Strengthening of antitumor effects in breast cancer from a novel B7-H4- and CD3-targeting bispecific antibody by an oncolytic virus
Introduction
Breast cancer (BC), the most common cancer in women, has three major clinically relevant subtypes (i.e., hormone receptor (HR) positive, human epidermal growth factor receptor 2 (HER2) positive and triple-negative). Compared to triple-negative breast cancer (TNBC) in metastatic setting, the treatment strategies of which used to be limited to chemotherapy, other two subtypes of BC have more treatment options, such as endocrine and anti-HER2-based therapy, other than chemotherapy (1). Over the last decade, Cytotoxic T Lymphocyte-associated antigen-4 (CTLA-4) (2) and programmed cell death protein 1 (PD-1) (3)/programmed death-ligand 1 (PD-L1) (4) inhibitors have become a routine part of treatment for nearly 50 different cancer indications, offering the possibility of long-term clinical response and cure for many patients with metastatic cancers (5,6). In TNBC, the median overall survival (mOS) used to be only 12–18 months in the metastatic setting due to limited treatment options until the treatment landscape started to change and the use of atezolizumab (7) and pembrolizumab (8,9), in combination with other therapies (10), offered new opportunities to extend survival in both early and advanced PD-L1+ TNBC. However, TNBC has long been considered to be a ‘cold’ immune microenvironment (TME) tumor with poor therapeutic effect, due to the relatively low level of T cell infiltration and mutational burden compared to melanoma or non-small cell lung cancer (11,12). Therefore, to permit precision deployment of immunotherapies beyond immune checkpoint inhibitors (ICIs), more effective therapeutic strategies need to be achieved through deeper understanding of other immune coinhibitors, like B7-H4, in addition to the attention that has been focused on co-stimulatory receptors, such as OX40 and 4-1BB/CD137 agonists, to boost overall antitumor immunity (13).
B7-H4, also known as B7x, B7S1, or VTCN1, is a coinhibitory molecule that is overexpressed across cancer cells. B7-H4 negatively regulates inflammatory CD4+ T cells, by which cancer cells evade antitumor immune responses (14-16). It was reported that the expression levels of B7-H4 were high in different subtypes of breast cancer (17). Increased B7-H4 expression levels on breast cancer cells were significantly correlated with one or more clinicopathological factors, including increased TNM malignant tumor score, decreased survival, and decreased number of tumor-infiltrating T cells, which was a significant independent prognostic indicator (18,19). The strategies to block B7-H4 include the use of classical monoclonal antibodies (mAbs) (20). However, previous data showed that anti-human B7-H4 mAbs alone were not sufficient to suppress the tumor growth in a mouse model (20). The bispecific antibodies targeting B7-H4 and CD3 have recently been discovered to have antitumor effects in TNBC (21).
More recently, the quest to improve the response rate of immunotherapy has prompted the combinations with other established drugs targeting multiple compensatory pathways (22). Chemotherapy and radiation are widely used in clinical practice to treat TNBC but the tolerability is generally poor. Other combination strategies, such as oncolytic viruses (OVs) and immunotoxins, are currently under scientific and clinical investigation and are worthwhile-to-try selections that may potentially open the door to high-order immunotherapy combinations (23-25). Oncolytic viruses are emerging as effective strategy in cancer treatment. A variety of viruses has been explored as potential cancer therapeutic agents (26). A novel oncolytic herpes simplex virus (HSV-2), oHSV2hGM-CSF (oHSV2), which was developed by our group, produces a more potent antitumor response than HSV-1 through 2 major mechanisms of action (27): one of which is the ability of selectively replicating within tumors and directly inducing cell lysis; and another is that OVs improves antigen cross-priming and recruitment of immune cells into TME, eliciting antitumor immunity. Our previous work demonstrated oHSV2 obviously promotes tumor regression and produces almost no side effects in preclinical mice models in vivo. Throughout the course of the study, the body weight of mice remained relatively stable and the pathologic changes in the main organs (lung, heart, liver, spleen, and kidney) were not observed (28). Apart from OVs, immunotoxins, which are cytolytic fusion proteins and composed of a targeting moiety, commonly a single-chain variable fragment (scFv) from of an antibody targeting a specific antigen on tumor cells and linked to a toxin, developed for cancer therapy (23,24). To date, two immunotoxins, moxetumomab pasudotox (Lumoxiti) and tagraxofusp (Elzonris), were approved by the Food and Drug Administration (FDA) for the treatment of relapsed or refractory hairy cell leukemia and blastic plasmacytoid dendritic cell neoplasm (29,30), respectively. Based on analysis of the aforementioned mechanism and reasonable speculation, current project aims to explore the efficacy and safety of B7-H4-targeting bispecific antibodies and immunotoxins in breast cancer. Further, it is also worthwhile to investigate whether oncolytic virus can enhance anti-tumor responses driven by bispecific antibodies. We present the following article in accordance with the ARRIVE reporting checklist (available at https://atm.amegroups.com/article/view/10.21037/atm-22-3423/rc).
