic symptoms in mice by inhibiting the TLR4/NF-κB signaling pathway
Introduction
Epilepsy is a chronic disease in which sudden abnormal neuronal discharges in the brain lead to transient brain dysfunction. There are many clinical types of epilepsy (1), including temporal lobe epilepsy, idiopathic epilepsy, and refractory epilepsy. According to the World Health Organization’s statistics, in 2018, the total number of epilepsy patients in the world exceeded 70 million (2). New patients are especially likely to be children and teenagers (2). In addition to causing physical harm to patients, epilepsy places a great burden on patients’ families and societies. It is an urgent social problem that needs to be addressed.
The inflammatory reaction of brain tissue as a result of seizures is the main cause of pathological changes to brain tissue after seizures. Currently, there is increasing evidence that there is a mutually reinforcing role between the inflammatory response and seizures (3,4). Toll-like receptors (TLRs) are innate immune receptors that recognize pathogen-associated molecular patterns, which initiate related signal transduction pathways and play an important role in immune and inflammatory responses (5,6). Nuclear factor kappa beta (NF-κB) is located in the toll-like receptor 4 (TLR4) downstream signaling pathway and is considered an important initiating factor in the inflammatory cascade. Studies have shown that the expression of TLR4 is significantly upregulated in the brain tissue samples of epileptic patients and animals with chronic epilepsy (7-10). Shi et al. showed that the inhibition of TLR4/NF-κB ameliorated coriamyrtin-induced epilepsy (11). These results suggest that TLR4/NF-kB and its downstream activated tumor necrosis factor alpha (TNF-α) may be used as new targets for the treatment of epilepsy.
TAK-242 is a potent TLR4 inhibitor that selectively binds to TLR4 and blocks the interaction of TLR4 with adaptor molecules, thereby inhibiting TLR4 signaling and its downstream NF-κB/TNF-α signaling events (12). A study demonstrated that TAK-242 exerted neuroprotective effects by inhibiting the TLR4/MyD88/TRIF/NF-κB signaling pathway in a rat model of neonatal hypoxic-ischemic encephalopathy (13). However, no studies have examined the protective effect of TAK-242 on epilepsy in neurological disorders or its mechanism.
Thus, in this study, we hypothesized that TAK-242 inhibits and protects epilepsy models through the above-mentioned inflammatory pathways. We established a mouse epilepsy model via the intraperitoneal injection of pentetrazol (PTZ). Additionally, we intragastrically administered the mice with 3 mg/kg of TAK-242 to investigate the protective effect of TAK-242 treatment on epileptic mice and its possible mechanism. At present, the main research directions of epilepsy drugs are: ion channel drugs and neurotransmitter drugs. The number of patients with refractory epilepsy is increasing, and some patients cannot tolerate the side effects of long-term use of such antiepileptic drugs. Therefore, it is of certain research value to find other therapeutic approaches. The relationship between inflammatory pathways and epilepsy has been confirmed in recent years (14). It provides a new direction for the treatment of epilepsy. Our study, based on the anti-inflammatory activity of TAK-242, preliminarily demonstrated the reversal of epileptic symptoms by inhibiting the TLR4/NF-κB inflammatory pathway. The results of this study provide a theoretical basis for understanding the mechanism of epileptogenesis and developing new target drugs for the prevention and treatment of epilepsy. Figure 1 shows our study groups and methods. We present the following article in accordance with the ARRIVE reporting checklist (available at https://atm.amegroups.com/article/view/10.21037/atm-22-2707/rc).
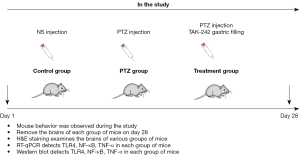
Methods
Grouping and administration
The study was approved by the ethics committee of North Sichuan Medical College (ethics No. 2021-67), and the experiments was conducted in compliance with the institutional guidelines for the care and use of animals. A protocol was prepared before the study without registration. A total of 24 4-week-old male C57 BL/6 mice (Beijing HFK Bio-Technology, China) were housed in an specific pathogen free (SPF) animal laboratory under diurnal cyclic light (12 h/12 h) at a temperature of (23±1) ℃. The mice weighed 18±1 g. We followed the principle of substituting, improving, or reducing (3R) the use of animals throughout the study.
The epilepsy model was established via intraperitoneally injecting the mice with PTZ. Using the random number table method, the mice were randomly divided into the control group, model (PTZ) group, and treatment [PTZ + TAK-242 (3 mg/kg)] group. Each group comprised 8 mice.
