Correlation between cholecystectomy and development of non-alcoholic liver disease in the mouse model
Introduction
Cholecystectomy, which is a common surgical procedure worldwide, is a therapeutic strategy for cholecystitis and symptomatic gall stone disease. Although cholecystectomy is not reported to affect the total bile acid levels, it may modulate glucose and lipid homeostasis through different mechanisms (1,2). Glucose homeostasis is impaired in patients undergoing cholecystectomy due to increased postprandial glucose fluctuations and decreased postprandial duodenal bile acid concentration (3). Chen et al. suggested that some patients may not respond to cholecystectomy and exhibit a rapid release of bile acids into the intestine after meal intake. The lack of synchronization between the amount of bile acid and food results in metabolic stress and altered receptor activation [especially farnesoid X receptor (FXR)]. Activated FXR improves insulin sensitivity and downregulates the plasma glucose level by downregulating gluconeogenesis and upregulating glycogen synthesis (4). One study suggested that human gallbladder (GB) mucosa functions as an endocrine organ by secreting FGF19 into bile (5). The microbial diversity in patients with NAFLD is reported to be lower than that in healthy controls (6). Cholecystectomy impairs aging-related changes in gut microbial composition (7). Patients who have undergone cholecystectomy exhibit an increased abundance of bacterial species involved in bile acid metabolism (8). Bacterial dysbiosis-associated production of metabolites can be a feedback signal to the host (9). The contribution of the microbiome and other factors to the pathogenesis of NASH is unclear (10). The effect of cholecystectomy on bile composition and the metabolic network remains controversial.
Non-alcoholic fatty liver disease (NAFLD) is the most common chronic liver disease (11-13). Globally, rapid changes in lifestyle and diet have contributed to an increased prevalence of obesity and NAFLD. However, the ability of cholecystectomy to induce NAFLD is unknown. Various mechanisms have been suggested to be involved in the development of NAFLD although the exact mechanisms have not been elucidated. One potential mechanism underlying the development of NAFLD involves changes in bile acid composition or level (14-16). Several population-based studies in the United States have demonstrated that cholecystectomy is an independent risk factor for the occurrence of NAFLD (17). Similar results were obtained in a study comparing Asian patients subjected to cholecystectomy and those with GB stones as controls (18-20). However, a large population-based study conducted in China revealed the lack of correlation between cholecystectomy and NAFLD occurrence (21).
Clinical studies have suggested a strong correlation between cholecystectomy and the incidence of NAFLD (17-20), although the exact correlation and causal relationship are unclear. This study aimed to investigate whether cholecystectomy increases the occurrence of NAFLD or aggravates pre-existing NAFLD. We present the following article in accordance with the ARRIVE reporting checklist (available at https://atm.amegroups.com/article/view/10.21037/atm-21-5414/rc).
Methods
Study design
Hepatic histological analysis was performed to investigate the effect of cholecystectomy on the healthy liver and pre-existing fatty liver. In particular, the effects of standard diet or 60% high-fat (HF) diet (Research Diets Inc., NJ, USA) on mouse hepatic histological parameters were evaluated at months 1, 2, and 4 post-surgery using liver biopsy.
Sequential liver biopsy
The changes in liver histological parameters were tracked at months 1, 2, and 4 post-surgery in the same mice without sacrificing them. Sequential liver biopsy was performed following a previously reported protocol (22). The first liver biopsy was performed in the left lateral lobe, while the second follow-up liver biopsy was performed in the left median lobe. Briefly, the mice were anesthetized by intraperitoneally injecting Zoletil and Rompun (1:1) before liver biopsy. The abdominal site was shaved and disinfected using a 10% iodine solution. Next, a midline incision was introduced, and a biopsy sample was collected from the left lateral lobe of the liver. A heated spatula was placed on the bleeding liver site to ensure hemostasis. The abdomen was sutured with a device. The same procedure was used for the second biopsy at the left median lobe.
Animal experiments (study 1): cholecystectomy in standard diet-fed mice
Twenty male C57BL6N mice (aged 8 weeks; Orient Animal Laboratory, Seoul, South Korea) were fed a standard diet (standard chow) and observed for 16 weeks. The mice were maintained under a 12/12 h light/dark cycle. All mice were divided into the following two groups: sham and cholecystectomy groups. To perform cholecystectomy, the mice were anesthetized by intraperitoneally injecting Zoletil and Rompun (1:1). After introducing a midline incision, the cystic duct was ligated and the GB was removed. The abdominal wall was closed in two layers after the surgery. Mice in the sham group underwent the same procedure without GB removal. At months 1, 2, and 4 post-surgery, the animals were euthanized, and serum and liver samples were obtained for further analysis.