Methods
Cell lines
Two human breast cancer cell lines (SKBR3 and ZR75) and a B7-H4-negative A431 cell line (human squamous cell carcinoma cell line) were maintained as adherent monolayer cultures in Dulbecco’s Modified Eagle Medium (DMEM, from Invitrogen, Carlsbad, CA) with supplement of 2 mM L-glutamine, 10% fetal bovine serum (FBS), and 100 IU/mL penicillin-streptomycin and incubated in 5% CO2 at 37 ℃. All cell lines (A431, SJBR3 and ZR75) were purchased from the Cell Bank of the Chinese Academy of Science. A431 cells were transfected with a plasmid encoding the full-length B7-H4 gene (GenBank accession no NM_024626.2), engineered to overexpress B7-H4. Both the B7-H4-negative A431 and the B7-H4 overexpressed A431 (B7-H4) cells (Feng Lab, Huazhong Agricultural University, Wuhan, China) were maintained in DMEM. All cells were cultured under aseptic conditions, with the media being refreshed every 3 days.
Preparation of recombinant B7-H4 proteins
After fusing the extracellular domain (ECD) of B7-H4 (NM_024626.2) with human IgG1-Fc, we cloned the fusion into mammalian expression vector pFUSE-hIgG1-Fc2 (cat. no. pfuse-hg1fc2; Invitrogen) to generate B7H4-hFc. The expression vectors were then introduced into 293F cells by polyethylenimine (PEI) transfection. The transfected cells were cultured in SMM 293-TII Expression Medium (cat. no. M293TII; Sino Biological Inc., Beijing, China). The expressed protein was secreted into the cell culture medium and purified by protein A affinity chromatography (cat. no. C600952-0501; Sangon Biotech Co., Ltd; Shanghai, China). The purity of the preparations was analyzed by SDS-PAGE (31).
Generation of B7-H4 mAbs
Recombinant B7-H4-hFc was used to immunize 4 mice by subcutaneous injection of 100 µg of protein mixed with 100 µL of Complete Freund’s adjuvant (Sigma-Aldrich; Merck KGaA, Burlington, MA, USA). Fourteen days later, mice were boosted with half the amount of the protein mixed with Incomplete Freund’s adjuvant. One week later after boosting, the immunized spleens were harvested according the protocol (32). The total splenic RNAs were isolated using TRIzol (cat. no. 15596018; Invitrogen ThermoFisher Scientific, Inc.) and reverse transcribed into complement cDNA using a PrimeScript kit (Takara Bio, Inc., Shiga, Japan). Polymerase chain reaction (PCR) amplification of the scFv fragments and construction of the phage display library was performed as previously described (33). The resultant library contained about 108 original transformants. Screening of the phage library followed the previous protocol (34).
Production of B7-H4 mAbs
Recombinant scFv of the B7-H4 binders was fused with the rFc tag for purification and detection purposes, inserted into the expression vector, pFUSE-hIgG1-Fc2 (pfuse-hg1fc2; InvivoGen, San Diego, CA, USA); and expressed as a scFv-rFc format in 293F cells according to the aforementioned method. The rFc tag was used in the cell binding assay through flow cytometry.
Enzyme-linked immunosorbent assay
5 µg/mL B7H4-hFc protein was coated on a 96-well MaxiSorp plate and incubated at 37 ℃ for 30 min. After washing the plate twice with Phosphate buffer saline tween (PBST), blocking buffer [2% bovine serum albumin (BSA) in Phosphate buffered solution (PBS) buffer] was added to the plate and blocked for 30 min with. After removing the blocking buffer, biotinylated B7-H4 mAbs (scFv-rFc format) were incubated within the plate at indicated concentrations starting from 200 µg/mL and followed by 1:2 serial dilutions. Binding of B7-H4 mAbs was detected with horseradish peroxidase (HRP)-conjugated streptavidin (Invitrogen; Thermo Fisher Scientific, Inc.; 1:4,000).