PTZ and TAK-242 were freshly prepared before each administration in physiological saline. The mice in the control group were intraperitoneally injected with normal saline (10 mL/kg) every other day for a total of 14 times. The mice in the model group were intraperitoneally injected with PTZ (37 mg/kg) for a total of 14 times. The mice in the treatment group were intraperitoneally injected with PTZ 37 mg/kg every other day and intragastrically administered with 3 mg/kg TAK-242 daily. The injection and gastric filling time ran from 9:00 to 11:00. After each injection, the injection site of the mice was sterilized with 75% ethanol.
After each intraperitoneal injection of PTZ, the behavior of the mice was observed for 30 minutes, and a seizure grade was recorded with reference to the Racine grading criteria. Under the Racine criteria (15), grade 0 indicates normal behavior and no abnormalities, grade 1 indicates facial spasms, including frequent whisker shaking and rhythmic mastication, grade 2 indicates nodding neck myoclonic manifestations, grade 3 indicates longitudinal twitching along the body, grade 4, indicates bilateral forelimb clonus, and grade 5 indicates generalized tonic-clonic “grand mal” seizures or death. The brain tissue of the mice was removed on day 28, and deep anesthesia was performed using chloral hydrate during tissue removal.
Detection of mRNA expression of TLR4, NF-κB, and TNF-α (RT-qPCR)
About 30 mg of mouse brain tissue was added to extract brain tissue RNA using Trizol. Reverse transcription was performed using a reverse transcription kit (abm; G492). Table 1 shows the real-time quantitative polymerase chain reaction (RT-qPCR) reaction system, Table 2 provides details of the RT-qPCR primers.
Table 1
Reagent | Volume |
---|---|
Maxima SYBR Green/ROX qPCR Master Mix (2×) | 10 μL |
Complementary deoxyribonucleic acid | 0.5 μL |
Forwards primer | 0.5 μL |
Backwards primer | 0.5 μL |
Water (ddH2O) | 8.5 μL |
Total volume | 20 μL |
RT-qPCR, real time quantitative polymerase chain reaction; ddH2O, double distilled H2O.
Table 2
Primer | Sequence |
---|---|
TLR4 forwards | 5'-ATGGCATGGCTTACACCACC-3' |
TLR4 backwards | 5'-GAGGCCAATTTTGTCTCCACA-3' |
NF-κB forwards | 5'-GCCAGACACAGATGATCGCC-3' |
NF-κB backwards | 5'-GTTTCGGGTAGGCACAGCAA-3' |
TNF-α forwards | 5'-ATGTCTCAGCCTCTTCTCATTC-3' |
TNF-α backwards | 5'-GCTTGTCACTCGAATTTTGAGA-3' |
Actin forwards | 5'-GTGCTATGTTGCTCTAGACTTCG-3' |
Actin backwards | 5'-ATGCCACAGGATTCCATACC-3' |
RT-qPCR, real time quantitative polymerase chain reaction; TLR4, toll-like receptor 4; NF-κB, nuclear factor kappa beta, TNF-α, tumor necrosis factor-α.
Detection of protein expression of TLR4, NF-kB, and TNF-α (Western blot)
Lysate was added to the mouse brain tissues, which were then lysed on ice for 2 h for BCA kit determination and adjustment (the total concentration of each histone was 50 µg/µL). The tissue protein samples were added to polyacrylamide gels [10% sodium dodecyl sulfate (SDS)] for electrophoresis, transfer membrane and primary antibody [diluted TLR4 (Abcam, USA, ab217274), NF-κB (CST, USA, 8242S), TNF-α (Abcam, USA, ab6671), or β-actin (Affinity, USA, AF7018) antibodies were added at a ratio of 1:1,000] was incubated overnight. After washing the membranes every other day, secondary antibody (Abcam, USA) was added. Diaminobenzidine (DAB) chromogenic solution was used for color development. A Bio-Pro gel-imaging analyzer was used to scan the bands for imaging. Quantity-one software was used to perform a gray-scale scanning analysis of each histone lane band to calculate the corresponding protein expression.
Hematoxylin and eosin (H&E) staining
The mice brain was harvested after being perfused paraformaldehyde through the heart and the mice brain was embedded in paraffin. The paraffin sections of mouse hippocampus were obtained by coronal section. After dewaxing and hydration, then the sections were stained with H&E. After the neutral resin was sealed, image information was acquired under a microscope.