Animal experiments (study 2): cholecystectomy in the HF diet-fed mice
Male C57BL/6N mice (Orient Animal Laboratory, Seoul, South Korea) aged 8 weeks were used to establish the fatty liver model. Mice were allowed to adapt for 1 week and fed on a 60% HF diet (Research Diets Inc, NJ, USA) for 4 weeks. The animals were then randomly divided into the sham (n=11) and cholecystectomy (n=14) groups. After cholecystectomy, the mice were maintained on HF diet. Liver biopsy was performed at months 2, 3, and 6 post-surgery. The total duration of the experiment was 24 weeks. Cholecystectomy was performed as described above. Mice in the sham group underwent the same surgical procedure without removal of the GB. The animals were followed up for 20 weeks after cholecystectomy. After 24 weeks, the mice were euthanized, and the serum and the liver tissue were harvested and frozen. The bodyweight of the mice was evaluated once every week. Liver weight and liver-to-body weight ratio were assessed. Experiments were performed under a project license (No. HY-IACUC-19-0022) granted by the Hanyang University Institutional Animal Care and Use Committee, in compliance with institutional guidelines for the care and use of animals.
Histological assessment of liver biopsy samples
Liver histological analysis was performed by a pathologist. The NASH clinical research network scoring system was used for histological grading (23). Steatosis, hepatocyte ballooning, and lobular inflammation were semi-quantitatively scored. The NAFLD activity score (NAS) was assessed by combining steatosis, hepatocyte ballooning, and lobular inflammation scores. Based on NAS, the cumulative scores of 0–2, 3–4, and, >5 were assigned as non-NASH, borderline NASH, and probable NASH, respectively (23). Hematoxylin and eosin (H&E) staining was performed to evaluate NAFLD. All histological images were re-evaluated by an independent pathologist who was blinded to the experimental group.
Histopathological analysis
Liver tissue fixed in formalin was trimmed and dehydrated to prepare a paraffin block. Paraffin-embedded tissues were sectioned to a thickness of 4 µm. The tissue samples were stained using the M30 cytoDEATH reagent (Sigma Aldrich, USA). Apoptosis was evaluated using a mouse monoclonal antibody that detects the caspase cleavage product of Krt18 in epithelial cells (M30). Terminal deoxynucleotidyl transferase dUTP nick-end labeling (TUNEL) assay was performed using the in situ cell death detection kit TMR red (Sigma Aldrich, USA) to detect single-stranded and double-stranded DNA breaks that occur during the early stages of apoptosis.
Serum analysis
For serum analysis, the euthanized animals were subjected to thoracotomy. The blood sample collected through cardiac puncture was centrifuged at 3,000 rpm and 4 ℃ for 15 min. The serum was stored at −80 ℃ until further analysis. The levels of aspartate aminotransferase (AST), alanine aminotransferase (ALT), and bilirubin in the serum were measured using a biochemical analytical system (Hitachi-747; Hitachi, Tokyo, Japan).
Quantitative real-time polymerase chain reaction (qRT-PCR) analysis
Total RNA was extracted from the liver tissue using TRIzol reagent (Invitrogen, USA). The concentration of RNA was measured using a Nanodrop ND-2000 (Thermo Fisher Scientific Inc., USA). RNA purity was determined by measuring the A260/A280 ratio. The isolated RNA was reverse-transcribed into cDNA using SuperScript III (Invitrogen) and PrimeScript RT reagent kit (#RR037A; Takara, Japan). qRT-PCR analysis was performed in a 10-µL reaction mix using the LightCycler 480 system (Roche Diagnostics, Germany) with LightCycler480 SYBRGreen I Mastermix (Roche Diagnostics). The expression levels of target genes were normalized to those of Gapdh and expressed as fold changes. The primer sequences used in this study are shown in Table 1.