Flow cytometry
To test the cell surface expression of B7-H4, cancer cells were coincubated with B7-H4 mAbs (scFv-rFc) of 10 µg/mL for an hour on ice. Cell binding ability of antibodies was detected with phycoerythrin-conjugated goat-anti-rabbit polyclonal antibodies (Jackson ImmunoResearch Laboratories, Inc., West Grove, PA, USA). We use a FACS Calibur Flow Cytometer (BD Biosciences, Franklin Lakes, NJ, USA) to measure the fluorescence intensity.
Production of B7-H4 bispecific antibodies and immunotoxins
To make the B7-H4 bispecific antibody, the coding sequences of B7-H4 scFv were fused with a (G4S)3 linker and then with OKT3 scFv and hFc knob mutants (N297A/S354C/T366W). The expression cassette was cloned into a pFUSE vector and introduced into Expi293 cells (Gibco, Thermo Fisher Scientific). Purification of the bispecific antibodies was performed by using a protein A column.
Oncolytic viruses
The OV, oHSV2hGM-CSF (HG52/34.5-/47-hGM-CSF), was used in the current project. The virus was produced in a good manufacturing production (GMP) facility by Wuhan Binhui, LLC (www.binhui-bio.com.cn; Wuhan, China) according to standard protocols (batch no. VBT-oHSV2-hGM-CSF-201908A-KZH-NS) (27). The virus was resuspended in preparation buffer and stored at –70 ℃ in aliquots to avoid repeated freezing and thawing.
In vitro cytotoxicity assays
Cytotoxicity of B7-H4 bispecific antibody, immunotoxins, and the OVs was measured through a luciferase reporter assay. To constitutively express the firefly luciferase gene (ffLuc2), target cells were stably transfected with a lentiviral vector. For the assay, target cells were cultured on 96-well tissue-culturing plates at 10,000 cells per well and cytotoxic agent was added to each well at the indicated concentrations. After 3 days of co-incubation in a CO2 incubator, cell viability was quantified by measuring the intracellular luciferase activity according to previously published method (31).
In vivo efficacy study
NSG mice (5-week-old, female, average weight ~21 g; provided by the Animal Facility of Huazhong Agricultural University, Wuhan, China) were assigned to different groups, 5 mice per group. Mice were subcutaneously injected with 2×106 A431 (B7H4) cells in a volume of 200 µL serum-free DMEM. When tumor grew to 100–200 mm3, treatments were started. Tumor growth was measured with a caliper every 2 days. The tumor volume (mm3) was calculated using the following formula: (a × b2)/2, where a is the length of tumor and b is the width of tumor in mm. When the tumor size exceeded 1,600 mm3, mice were sacrificed by anesthesia, and serum and tumor masses were collected for further analysis.
In a dose escalation experiment, the B7H4 bispecific antibody M16-OKT3-hFc was administered intravenously at 0.5, 1.0 and 2.0 mg/kg every three days for a total of five injections. B7H4 immunotoxin M16-PE24 was intravenously administered at 2.5, 5.0 and 10.0 mg/kg on alternate days for a total of 6 injections.
In the bispecific antibody-oncolytic virus combination study, six groups of mice were set up with five mice in each group. The PBS control group received only PBS as solvent. The peripheral blood mononuclear cell (PBMC) group was treated once with 107 PBMC by tail vein injection. The OVs group was treated with HSV-2 oncolytic virus at a dose 150 µL by intratumoral administration every other day for three injections. The OVs and PBMC group was treated with PBMC and OVs in the same way as the OVs group and PBMC group. The bispecific antibody group was treated with 107 PBMC and 1 mg/kg B7H4-M16-OKT3-hFc via tail vein injection once every three days for a total of five injections. The bispecific/oncolytic combination group was exactly the treatment of bispecific antibody plus that of oncolytic virus (Table 1). All the procedures used in the animal studies were approved by the Animal Care and Use Committee of Huazhong Agricultural University (approval No. HZAUMO-2017-051), in compliance with institutional guidelines for the care and use of animals. Peripheral blood materials used in this study were obtained from healthy donors with informed consent. The study was conducted in accordance with the Declaration of Helsinki (as revised in 2013). The use of human blood samples was reviewed and approved by Biomedical Research Ethical Committee, Huazhong Agricultural University (approval No. HZAUHU-2017-002).
