Effect of fecal microbiota transplantation on the TGF-β1/Smad signaling pathway in rats with TNBS-induced colitis
Introduction
Inflammatory bowel disease (IBD) is a chronic intestinal disorder, which is associated with exacerbation of the host immune response, the disruption of the intestinal barrier, and alterations in the luminal and mucosal microbial communities (1). The 2 major chronic forms of this disease are ulcerative colitis and Crohn’s disease (2). Currently, IBD remains incurable, and treatments to alleviate its effects include 5-aminosalicylic acid, steroids, antimicrobials, immunosuppressive agents, and tumor necrosis factor (TNF) blockers (3). However, the adverse side effects of these treatments and the high recurrence rates in patients taking such drugs have necessitated the development of novel therapeutic approaches (4-6).
It is well known that the development and maintenance of IBD is associated with an imbalance of the intestinal microbiota (7). Fecal microbiota transplantation (FMT), a process by which feces from a healthy donor is transferred to a patient suffering from intestinal microbial imbalance, has become an increasingly popular therapeutic option for restoring gut homeostasis in patients with IBD (8).
A previous study has shown that the transforming growth factor-beta 1 (TGF-β1)/Small mothers against decapentaplegic (Smad) signaling pathway defected involved in IBD (9). Thus, the TGF-β1/Smad signaling pathway has potential in the development of possible novel therapies for IBD (10). Additionally, FMT has been proven to be related to the TGF-β1/Smad signaling pathway. FMT significantly decreases the expression of TGF-β1 and Smad3, and increases the levels of Smad7 (11). However, the mechanisms by which FMT regulates the TGF-β1/Smad signaling pathway to alleviate the inflammatory effects of IBD remain unclear.
This study was conducted to verify the effects of FMT on IBD and obtain insights into the mechanisms involved. First, we used 2,4,6-trinitrobenzenesulfonic acid (TNBS) to experimentally induce colitis in rats. Next, we used the colitic rats to explore whether the TGF-β1/Smad signaling pathway plays a critical role in the treatment effects induced by FMT. We present the following article in accordance with the ARRIVE reporting checklist (available at https://atm.amegroups.com/article/view/10.21037/atm-22-3227/rc).
Methods
Ethics statement
This study was approved by the Animal Ethics Committee of Fuzhou Traditional Chinese Medicine Hospital (No. 2020-05-01-01). All animal care procedures were carried out in accordance with national policies for animal health and wellbeing. All the animal surgeries were performed under appropriate anesthesia to minimize the suffering of the rats.
Animals and experimental procedures
A total of 25 male Sprague-Dawley rats, weighing 200–250 g, purchased from Guangdong Medical Laboratory Animal Center (Guangzhou, China), were raised in a humidity-controlled room with free access to food and water. The temperature of the facility was maintained at 23±2 ℃ with a 12:12-light-dark cycle. The rats were randomly allocated into the following 5 groups (n=5 per group): the Normal group (blank control), the TNBS colitis group (TNBS only), the FMT group (TNBS + FMT), the Prednisone group (TNBS + prednisone), and the TGF-β1 inhibitor group (TNBS + SB431542) (see Table 1). The rats in the Normal group were used as the fecal microbiota donors for the FMT group. Fecal samples from the normal rats were collected daily at 10:00 am and pooled at equal amounts within the group. Next, 7 g of the pooled fecal samples was suspended in 15 mL of sterile 0.9% normal saline by vortex mixing. The fecal suspension was then filtered through a stainless-steel mesh and centrifuged at 6,000 ×g for 15 min, after which the supernatant was stored immediately at 4 ℃.