Statistical methods
All the data results are presented as the mean ± standard error (mean ± SEM). The statistical analysis was performed using a 1-way analysis of variance, and the F-test was performed using SAS9.3 analysis software (SAS Institute Inc., Carry, NC, USA). A P value <0.05 indicated a statically significant difference.
Results
Epilepsy determination
Based on the criteria set out in Section Grouping and administration above, 7 mice in the model group had grade 3 and above symptoms, and 2 mice in the treatment group had grade 3 and above symptoms, with statistically significant difference (P<0.05; see Table 3 for further details).
Table 3
Group | Grade 0 | Grade 1 | Grade 2 | Grade 3 | Grade 4 | Grade 5 |
---|---|---|---|---|---|---|
Control group | 8 | 0 | 0 | 0 | 0 | 0 |
Model group | 0 | 0 | 1 | 2 | 3 | 2 |
Treatment group | 0 | 4 | 2 | 1 | 1 | 0 |
H&E staining results
As Figure 2 shows, the pyramidal cells and granulosa cells in the hippocampal area of the control mice were arranged in a regular, dense, normal shape, with uniform staining, a transparent cytoplasm, round nucleus, and no clear morphological abnormalities. Compared to the control group, the number of pyramidal cells and granulosa cell neurons in the model group was significantly reduced, and the staining was more intense. In the model group, the cytoplasm of the neurons was constricted, and the cell shape was irregular, mostly conical, and polygonal, and the nucleus was difficult to see. The number of surviving nerve cells in the treatment group was higher than that in the model group, and the cell morphology in the treatment group was nearly normal.
Detection of mRNA expression of TLR4, NF-κB, and TNF-α (RT-qPCR)
As Figure 3 shows, the messenger RNA (mRNA) expression levels of TLR4, NF-κB, and TNF-α in the hippocampus and brain-mantle tissue of the mice in the model group were significantly upregulated compared to those of mice in the control group (P<0.05); the mRNA expressions of TLR4, NF-κB, and TNF-α in the hippocampus and brain-mantle tissue of the mice in the treatment group were significantly decreased compared to those of mice in the model group (P<0.05).
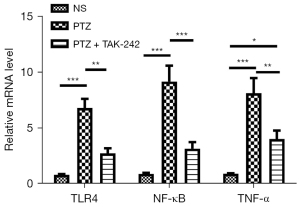
Detection of protein expression of TLR4, NF-κB, and TNF-α (Western blot)
As Figures 4,5 show, the protein expression of TLR4 and TNF-α in both the hippocampus and brain-mantle tissue was significantly upregulated (P<0.05), and the expression of NF-κB tended to be more upregulated in the model group than the control group. The protein expression of TLR4 in the hippocampus and brain-mantle tissue was significantly downregulated (P<0.05), and NF-κB and TNF-α expression tended to be more downregulated in the treatment group than the model group.
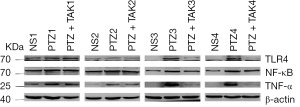
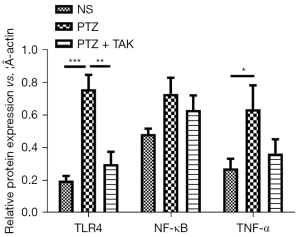
Statistical inclusion and side effects
We reviewed the literature and found that mice had a higher mortality rate during PTZ modeling. During the pre-experiment, we found that the main cause of death in the mice was airway obstruction caused by spasms in the laryngeal muscles. During the pre-experiment and formal experiment, when seizures were observed in mice with grade 3 and above symptoms, we opened the mouth of the mouse by pressing on the head of the mouse with a cotton swab to achieve the purpose of unclogging the respiratory tract; the operation lasted for 1 minute. Fortunately, after the above operations, most of the mice survived. Thus, in the formal experiments, no mouse died unexpectedly, and all the mice were included in the statistical analysis.
During the modeling process, the mice in the control group did not receive any other special treatments, as such treatments could have produced errors; thus, we only injected the control group with normal saline to reduce the generation of errors.
Discussion
The pathogenesis of epilepsy is complex and closely related to immune inflammation. Most experimental data and clinical evidence have shown that the expression of some specific inflammatory mediators, such as HMGB-1, NF-κB, TNF-α, and cognate receptors, such as TLR4, is increased in chronic epileptic brain tissue, a phenomenon called “neuroinflammation” (16,17). Experimental epileptic seizures can be inhibited by drug interventions directed at the related inflammatory signaling pathways.