Table 1
Groups | Treatments | Schedule (days post inoculation) |
---|---|---|
Control | PBS | – |
PBMC control | PBMC | D10 |
Bispecific antibody + PBMCs | B7H4-M16-OKT3-hFc and PBMCs | D10 for PBMCs; d10, d13, d16, d19, and d22 for B7H4-M16-OKT3-hFc |
OVs | OVs | D10, d12, and d14 for OVs |
OVs + PBMCs | OVs and PBMCs | D10 for PBMCs; d10, d12, and d14 for OVs |
Bispecific antibody + OVs + PBMCs | B7H4-M16-OKT3-hFc, OVs, and PBMCs | D10 for PBMCs; d10, d13, d16, d19, and d22 for B7H4-M16-OKT3-hFc; d10, d12, and d14 for OVs |
PBS, phosphate-buffered saline; PBMC, peripheral blood mononuclear cell; OVs, oncolytic viruses.
Statistical analysis
All statistical analyses were conducted using GraphPad Prism9.3.0 (GraphPad Software, La Jolla, CA, USA). The data are expressed as the mean ± standard error of mean (SEM). Comparisons between two groups were performed using an unpaired Student’s t-test (2-tailed). Comparisons among three and more groups were performed using a one-way analysis of variance (ANOVA) followed by Tukey’s multiple comparison test. P value <0.05 was considered to indicate a significant difference.
Results
Generation and binding properties of B7-H4 mAbs
The extracellular domain (ECD) of B7-H4 was fused with human IgG1 Fc (B7H4-hFc). Recombinant B7H4-hFc was purified via protein A chromatography. SDS-PAGE analysis showed that the protein may be highly glycosylated (Figure 1A), which was consistent with the prediction that the protein has seven predicted N-glycosylation sites. Purified B7H4-hFc was used to immunize mice and to generate the mAbs M2, M16, M37, and M64 via phage display according to published protocol (33). The scFv sequences of the mAbs were fused with rabbit Fc (scFv-rFc) and expressed in 293F cells. SDS-PAGE analysis of the recombinant antibodies revealed high homogeneousness (Figure 1B). ELISA analysis showed that all mAbs had high affinity binding to recombinant B7-H4, with the calculated EC50 values of being 0.1 to 0.4 nM (Figure 1C). Flow cytometry analysis showed that all mAbs bound to B7-H4-overexpressed A431 cells (Figure 1D). Since M37 had the highest affinity to cell surface B7-H4, it was used to screen B7-H4 positive breast cancer cell lines. As shown in Figure 1E, M37 did not bind to A431 cells known to be B7-H4 negative, but efficiently bound to B7-H4 overexpressing A431 cells as well as to the native breast cancer cells ZR75 and SKBR3.
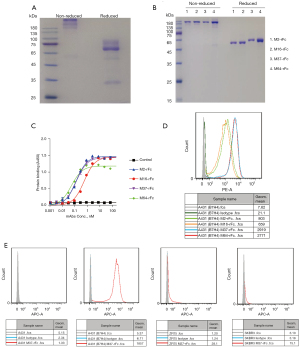
Generation of B7-H4 bispecific antibodies and immunotoxins
B7-H4 bispecific antibody was constructed by fusing the scFv of M2, M16, M37, and M64 with that of CD3-targeted antibody OKT3, followed by hFc knob mutant with N297A mutation (Figure 2A). Immunotoxin was constructed by fusing afore-mentioned scFv of B7-H4 mAbs with a truncated form of pseudomonas exotoxin A (PE24) (Figure 2B). The bispecific antibodies were expressed in 293F cells and purified via protein A column (Figure 2C), and immunotoxins were expressed in E. coli and purified via nickel column (Figure 2D). Binding activity of the purified proteins was confirmed by ELISA for both bispecific antibodies (Figure 2E) and immunotoxin (Figure 2F), all of which showed similar affinities except M37 immunotoxin. The calculated EC50 values for bispecific antibodies were about 0.4 nM, while the EC50 values for immunotoxins were about 0.1 nM. Cell surface B7-H4 binding of the bispecific antibodies and immunotoxins was also confirmed by flow cytometry (Figure 2G), where Jurkat cells and PBMC were used as CD3 positive cells, while A431 (B7H4) cells that were forcefully overexpressing B7-H4 were used as B7-H4 positive cells. In the flow cytometry, the bispecific antibody M37 and M64 showed much stronger binding compared to M2 and M16, which was consistent with the binding of monoclonal antibodies.