Table 1
Gene | Primer |
---|---|
Gapdh | Forward: 5'-CAG CCT CAA GAT CAT CAG-3' |
Reverse: 5'-TGT GGT CAT GAG TCC TTC-3' | |
Bax | Forward: 5'-AGA TGA ACT GGA CAG CAA TAT GGA G-3' |
Reverse: 5'-ACC CGG AAG AAG ACC TCT CG-3' | |
Bcl2 | Forward: 5'-TGT GGA TGA CTG AGT ACC TGA ACC-3' |
Reverse: 5'-TCA TTC AAC CAG ACA TGC ACC TAC-3' | |
Hnf4a | Forward: 5'-TCT GCG AAC TCC TTC TGG AT-3' |
Reverse: 5'-AGG AGC ACG TCC TTA AA-3' | |
Scd1 | Forward: 5'-GCC CAC ATG CTC CAA GAG AT-3' |
Reverse: 5'-GGC ACC GTC TTC ACC TTC TC-3' | |
Fas | Forward: 5'-CCCTTTTTGAGGAGGCCAAT-3' |
Reverse: 5'-GCTTCACGACTCCATCACGA-3' | |
Srebf1c | Forward: 5'-GAAACACTCAGCAGCCACCA-3' |
Reverse: 5'-CAAGCTTTGGACCTGGGTGT-3' | |
Bsep | Forward: 5'-GGA CAA TGA TGT GCT TGT GG-3' |
Reverse: 5'-CAC ACA AAG CCC CTA CCA GT-3' | |
Slc10a1 | Forward: 5'-GGT GCC CTA CAA AGG CAT TA-3' |
Reverse: 5'-ACA GCC ACA GAG AGG GAG AA-3' | |
Cd36 | Forward: 5'-CCT TAA AGG AAT CCC CGT GT-3' |
Reverse: 5'-TTG CCA ATG TCT AGC ACA CC-3' | |
Abcg5 | Forward: 5'-GGT GTC CTG CAT GTG TCC TA-3' |
Reverse: 5'-ATT TGC CTG TCC CAC TTC TG-3' | |
Cyp7a1 | Forward: 5'-CCG TCT ACG CAT GTT TCT CA-3' |
Reverse: 5'-GAA GGT TGC AGG AAT GGT GT-3' | |
Cyp27a1 | Forward: 5'-GAG AGT GAA TCA GGG GAC CA-3' |
Reverse: 5'-TCA GGA ATG GAG GGT TTC AG-3' | |
Star | Forward: 5'-GGG CAT ACT CAA CAA CCA GGA-3' |
Reverse: 5'-CGA AAC ACC TTG CCC ACA TC-3' | |
Cyp7b1 | Forward: 5'-TTC TCT GGG CCT CTC TAG CA-3' |
Reverse: 5'-CAG GGC TTC CAT AGC TTC AG-3' | |
Cyp46a1 | Forward: 5'-CAT GAG ACT TCT GCC AAC CA-3' |
Reverse: 5'-CTT GGA ACC GAC AAC CTC AT-3' | |
Cyp39a1 | Forward: 5'-TCA TTC TGG AAC CCT CTT GC-3' |
Reverse: 5'-CAG CCC AAA GTA CGA CCA GT-3' | |
Cyp8b1 | Forward: 5'-AGT TGC AGC GTC TCT TCC AT-3' |
Reverse: 5'-CCT TGC TCC CTC AGA AAC TG-3' |
Metabolomic assay
Liver tissue was homogenized in an extraction solvent. The homogenate was centrifuged at 14,000 rpm for 10 min. The supernatant was subjected to liquid chromatography-tandem mass spectrometry (LC/MS) analysis using a UPLC-Q-TOF MS instrument (Vion, Waters, Milford, MA, USA) equipped with an Acquity UPLC BEH C18 column. The LC conditions were as follows: solvent A, 0.1% formic acid (FA) in water; solvent B, 0.1% FA in acetonitrile (ACN); flow rate, 0.35 mL/min; injection volume, 1 µL. The MS conditions were as follows: mode, multiple reaction monitoring; capillary voltage, 3 kV; sample cone voltage, 40 V; desolvation gas flow rate, 800 L/h; cone gas flow rate, 30 L/h; desolvation temperature, 400 ℃; ion source temperature, 100 ℃; lock mass, leucine-enkephalin (556.2771 Da). Data were processed using UNIFI version 1.8.2.169 (Waters). Partial least squares-discriminant analysis (PLS-DA) and permutation test were performed, and variable importance for projection and P values were determined using SIMCA-P+ version 12.0.1, Umetrics, Sweden.
Statistical analysis
All data are expressed as mean ± standard error of mean. Statistical analysis was performed using PRISM 8.02. The experiments were performed in triplicate. The data for the standard diet-fed sham and cholecystectomy groups were compared using one-way analysis of variance, whereas the results of liver weight, liver/bodyweight ratio, serum biochemical, and qRT-PCR analyses for HF diet-fed mice study 2 were compared using the unpaired t-test. Differences were considered significant at P<0.05.