As a pattern recognition receptor, TLR4 elicits the body’s innate immune response and induces an inflammatory response by recognizing and binding to the corresponding pathogen-associated molecular patterns. In neurological diseases, neuroinflammatory responses play an important role in brain tissue damage. TLR4 is widely expressed in the nervous system, and neurological diseases, such as cerebral hemorrhage, cerebral ischemia, and traumatic brain injury, all lead to the release of various endogenous ligands and the upregulation of TLR4 expression levels, which are then involved in the pathophysiological process of neurological diseases through signaling cascade transduction mechanisms (18).
It was found that neurotransmitters such as gamma-aminobutyric acid (GABA), Serotonin, Glutamate, Dopamine were changed in neuroinflammation induced by TLR4 (19-21), For example (22): GABA:LPS inhibits GABA receptor activity at postsynaptic sites by activating TLR4, releasing IL-1β, and by activating protein kinase C (PKC) in neurons. After TLR4 activation, LPS reduced GABAergic activities by impelling GABA synthesis at the presynaptic site.
Research has shown that TLR4 and its downstream NF-κB signaling pathway are strongly activated in epilepsy models (23-26). Shi et al. found that TLR4/NF-κB expression was significantly upregulated in the brain tissue of patients with intractable epilepsy, and the inhibition of its expression improved epileptogenesis (11). Yu et al. found that the TLR4/NF-κB signaling pathway was strongly activated in epilepsy models, and the inhibition of TLR4/NF-κB pathway activation had anticonvulsant and neuroprotective effects in a PTZ-induced acute epilepsy model in mice (23). Liu et al. found that the TLR4/NF-κB pathway was significantly activated in autoimmune encephalomyelitis (26). Additionally, research has shown that the TLR4 pathway is activated in immature rat models and pediatric temporal lobe epilepsy models (25). Consistent with these results, in this experiment, a mouse epilepsy model was established using PTZ, and we found that after modeling, the mice displayed generalized tonic convulsions and involuntary falling, and the expression of the TLR4/NF-κB and TNF-α gene and protein was significantly upregulated in the mouse brain tissue.
TAK-242 is a potent TLR4 inhibitor that selectively binds to TLR4 and blocks the interaction of TLR4 with adaptor molecules, thereby inhibiting TLR4 signaling and its downstream NF-κB/TNF-α signaling events (12). Research has shown that TAK-242 exerted neuroprotective effects by inhibiting the TLR4/MyD88/TRIF/NF-κB signaling pathway in a rat model of neonatal hypoxic-ischemic encephalopathy (13). Numerous studies have shown that the inhibition of the TLR4/NF-κB pathway has a protective effect on epilepsy (27-29). Liu et al. found that microRNA-129-5p inhibits the development of autoimmune encephalomyelitis-associated epilepsy by targeting HMGB1 through the TLR4/NF-κB signaling pathway (26). MiR-181b attenuated autophagy and apoptosis in rats with juvenile epilepsy induced by kainic acid by inhibiting the P38 the mitogen-activated protein kinase/c-Jun N-terminal kinase (p38/JNK) signaling pathway by targeting TLR4 (30). Epigallocatechin-3 gallate protects against lithium-pilocarpine epilepsy by inhibiting the TLR4/NF-κB signaling pathway (31). Rhein attenuates PTZ-induced epilepsy and exerts neuroprotective effects by inhibiting the TLR4/NF-κB signaling pathway (23). Consistent with the above results, in the present study, we used TAK-242 in a PTZ epileptic mice model and observed the key factors and histomorphological changes of the inflammatory pathways in each group of mice. We found that TAK-242 significantly inhibited the TLR4/NF-κB signaling pathway and significantly improved epileptic symptoms in mice due to its neuroprotective effects.
In summary, this study is the first to show that TAK-242 has a protective effect on epileptic symptoms in mice, and that this effect is associated with its inhibition of TLR4/NF-κB signaling pathway activation. Our results provide a rationale for understanding the mechanism of epileptogenesis and for the development of TAK-242 as a new target drug for the prevention and treatment of epilepsy.
Acknowledgments
We would like to acknowledge the Institute of Neurological Diseases of North Sichuan Medical College for providing experimental sites and equipment, and the teachers of the Institute of Neurological Diseases of Chuanbei Medical College for providing guidance on experimental ideas and methods.
Funding: This work was supported by funding from the National Natural Science Foundation of China (No. 81971220).