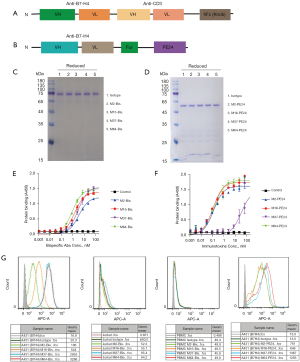
In vitro cytotoxicity of B7-H4 bispecific antibodies and immunotoxins
Cytotoxicity of the B7-H4 bispecific antibodies and immunotoxins was detected in the B7H4-negative A431 cells and A431 cells that were forcefully overexpressing B7-H4 [named A431 (B7H4)], as well as in B7-H4-positive breast cancer cells SKBR3 and ZR75. A431 cells were used as a control for non-specific toxicity independent of the antigen. As shown in Figure 3, bispecific antibodies had no detectable toxicity to A431 cells (Figure 3A), but potently killed A431 (B7H4) in the presence of unstimulated PBMC (Figure 3B), and moderately killed native breast cancer cells ZR75 (Figure 3C) and SKRB3 (Figure 3D). Unlike the bispecific antibodies, while the immunotoxin was not toxic to A431 (Figure 3E) and had similar potency against A431 (B7H4) in a dose-dependent manner (Figure 3D), its potency against ZR75 and SKBR3 was almost negligible (Figure 3G,3H), probably due to the relatively low expression level of B7-H4 on the native cell lines
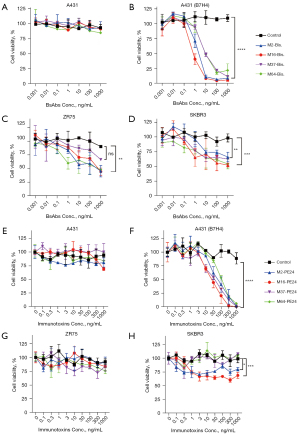
In vivo efficacy of B7-H4 bispecific antibodies and immunotoxins
M16-based bispecific antibodies and immunotoxins were selected for in vivo studies because of their relatively stronger activity in the in vitro cytotoxicity assays. The in vivo antitumor efficacy was tested in NSG xenograft mice. As shown in Figure 4A, dose escalating test of bispecific antibody at 0.5–2.0 mg/kg had comparable efficacy that partially suppressed tumor growth, while 1.0 and 2.0 mg/kg caused significant body weight loss (Figure 4B), suggesting a limited efficacy of bispecific antibody in controlling tumor growth. However, immunotoxins at 1.0–10.0 mg/kg dramatically inhibited tumor growth (Figure 4C), though 5.0 and 10.0 mg/kg doses also caused significant body weight loss (Figure 4D). Taken together, immunotoxin was superior to bispecific antibody in terms of efficacy and safety.
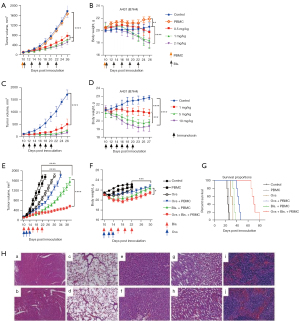
Our previous work had shown that HSV-2 oncolytic viruses produced strong anti-tumor response through antigen cross-priming and recruitment of immune cells into TME (27), therefore it would be interesting to know whether oncolytic virus could boost the efficacy of bispecific antibody. As expected, combination of oncolytic virus with low dose of bispecific antibody significantly inhibited tumor growth compared to monotherapy (oncolytic virus or bispecific antibody alone) (Figure 4E), and the combination did not cause dramatic body weight loss (Figure 4F), which was in contrast to the treatment with high doses of bispecific antibody. Further, combination significantly prolonged the survival time (Figure 4G). To examine any possible damages in the main organs of mice treated by monotherapy and combination therapy, tissue section and H&E staining of heart, lung, liver, kidney and spleen was performed. The results showed that the immune response caused by bispecific antibodies, oncolytic virus alone or in combination resulted in a significant and rapid tumor cell clearance and did not cause obvious damage to the organs (40× micrograph) (Figure 4H). In summary, our data supported a more significantly synergistic inhibition of tumor growth by combination of bispecific antibody and oncolytic virus, which was worthy of further clinical investigation.