Results
Effect of cholecystectomy on the histological and clinical parameters in standard diet-fed mice
The liver tissues of mice fed on a standard diet were biopsied at months 1, 2, and 4 post-surgery. The tissue sections were subjected to H&E staining to compare the NAS between the sham and cholecystectomy groups (Figure 1A). The degree of steatosis and lobular inflammation were not significantly different between the sham and cholecystectomy groups at months 1, 2, and 4 post-surgery (Figure 1B). However, the ballooning degeneration score and NAS in the cholecystectomy group were significantly higher than those in the sham group at months 1 (P=0.007), 2 (P=0.018), and 4 (P=0.002) post-surgery. The AST, ALT, triglyceride (TG), and cholesterol levels were not significantly different between the sham and cholecystectomy groups (Figure 1C). Additionally, the histological scores of the H&E-stained sections were not significantly different between the sham and cholecystectomy groups at months 1, 2, and 4 post-surgery (Figure 1D).
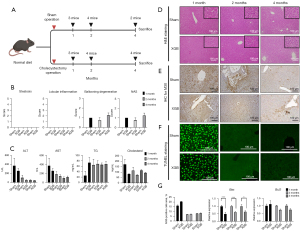
Effect of cholecystectomy on apoptosis-related markers in standard diet-fed mice
Hepatic Krt18 expression and TUNEL staining intensity (indicates the degree of DNA fragmentation) were not significantly different between the sham and cholecystectomy groups. However, hepatic Krt18 expression and the TUNEL staining intensity at month 1 post-surgery were markedly different from those at months 2 and 4 post-surgery. This indicated that apoptosis at month 1 post-surgery was mitigated at months 2 and 4 post-surgery (Figure 1E-1G, left graph). The expression of Bax, a pro-apoptotic gene, was significantly downregulated in the cholecystectomy group at months 1 (P<0.001), 2 (P<0.001), and 4 (P=0.012) post-surgery. In contrast, the expression of Bcl2, an anti-apoptotic marker, was not significantly between the sham and cholecystectomy groups (Figure 1G, middle and right panel). Primer sequences are provided in Table 1.
Effect of cholecystectomy on hepatic fat and bile acid synthesis pathways in standard diet-fed mice
The changes in the cholesterol and bile acid synthesis pathways were compared between the standard diet-fed sham and cholecystectomy groups. The expression levels of fatty acid biosynthesis-related (Srebf1c, Fas, Scd1, and Hnf4a) genes were not significantly different between the two groups. Compared with those in the sham group, the expression levels of Abcg5 (involved in bile secretion) and Cd36 (involved in the secretion of other fatty acids) were downregulated in the cholecystectomy group (Figure 2A,2B). The expression levels of classical bile acid synthesis pathway-related genes in the cholecystectomy group were lower than those in the sham group. At months 1 and 4 post-surgery, the hepatic expression levels of Cyp7a1, which encodes a rate-limiting enzyme in the acidic pathway, in the cholecystectomy group were downregulated when compared with those in the sham group (Figure 2C,2D). Additionally, the expression levels of Cyp7b1, which encodes a rate-limiting enzyme in the acidic pathway, in the cholecystectomy group were significantly lower than those in the sham group at months 1, 2, and 4 post-surgery (Figure 2C,2D). These gene primer sequences are provided in Table 1.
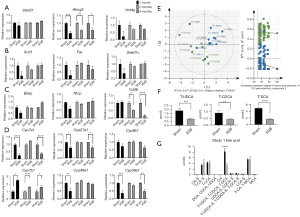
Effect of cholecystectomy on hepatic bile acid composition in standard diet-fed mice
Next, this study examined whether the changes in gene expression related to bile production affect the bile acid composition in the liver. Metabolomics analysis was performed to confirm the changes in the composition of bile acids in the liver after GB resection. PLS-DA indicated that the distribution of bile acid composition was not significantly different between the sham and cholecystectomy groups (Figure 2E,2F). The taurine-conjugated ursodeoxycholic acid (T-UDCA), taurine-conjugated chenodeoxycholic acid (T-CDCA), and taurine-conjugated deoxycholic acid (T-DCA) levels in the cholecystectomy group were significantly lower than those in the sham group (P<0.001) (Figure 2G).