Footnote
Reporting Checklist: The authors have completed the ARRIVE reporting checklist. Available at https://atm.amegroups.com/article/view/10.21037/atm-22-2707/rc
Data Sharing Statement: Available at https://atm.amegroups.com/article/view/10.21037/atm-22-2707/dss
Conflicts of Interest: All authors have completed the ICMJE uniform disclosure form (available at https://atm.amegroups.com/article/view/10.21037/atm-22-2707/coif). The authors have no conflicts of interest to declare.
Ethical Statement: The authors are accountable for all aspects of the work in ensuring that questions related to the accuracy or integrity of any part of the work are appropriately investigated and resolved. The study was approved by the ethics committee of North Sichuan Medical College (ethics No. 2021-67), and the experiments was conducted in compliance with institutional guidelines for the care and use of animals.
Open Access Statement: This is an Open Access article distributed in accordance with the Creative Commons Attribution-NonCommercial-NoDerivs 4.0 International License (CC BY-NC-ND 4.0), which permits the non-commercial replication and distribution of the article with the strict proviso that no changes or edits are made and the original work is properly cited (including links to both the formal publication through the relevant DOI and the license). See: https://creativecommons.org/licenses/by-nc-nd/4.0/.
References
- Symonds JD, Zuberi SM, Johnson MR. Advances in epilepsy gene discovery and implications for epilepsy diagnosis and treatment. Curr Opin Neurol 2017;30:193-9. [Crossref] [PubMed]
- Guerrini R. Epilepsy in children. Lancet 2006;367:499-524. [Crossref] [PubMed]
- Vezzani A, Aronica E, Mazarati A, et al. Epilepsy and brain inflammation. Exp Neurol 2013;244:11-21. [Crossref] [PubMed]
- Terrone G, Balosso S, Pauletti A, et al. Inflammation and reactive oxygen species as disease modifiers in epilepsy. Neuropharmacology 2020;167:107742. [Crossref] [PubMed]
- Behzadi P, García-Perdomo HA, Karpiński TM. Toll-Like Receptors: General Molecular and Structural Biology. J Immunol Res 2021;2021:9914854. [Crossref] [PubMed]
- Lwin T, Yang JL, Ngampramuan S, et al. Melatonin ameliorates methamphetamine-induced cognitive impairments by inhibiting neuroinflammation via suppression of the TLR4/MyD88/NFκB signaling pathway in the mouse hippocampus. Prog Neuropsychopharmacol Biol Psychiatry 2021;111:110109. [Crossref] [PubMed]
- Walker A, Russmann V, Deeg CA, et al. Proteomic profiling of epileptogenesis in a rat model: Focus on inflammation. Brain Behav Immun 2016;53:138-58. [Crossref] [PubMed]
- Li H, Liu S, Han J, et al. Role of Toll-Like Receptors in Neuroimmune Diseases: Therapeutic Targets and Problems. Front Immunol 2021;12:777606. [Crossref] [PubMed]
- Matin N, Tabatabaie O, Falsaperla R, et al. Epilepsy and innate immune system: A possible immunogenic predisposition and related therapeutic implications. Hum Vaccin Immunother 2015;11:2021-9. [Crossref] [PubMed]
- Raetz CR, Whitfield C. Lipopolysaccharide endotoxins. Annu Rev Biochem 2002;71:635-700. [Crossref] [PubMed]
- Shi Y, Zhang L, Teng J, et al. HMGB1 mediates microglia activation via the TLR4/NF-κB pathway in coriaria lactone induced epilepsy. Mol Med Rep 2018;17:5125-31. [Crossref] [PubMed]
- Matsunaga N, Tsuchimori N, Matsumoto T, et al. TAK-242 (resatorvid), a small-molecule inhibitor of Toll-like receptor (TLR) 4 signaling, binds selectively to TLR4 and interferes with interactions between TLR4 and its adaptor molecules. Mol Pharmacol 2011;79:34-41. [Crossref] [PubMed]
- Jiang L, Xu Z, Li H, et al. TAK-242 exerts a neuroprotective effect via suppression of the TLR4/MyD88/TRIF/NF-κB signaling pathway in a neonatal hypoxic-ischemic encephalopathy rat model. Mol Med Rep 2020;22:1440-8. [Crossref] [PubMed]