Discussion
Despite the unprecedented clinical benefits from immunotherapy observed in subsets of breast cancer patients, the majority of TNBC patients show limited responses to single-agent anti-PD-1 or PD-L1 treatment. Therefore, there is still a need to identify new targets and develop optimal immunotherapy strategies to improve the efficacy of breast cancer. B7-H4 is a promising new target for cancer immunotherapy. It has been reported that B7-H4 was selectively expressed in breast cancer and other cancer types, with minimal expression on normal adult tissues. To explore the potential of B7-H4-targeted immunotherapy in breast cancer, current study generated four mouse monoclonal antibodies, M2, M16, M37, and M64. Among them, M16-based bispecific antibody and immunotoxin had a relatively lower cell binding affinity (Figure 2G), however they exhibited the strongest cancer cell killing activity (Figure 3B-3H), indicating that cell binding affinity of bispecific antibody and immunotoxin does not necessarily correlate with their cytotoxic potency. In some cases, the epitope may be more important than affinity. Similar studies on GPC3-targeted immunotherapy demonstrated that bispecific antibody that targets the C-terminal had stronger potency (35), while immunotoxins target the N-terminal of GPC3 had stronger potency (36).
It has been shown that T cell-engaged bispecific antibody and CAR-T may not need high level of antigen density on cell surface for efficient tumor killing (31). In the current study, we found similar phenomenon. Although ZR75 and SKBR3 had very low level of B7-H4 expression (Figure 1E), they were sensitive to T cell-engaged bispecific antibody treatment (Figure 3C,3D). However, low antigen density is not sufficient for immunotoxin-based therapy (Figure 3G,3H). Therefore, T cell-engaged immunotherapy may be more suitable for breast cancer that targets B7-H4.
Recently, studies have highlighted the critical role of the immune system in the control of cancer progression/regression, which provides a framework for investigating various immunotherapy approaches, including cancer vaccines, adoptive T cell transfer, and immune checkpoint inhibitors such as anti-PD-1/PD-L1 monoclonal antibodies. Oncolytic virus improves the immunogenic properties of cancer cells by enhancing their necrosis and pyroptosis and their capacity to elicit CD8+ interferon-γ-producing T cell responses in tumor microenvironment. Our previous work demonstrated that oHSV2 obviously promotes tumor regression and produces almost no side effects in preclinical mice models in vivo (28), and in patients with metastatic esophageal and rectal cancer, intratumoral injection of oHSV2 was well-tolerated, and demonstrated durable antitumor activity (37). For the purpose of improving the efficacy of T cell-engaged bispecific antibody, oHSV2, which produces a more potent antitumor response than HSV-1 through 2 major mechanisms of action mentioned above (27), are one of the optimal choices. Indeed, our study supported the combination of B7-H4-targeted bispecific antibody with oncolytic virus, which demonstrated a significantly synergistic effect in suppressing tumor growth in xenograft mouse models (Figure 4E). Further, the combination was considerably safe (Figure 4F,4H), and therefore warrants further clinical investigation.
Collectively, our findings uncover a novel strategy that the interrogation of targeting multiple pathways is able to reinforce anti-tumor immune response driven by B7-H4-targeting bispecific antibody in TNBC through high-order immunotherapy combinations. However, there are still some shortcomings in our study, for example, more antibodies with higher B7-H4 affinity and epitope coverage need to be tested thus further research is preferred.
Acknowledgments
Funding: The present study was supported by the Natural Science Foundation of China (No. 81972308) and the Key Frontier Project of Application Foundation of Wuhan Science and Technology Bureau (No. 2019020701011438).
Footnote
Reporting Checklist: The authors have completed the ARRIVE reporting checklist. Available at https://atm.amegroups.com/article/view/10.21037/atm-22-3423/rc
Data Sharing Statement: Available at https://atm.amegroups.com/article/view/10.21037/atm-22-3423/dss
Conflicts of Interest: All authors have completed the ICMJE uniform disclosure form (available at https://atm.amegroups.com/article/view/10.21037/atm-22-3423/coif). The authors have no conflicts of interest to declare.
Ethical Statement: The authors are accountable for all aspects of the work in ensuring that questions related to the accuracy or integrity of any part of the work are appropriately investigated and resolved. All the procedures used in the animal studies were approved by the Animal Care and Use Committee of Huazhong Agricultural University (approval No. HZAUMO-2017-051), in compliance with institutional guidelines for the care and use of animals. Peripheral blood materials used in this study were obtained from healthy donors with informed consent. The study was conducted in accordance with the Declaration of Helsinki (as revised in 2013). The use of human blood samples was reviewed and approved by Biomedical Research Ethical Committee, Huazhong Agricultural University (approval No. HZAUHU-2017-002).