Effect of cholecystectomy on the histological and clinical parameters in HF diet-fed mice
Mice fed on HF diet for 1 month to induce fatty liver were subjected to cholecystectomy. Sequential liver biopsy was performed without sacrificing the mice at months 2, 4, and 6 post-surgery (Figure 3A,3B). The liver weight, bodyweight, and liver/body weight ratio were not significantly different between the sham and cholecystectomy groups (Figure 3C). The NAS in the cholecystectomy group was significantly higher than that in the sham operation group at month 2 post-surgery (P=0.008). However, the NAS was not significantly different between the sham and cholecystectomy groups at months 3 and 6 post-surgery (Figure 3D). Additionally, the serum AST, ALT, and TG levels were not significantly different between the cholecystectomy and sham groups at the end of the study period (6 months) (Figure 3E).
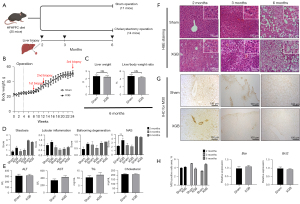
Effect of cholecystectomy on hepatic bile acid composition in HF diet-fed mice
The hepatic histological parameters were not significantly different between the sham and cholecystectomy groups at months 2, 3, and 6 post-surgery (Figure 3F). The results of the TUNEL assay revealed that apoptosis was not significantly different between the sham and cholecystectomy groups. mRNA levels of Bax and Bcl2 (apoptosis markers) were not significantly different between the sham and cholecystectomy groups (Figure 3G-3H). Stard1 (cholesterol transport marker), Abcg5 (involved in bile secretion marker) and Hnf4α (lipid homeostasis marker) genes were not significantly different between the sham and cholecystectomy groups (Figure 4A). Comparative analysis of the expression of liver fat synthesis and bile acid production-related genes (Figure 4B) revealed that Cyp7b1 expression was upregulated in the cholecystectomy group, whereas the expression of other genes was not significantly different between the two groups (Figure 4C,4D). Hepatic bile acid composition in the sham and cholecystectomy groups was evaluated at month 6 post-surgery. PLS-DA revealed that bile acid composition was not significantly different between the sham and cholecystectomy groups (Figure 4E,4F). However, T-UDCA and T-CDCA levels were significantly downregulated in the cholecystectomy group (P<0.001) (Figure 4G).
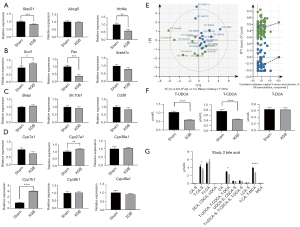
Discussion
This study investigated whether cholecystectomy could increase the incidence of fatty liver in mice.
Cholecystectomy did not induce fatty liver in standard diet-fed mice. Furthermore, cholecystectomy did not increase the risk of fatty liver or exacerbate fatty liver in HF diet-fed mice. The major strength of this study is the continuous monitoring of the development of fatty liver through liver biopsy without sacrificing mice after cholecystectomy.
A recent study showed that NAFLD could be induced by altering the intestinal microbiome profile after cholecystectomy in the standard diet-fed and HF diet-fed groups (24). However, one study involving 32,428 human subjects reported that cholecystectomy may not be a risk factor for fatty liver disease (21). Additionally, the increased prevalence of NAFLD among patients undergoing cholecystectomy was attributed to the common risk factors. Thus, cholecystectomy has no causal relationship with the development and progression of NAFLD (25). Previous reports have shown increased levels of bile acid in the early stages after cholecystectomy. However, the bile acid levels reach a steady-state at month 3 post-surgery. No marked changes in the amount and conversion rate of bile acids were observed among non-surgical patients (26). Therefore, the inconsistency of results between this study and previous studies can be attributed to different post-cholecystectomy analysis periods.
Cholecystectomy is an effective and safe treatment for GB diseases (1). Recent epidemiological studies have reported that NAFLD, dyslipidemia, and hyperglycemia are related to the occurrence of metabolic syndromes after GB resection (2,16,17,19,27,28). However, the causal relationship between cholecystectomy and metabolic disease and the underlying mechanism are unclear. Cholecystectomy does not change the bile acid pool size or composition but can increase enterohepatic circulation. Several studies have suggested that increasing bile acid exposure affects the metabolic parameters (1,17,29-31). Amigo et al. demonstrated that cholecystectomy did not affect the bile acid pool size but doubled the bile acid circulation rate (32). The authors reported that cholecystectomy increased the hepatic triglyceride content and very-low-density lipoprotein production in mice. However, the authors did not demonstrate histological changes in the liver or examine the development of fatty liver. In this study, serum triglyceride concentration transiently increased at month 1 post-cholecystectomy. However, the serum triglyceride concentrations were similar between the two groups at month 2 post-cholecystectomy. These results suggest that cholecystectomy promotes changes in the metabolic pathways but does not induce histological changes in the liver.