- Rana A, Musto AE. The role of inflammation in the development of epilepsy. J Neuroinflammation 2018;15:144. [Crossref] [PubMed]
- Racine RJ, Steingart M, McIntyre DC. Development of kindling-prone and kindling-resistant rats: selective breeding and electrophysiological studies. Epilepsy Res 1999;35:183-95. [Crossref] [PubMed]
- Amhaoul H, Hamaide J, Bertoglio D, et al. Brain inflammation in a chronic epilepsy model: Evolving pattern of the translocator protein during epileptogenesis. Neurobiol Dis 2015;82:526-39. [Crossref] [PubMed]
- Kamaşak T, Dilber B, Yaman SÖ, et al. HMGB-1, TLR4, IL-1R1, TNF-α, and IL-1β: novel epilepsy markers? Epileptic Disord 2020;22:183-93. [Crossref] [PubMed]
- Deng YN, Zhou WB. Expression of TLR4 and TLR9 mRNA in Lewis rats with experimental allergic neuritis. Neuroimmunomodulation 2007;14:337-43. [Crossref] [PubMed]
- Campolo M, Paterniti I, Siracusa R, et al. TLR4 absence reduces neuroinflammation and inflammasome activation in Parkinson's diseases in vivo model. Brain Behav Immun 2019;76:236-47. [Crossref] [PubMed]
- Dionisie V, Filip GA, Manea MC, et al. The anti-inflammatory role of SSRI and SNRI in the treatment of depression: a review of human and rodent research studies. Inflammopharmacology 2021;29:75-90. [Crossref] [PubMed]
- Wang K, Liu Y, Shi Y, et al. Amomum tsaoko fruit extract exerts anticonvulsant effects through suppression of oxidative stress and neuroinflammation in a pentylenetetrazol kindling model of epilepsy in mice. Saudi J Biol Sci 2021;28:4247-54. [Crossref] [PubMed]
- Yan X, Jiang E, Weng HR. Activation of toll like receptor 4 attenuates GABA synthesis and postsynaptic GABA receptor activities in the spinal dorsal horn via releasing interleukin-1 beta. J Neuroinflammation 2015;12:222. [Crossref] [PubMed]
- Yu L, Yang J, Yu W, et al. Rhein attenuates PTZ-induced epilepsy and exerts neuroprotective activity via inhibition of the TLR4-NFκB signaling pathway. Neurosci Lett 2021;758:136002. [Crossref] [PubMed]
- von Rüden EL, Gualtieri F, Schönhoff K, et al. Molecular alterations of the TLR4-signaling cascade in canine epilepsy. BMC Vet Res 2020;16:18. [Crossref] [PubMed]
- Yang W, Li J, Shang Y, et al. HMGB1-TLR4 Axis Plays a Regulatory Role in the Pathogenesis of Mesial Temporal Lobe Epilepsy in Immature Rat Model and Children via the p38MAPK Signaling Pathway. Neurochem Res 2017;42:1179-90. [Crossref] [PubMed]
- Liu AH, Wu YT, Wang YP. MicroRNA-129-5p inhibits the development of autoimmune encephalomyelitis-related epilepsy by targeting HMGB1 through the TLR4/NF-kB signaling pathway. Brain Res Bull 2017;132:139-49. [Crossref] [PubMed]
- Iori V, Iyer AM, Ravizza T, et al. Blockade of the IL-1R1/TLR4 pathway mediates disease-modification therapeutic effects in a model of acquired epilepsy. Neurobiol Dis 2017;99:12-23. [Crossref] [PubMed]
- Li X, Lin J, Hua Y, et al. Agmatine Alleviates Epileptic Seizures and Hippocampal Neuronal Damage by Inhibiting Gasdermin D-Mediated Pyroptosis. Front Pharmacol 2021;12:627557. [Crossref] [PubMed]
- Paudel YN, Khan SU, Othman I, et al. Naturally Occurring HMGB1 Inhibitor, Glycyrrhizin, Modulates Chronic Seizures-Induced Memory Dysfunction in Zebrafish Model. ACS Chem Neurosci 2021;12:3288-302. [Crossref] [PubMed]
- Wang L, Song LF, Chen XY, et al. MiR-181b inhibits P38/JNK signaling pathway to attenuate autophagy and apoptosis in juvenile rats with kainic acid-induced epilepsy via targeting TLR4. CNS Neurosci Ther 2019;25:112-22. [Crossref] [PubMed]
- Qu Z, Jia L, Xie T, et al. (-)-Epigallocatechin-3-Gallate Protects Against Lithium-Pilocarpine-Induced Epilepsy by Inhibiting the Toll-Like Receptor 4 (TLR4)/Nuclear Factor-κB (NF-κB) Signaling Pathway. Med Sci Monit 2019;25:1749-58. [Crossref] [PubMed]