Open Access Statement: This is an Open Access article distributed in accordance with the Creative Commons Attribution-NonCommercial-NoDerivs 4.0 International License (CC BY-NC-ND 4.0), which permits the non-commercial replication and distribution of the article with the strict proviso that no changes or edits are made and the original work is properly cited (including links to both the formal publication through the relevant DOI and the license). See: https://creativecommons.org/licenses/by-nc-nd/4.0/.
References
- Gennari A, Andre F, Barrios CH, et al. ESMO Clinical Practice Guideline for the diagnosis, staging and treatment of patients with metastatic breast cancer. Ann Oncol 2021;32:1475-95. [Crossref] [PubMed]
- Ribas A, Camacho LH, Lopez-Berestein G, et al. Antitumor activity in melanoma and anti-self responses in a phase I trial with the anti-cytotoxic T lymphocyte-associated antigen 4 monoclonal antibody CP-675,206. J Clin Oncol 2005;23:8968-77. [Crossref] [PubMed]
- Topalian SL, Hodi FS, Brahmer JR, et al. Safety, activity, and immune correlates of anti-PD-1 antibody in cancer. N Engl J Med 2012;366:2443-54. [Crossref] [PubMed]
- Brahmer JR, Tykodi SS, Chow LQ, et al. Safety and activity of anti-PD-L1 antibody in patients with advanced cancer. N Engl J Med 2012;366:2455-65. [Crossref] [PubMed]
- Sharma P, Siddiqui BA, Anandhan S, et al. The Next Decade of Immune Checkpoint Therapy. Cancer Discov 2021;11:838-57. [Crossref] [PubMed]
- Yap TA, Parkes EE, Peng W, et al. Development of Immunotherapy Combination Strategies in Cancer. Cancer Discov 2021;11:1368-97. [Crossref] [PubMed]
- Emens LA, Adams S, Barrios CH, et al. First-line atezolizumab plus nab-paclitaxel for unresectable, locally advanced, or metastatic triple-negative breast cancer: IMpassion130 final overall survival analysis. Ann Oncol 2021;32:983-93. [Crossref] [PubMed]
- Cortes J, Cescon DW, Rugo HS, et al. Pembrolizumab plus chemotherapy versus placebo plus chemotherapy for previously untreated locally recurrent inoperable or metastatic triple-negative breast cancer (KEYNOTE-355): a randomised, placebo-controlled, double-blind, phase 3 clinical trial. Lancet 2020;396:1817-28. [Crossref] [PubMed]
- Winer EP, Lipatov O, Im SA, et al. Pembrolizumab versus investigator-choice chemotherapy for metastatic triple-negative breast cancer (KEYNOTE-119): a randomised, open-label, phase 3 trial. Lancet Oncol 2021;22:499-511. [Crossref] [PubMed]
- Bianchini G, De Angelis C, Licata L, et al. Treatment landscape of triple-negative breast cancer - expanded options, evolving needs. Nat Rev Clin Oncol 2022;19:91-113. [Crossref] [PubMed]
- Marra A, Viale G, Curigliano G. Recent advances in triple negative breast cancer: the immunotherapy era. BMC Med 2019;17:90. [Crossref] [PubMed]
- Bonaventura P, Shekarian T, Alcazer V, et al. Cold Tumors: A Therapeutic Challenge for Immunotherapy. Front Immunol 2019;10:168. [Crossref] [PubMed]
- Marin-Acevedo JA, Dholaria B, Soyano AE, et al. Next generation of immune checkpoint therapy in cancer: new developments and challenges. J Hematol Oncol 2018;11:39. [Crossref] [PubMed]
- Sica GL, Choi IH, Zhu G, et al. B7-H4, a molecule of the B7 family, negatively regulates T cell immunity. Immunity 2003;18:849-61. [Crossref] [PubMed]
- Prasad DV, Richards S, Mai XM, et al. B7S1, a novel B7 family member that negatively regulates T cell activation. Immunity 2003;18:863-73. [Crossref] [PubMed]
- Zang X, Loke P, Kim J, et al. B7x: a widely expressed B7 family member that inhibits T cell activation. Proc Natl Acad Sci U S A 2003;100:10388-92. [Crossref] [PubMed]