Several limitations were associated with this animal study. The GB resection condition in mice is different from that in humans. In humans, cholecystectomy is performed to alleviate gallstones or chronic inflammation. Thus, the pre-existing altered metabolism in humans cannot be directly compared to cholecystectomy-induced altered metabolism in mice. In study 1, the number of mice in the sham group was only 2 at month 4 post-surgery, which may affect the statistical power. However, this is a proof of study for hypothesis testing analyzing the possibility of serialization. Therefore, additional experiments are needed with a large number of animals. Furthermore, the bile acid composition of mice is significantly different from that of humans. The final product of bile acid metabolism in humans is CDCA. In contrast, CDCA is metabolized to MCA in mice. The human intestinal microbiota transforms primary bile acids (CA and CDCA) into secondary bile acids (DCA and LCA). In mice, hepatic 7α-hydroxylase transforms secondary bile acid into primary bile acid (33).
Several studies have shown that cholecystectomy regulates metabolic indicator levels by activating FXR (34). Bile acid-activated FXR increases insulin sensitivity, downregulates gluconeogenesis, and upregulates glycogen synthesis, which leads to decreased plasma glucose levels (4,35). Furthermore, FXR decreases plasma TG and cholesterol levels. However, activation of the FXR pathway by increasing the bile acid circulation rate without affecting the bile acid pool size is unknown. FXR is a master transcription factor that regulates hepatic bile acid synthesis and metabolism. Hence, this study examined the changes in the bile acid synthesis pathway. Cholecystectomy did not affect the hepatic bile acid composition but downregulated the levels of taurine-conjugated bile acids. Analysis of bile composition in the liver revealed that taurine-conjugated bile acid levels decreased in the standard diet-fed and HF diet-fed cholecystectomy groups. Previous studies have demonstrated that taurine exerts antioxidant and anti-inflammatory effects (36). Administration of taurine decreases cholesterol levels in the serum and liver and increases the fecal excretion of bile acid and taurine-conjugated bile acids (37). In this study, taurine-conjugated bile acid levels in the cholecystectomy group were lower than those in the sham operation group. Reduction of taurine-conjugated bile acids may be associated with anti-oxidative and anti-apoptotic effects. Further studies are needed to elucidate the underlying mechanisms.
CYP7A1 is a rate-limiting enzyme in the classic bile acid synthesis pathway (38). One study showed that cholecystectomy significantly upregulated the expression of Cyp7a1 in female mice without affecting the bodyweight (39). Nuclear receptors such as PXR, PPARα, and LXRα regulate Cyp7a1 expression (33). In this study, the expression levels of Cyp7a1 and Cyp27a1 were significantly downregulated at months 1 and 4 post-surgery. The underlying mechanism must be elucidated in the future.
In conclusion, this study demonstrated that the incidence of NAFLD did not increase after cholecystectomy in mice with healthy and fatty livers. Similarly, cholecystectomy did not significantly affect hepatic bile acid composition and bile acid synthesis pathways.
Acknowledgments
Funding: This study was supported by grants (No. 2020R1A2C2009227) and (No. NRF-2017R1D1A3B04033457) from the National Research Foundation of Korea.
Footnote
Reporting Checklist: The authors have completed the ARRIVE reporting checklist. Available at https://atm.amegroups.com/article/view/10.21037/atm-21-5414/rc
Data Sharing Statement: Available at https://atm.amegroups.com/article/view/10.21037/atm-21-5414/dss
Peer Review File: Available at https://atm.amegroups.com/article/view/10.21037/atm-21-5414/prf
Conflicts of Interest: All authors have completed the ICMJE uniform disclosure form (available at https://atm.amegroups.com/article/view/10.21037/atm-21-5414/coif). The authors have no conflicts of interest to declare.
Ethical Statement: The authors are accountable for all aspects of the work in ensuring that questions related to the accuracy or integrity of any part of the work are appropriately investigated and resolved. Experiments were performed under a project license (No. HY-IACUC-19-0022) granted by the Hanyang University Institutional Animal Care and Use Committee, in compliance with institutional guidelines for the care and use of animals.
Open Access Statement: This is an Open Access article distributed in accordance with the Creative Commons Attribution-NonCommercial-NoDerivs 4.0 International License (CC BY-NC-ND 4.0), which permits the non-commercial replication and distribution of the article with the strict proviso that no changes or edits are made and the original work is properly cited (including links to both the formal publication through the relevant DOI and the license). See: https://creativecommons.org/licenses/by-nc-nd/4.0/.
References
- Kullak-Ublick GA, Paumgartner G, Berr F. Long-term effects of cholecystectomy on bile acid metabolism. Hepatology 1995;21:41-5. [Crossref] [PubMed]
- Shen C, Wu X, Xu C, et al. Association of cholecystectomy with metabolic syndrome in a Chinese population. PLoS One 2014;9:e88189. [Crossref] [PubMed]
- Di Ciaula A, Garruti G, Wang DQ, et al. Cholecystectomy and risk of metabolic syndrome. Eur J Intern Med 2018;53:3-11. [Crossref] [PubMed]
- Chen Y, Wu S, Tian Y. Cholecystectomy as a risk factor of metabolic syndrome: from epidemiologic clues to biochemical mechanisms. Lab Invest 2018;98:7-14. [Crossref] [PubMed]
- Zweers SJ, Booij KA, Komuta M, et al. The human gallbladder secretes fibroblast growth factor 19 into bile: towards defining the role of fibroblast growth factor 19 in the enterobiliary tract. Hepatology 2012;55:575-83. [Crossref] [PubMed]
- Albhaisi SAM, Bajaj JS. The Influence of the Microbiome on NAFLD and NASH. Clin Liver Dis (Hoboken) 2021;17:15-8. [Crossref] [PubMed]
- Wang W, Wang J, Li J, et al. Cholecystectomy Damages Aging-Associated Intestinal Microbiota Construction. Front Microbiol 2018;9:1402. [Crossref] [PubMed]
- Ren X, Xu J, Zhang Y, et al. Bacterial Alterations in Post-Cholecystectomy Patients Are Associated With Colorectal Cancer. Front Oncol 2020;10:1418. [Crossref] [PubMed]
- Wahlström A, Sayin SI, Marschall HU, et al. Intestinal Crosstalk between Bile Acids and Microbiota and Its Impact on Host Metabolism. Cell Metab 2016;24:41-50. [Crossref] [PubMed]
- Chavez-Tapia NC, Tellez-Avila FI, Barrientos-Gutierrez T, et al. Bariatric surgery for non-alcoholic steatohepatitis in obese patients. Cochrane Database Syst Rev 2010;CD007340. [Crossref] [PubMed]
- Younossi ZM, Koenig AB, Abdelatif D, et al. Global epidemiology of nonalcoholic fatty liver disease-Meta-analytic assessment of prevalence, incidence, and outcomes. Hepatology 2016;64:73-84. [Crossref] [PubMed]
- Loomba R, Sanyal AJ. The global NAFLD epidemic. Nat Rev Gastroenterol Hepatol 2013;10:686-90. [Crossref] [PubMed]
- Ray K. NAFLD-the next global epidemic. Nat Rev Gastroenterol Hepatol 2013;10:621. [Crossref] [PubMed]
- Yuan L, Bambha K. Bile acid receptors and nonalcoholic fatty liver disease. World J Hepatol 2015;7:2811-8. [Crossref] [PubMed]
- Schaap FG, Trauner M, Jansen PL. Bile acid receptors as targets for drug development. Nat Rev Gastroenterol Hepatol 2014;11:55-67. [Crossref] [PubMed]
- Chávez-Talavera O, Tailleux A, Lefebvre P, et al. Bile Acid Control of Metabolism and Inflammation in Obesity, Type 2 Diabetes, Dyslipidemia, and Nonalcoholic Fatty Liver Disease. Gastroenterology 2017;152:1679-1694.e3. [Crossref] [PubMed]
- Ruhl CE, Everhart JE. Relationship of non-alcoholic fatty liver disease with cholecystectomy in the US population. Am J Gastroenterol 2013;108:952-8. [Crossref] [PubMed]
- Yun S, Choi D, Lee KG, et al. Cholecystectomy Causes Ultrasound Evidence of Increased Hepatic Steatosis. World J Surg 2016;40:1412-21. [Crossref] [PubMed]
- Kwak MS, Kim D, Chung GE, et al. Cholecystectomy is independently associated with nonalcoholic fatty liver disease in an Asian population. World J Gastroenterol 2015;21:6287-95. [Crossref] [PubMed]