- Leong SR, Liang WC, Wu Y, et al. An anti-B7-H4 antibody-drug conjugate for the treatment of breast cancer. Mol Pharm 2015;12:1717-29. [Crossref] [PubMed]
- Mugler KC, Singh M, Tringler B, et al. B7-h4 expression in a range of breast pathology: correlation with tumor T-cell infiltration. Appl Immunohistochem Mol Morphol 2007;15:363-70. [Crossref] [PubMed]
- Huang H, Li C, Ren G. Clinical significance of the B7-H4 as a novel prognostic marker in breast cancer. Gene 2017;623:24-8. [Crossref] [PubMed]
- Iizuka A, Kondou R, Nonomura C, et al. Unstable B7-H4 cell surface expression and T-cell redirection as a means of cancer therapy. Oncol Rep 2016;36:2625-32. [Crossref] [PubMed]
- Iizuka A, Nonomura C, Ashizawa T, et al. A T-cell-engaging B7-H4/CD3-bispecific Fab-scFv Antibody Targets Human Breast Cancer. Clin Cancer Res 2019;25:2925-34. [Crossref] [PubMed]
- Galvan Morales MA, Barrera Rodriguez R, Santiago Cruz JR, et al. Overview of New Treatments with Immunotherapy for Breast Cancer and a Proposal of a Combination Therapy. Molecules 2020;25:5686. [Crossref] [PubMed]
- Li M, Liu ZS, Liu XL, et al. Clinical targeting recombinant immunotoxins for cancer therapy. Onco Targets Ther 2017;10:3645-65. [Crossref] [PubMed]
- Kim JS, Jun SY, Kim YS. Critical Issues in the Development of Immunotoxins for Anticancer Therapy. J Pharm Sci 2020;109:104-15. [Crossref] [PubMed]
- Lawler SE, Speranza MC, Cho CF, et al. Oncolytic Viruses in Cancer Treatment: A Review. JAMA Oncol 2017;3:841-9. [Crossref] [PubMed]
- Miao L, Fraefel C, Sia KC, et al. The potential application of a transcriptionally regulated oncolytic herpes simplex virus for human cancer therapy. Br J Cancer 2014;110:94-106. [Crossref] [PubMed]
- Wang Y, Jin J, Wu Z, et al. Stability and anti-tumor effect of oncolytic herpes simplex virus type 2. Oncotarget 2018;9:24672-83. [Crossref] [PubMed]
- Yang H, Peng T, Li J, et al. Treatment of colon cancer with oncolytic herpes simplex virus in preclinical models. Gene Ther 2016;23:450-9. [Crossref] [PubMed]
- Janus A, Robak T. Moxetumomab pasudotox for the treatment of hairy cell leukemia. Expert Opin Biol Ther 2019;19:501-8. [Crossref] [PubMed]
- Syed YY. Tagraxofusp: First Global Approval. Drugs 2019;79:579-83. [Crossref] [PubMed]
- Chen X, Amar N, Zhu Y, et al. Combined DLL3-targeted bispecific antibody with PD-1 inhibition is efficient to suppress small cell lung cancer growth. J Immunother Cancer 2020;8:e000785. [Crossref] [PubMed]
- Association APoEAVM. 2000 Report of the AVMA Panel on Euthanasia. J Am Vet Med Assoc 2001;218:669-96. [Crossref] [PubMed]
- Dong S, Bo Z, Zhang C, et al. Screening for single-chain variable fragment antibodies against multiple Cry1 toxins from an immunized mouse phage display antibody library. Appl Microbiol Biotechnol 2018;102:3363-74. [Crossref] [PubMed]
- Feng M, Gao W, Wang R, et al. Therapeutically targeting glypican-3 via a conformation-specific single-domain antibody in hepatocellular carcinoma. Proc Natl Acad Sci U S A 2013;110:E1083-91. [Crossref] [PubMed]
- Chen X, Chen Y, Liang R, et al. Combination Therapy of Hepatocellular Carcinoma by GPC3-Targeted Bispecific Antibody and Irinotecan is Potent in Suppressing Tumor Growth in Mice. Mol Cancer Ther 2022;21:149-58. [Crossref] [PubMed]
- Li J, Xiang L, Wang Q, et al. Highly Potent Immunotoxins Targeting the Membrane-distal N-lobe of GPC3 for Immunotherapy of Hepatocellular Carcinoma. J Cancer 2022;13:1370-84. [Crossref] [PubMed]
- Zhang B, Huang J, Tang J, et al. Intratumoral OH2, an oncolytic herpes simplex virus 2, in patients with advanced solid tumors: a multicenter, phase I/II clinical trial. J Immunother Cancer 2021;9:e002224. [Crossref] [PubMed]
(English Language Editor: J. Gray)