- Ioannou GN. Cholelithiasis, cholecystectomy, and liver disease. Am J Gastroenterol 2010;105:1364-73. [Crossref] [PubMed]
- Wang HG, Wang LZ, Fu HJ, et al. Cholecystectomy does not significantly increase the risk of fatty liver disease. World J Gastroenterol 2015;21:3614-8. [Crossref] [PubMed]
- Chae YJ, Jun DW, Saeed WK, et al. Feasibility and Stability of Liver Biopsy before Treatment for Preclinical Nonalcoholic Fatty Liver Studies. J Korean Med Sci 2019;34:e14. [Crossref] [PubMed]
- Kleiner DE, Brunt EM, Van Natta M, et al. Design and validation of a histological scoring system for nonalcoholic fatty liver disease. Hepatology 2005;41:1313-21. [Crossref] [PubMed]
- Wang Q, Lu Q, Shao W, et al. Dysbiosis of gut microbiota after cholecystectomy is associated with non-alcoholic fatty liver disease in mice. FEBS Open Bio 2021; [Epub ahead of print]. [Crossref] [PubMed]
- Kakati D, Kumar U, Russ K, et al. Cholecystectomy does not worsen progression or outcomes in non-alcoholic fatty liver disease. Transl Gastroenterol Hepatol 2020;5:3. [Crossref] [PubMed]
- Berr F, Stellaard F, Pratschke E, et al. Effects of cholecystectomy on the kinetics of primary and secondary bile acids. J Clin Invest 1989;83:1541-50. [Crossref] [PubMed]
- Sonne DP, Hare KJ, Martens P, et al. Postprandial gut hormone responses and glucose metabolism in cholecystectomized patients. Am J Physiol Gastrointest Liver Physiol 2013;304:G413-9. [Crossref] [PubMed]
- Nervi F, Miquel JF, Alvarez M, et al. Gallbladder disease is associated with insulin resistance in a high risk Hispanic population. J Hepatol 2006;45:299-305. [Crossref] [PubMed]
- Shaffer EA, Small DM. Biliary lipid secretion in cholesterol gallstone disease. The effect of cholecystectomy and obesity. J Clin Invest 1977;59:828-40. [Crossref] [PubMed]
- Roda E, Aldini R, Mazzella G, et al. Enterohepatic circulation of bile acids after cholecystectomy. Gut 1978;19:640-9. [Crossref] [PubMed]
- Hylemon PB, Zhou H, Pandak WM, et al. Bile acids as regulatory molecules. J Lipid Res 2009;50:1509-20. [Crossref] [PubMed]
- Amigo L, Husche C, Zanlungo S, et al. Cholecystectomy increases hepatic triglyceride content and very-low-density lipoproteins production in mice. Liver Int 2011;31:52-64. [Crossref] [PubMed]
- Honda A, Miyazaki T, Iwamoto J, et al. Regulation of bile acid metabolism in mouse models with hydrophobic bile acid composition. J Lipid Res 2020;61:54-69. [Crossref] [PubMed]
- Rodríguez-Antonio I, López-Sánchez GN, Garrido-Camacho VY, et al. Cholecystectomy as a risk factor for non-alcoholic fatty liver disease development. HPB (Oxford) 2020;22:1513-20. [Crossref] [PubMed]
- Arab JP, Karpen SJ, Dawson PA, et al. Bile acids and nonalcoholic fatty liver disease: Molecular insights and therapeutic perspectives. Hepatology 2017;65:350-62. [Crossref] [PubMed]
- Qaradakhi T, Gadanec LK, McSweeney KR, et al. The Anti-Inflammatory Effect of Taurine on Cardiovascular Disease. Nutrients 2020;12:2847. [Crossref] [PubMed]
- Murakami S, Fujita M, Nakamura M, et al. Taurine ameliorates cholesterol metabolism by stimulating bile acid production in high-cholesterol-fed rats. Clin Exp Pharmacol Physiol 2016;43:372-8. [Crossref] [PubMed]
- Chiang JYL, Ferrell JM. Up to date on cholesterol 7 alpha-hydroxylase (CYP7A1) in bile acid synthesis. Liver Res 2020;4:47-63. [Crossref] [PubMed]
- Alexander C, Cross TL, Lee AH, et al. Development of a novel model of cholecystectomy in subsequently ovariectomized mice and characterization of metabolic and gastrointestinal phenotypes: a pilot study. BMC Gastroenterol 2021;21:62. [Crossref] [PubMed]