Table 1
Group | Day 1 | Day 2 | Day 3 | Day 4 | Day 5 | Day 6 | Day 7 | Day 8 | Day 9 | Day 10 |
---|---|---|---|---|---|---|---|---|---|---|
Blank | 0±0 | 0±0 | 0±0 | 0±0 | 0±0 | 0±0 | 0±0 | 0±0 | 0±0 | 0±0 |
TNBS | 1.20±0.73* | 1.40±0.68* | 1.00±0.38* | 1.17±0.59* | 1.17±0.59* | 1.00±0.56* | 0.83±0.67* | 0.92±0.76 | 0.67±0.56 | 0.50±0.60 |
TNBS + FMT | 1.67±0.58*# | 1.27±0.80*# | 1.17±0.60*# | 1.17±0.64* | 1.00±0.56* | 1.00±0.56* | 0.33±0.37# | 0.33±0.37 | 0.33±0.37 | 0.17±0.30 |
TNBS + Ped | 1.33±0.00* | 1.53±0.38* | 1.13±0.45* | 1.25±0.67* | 1.17±0.60* | 0.67±0.56*# | 0.67±0.56 | 0.67±0.56 | 0.42±0.47 | 0.17±0.30 |
TNBS + SB431542 | 1.73±0.72*# | 1.53±0.38*# | 1.27±0.37*# | 1.20±0.30* | 1.27±0.37* | 1.00±0.67* | 1.00±0.58* | 0.60±0.60 | 0.27±0.37 | 0.27±0.37 |
F | 10.549 | 7.532 | 12.244 | 13.300 | 13.776 | 4.328 | 3.372 | 2.139 | 1.697 | 1.031 |
P | <0.001 | 0.001 | <0.001 | <0.001 | <0.001 | 0.014 | 0.033 | 0.120 | 0.197 | 0.420 |
Blank: normal untreated rats; TNBS: colitic rats; TNBS + FMT: colitic rats treated with fecal microbiota transplantation; TNBS + Ped: colitic rats treated with prednisone; TNBS + SB431542: colitic rats treated with the TGF-β1 inhibitor SB431542. *, P<0.05 vs. the Blank group; #, P<0.05 vs. the TNBS group. DAI, disease activity index; TNBS, 2,4,6-trinitrobenzenesulfonic acid.
All the animals, except those in the Normal group, were deprived of food for 48 h before colitis modeling. The rats were then anesthetized with an intraperitoneal injection of pentobarbital sodium (30 mg/kg), and 1 mL of 30 mg/mL TNBS (Sigma-Aldrich, St. Louis, MO, USA) dissolved in ethanol (40%, v/v) was subsequently administered via intrarectal instillation. Thereafter, the rats in the FMT group were intrarectally administered 0.2 mL of the fecal supernatant at every 2 days for 10 days. At the same time, the rats in the Prednisone group, TGF-β1 inhibitor group, and TNBS colitis group were administered 0.2 mL of 5 mg/kg prednisone, 0.2 mL of 10.0 µM SB431542 (MedChemExpress, Monmouth Junction, NJ, USA), and 0.2 mL of 0.9% normal saline, respectively, via intrarectal instillation. The rats in the Normal group were left untreated and received a normal diet and water. After the 10 days of treatments, all the rats were sacrificed, and serum and intestinal tissue samples were collected and stored at −80 ℃.
Assessment of disease activity index (DAI) scores
Each rat was assigned a DAI score daily on the basis of weight loss, stool consistency, and bloody stool, as previously described (12).
Histologic analysis
The intestinal tissues were fixed in 4% formaldehyde and embedded in paraffin wax blocks. Next, 5-µm tissue sections were obtained and stained with hematoxylin-eosin-saffron (HES) to estimate the tissue damage caused by colitis. The sections were scored by the same pathologist, who was blinded to the samples. Epithelial necrosis, inflammatory cell infiltration, and the mucosa thickness were assessed using semi-quantitative scores that ranged from 0 to 3 for each variable (0, no inflammation; 1, very low level of inflammation; 2, moderate level of leukocyte infiltration; 3, high levels of leukocyte infiltration, vascular density, and ulceration). Leica QWin software (Leica Microsystems, Bensheim, Germany) was used for the image analysis (13).
Western blot analysis
The frozen intestinal tissue samples were homogenized in phosphate-buffered saline containing a 1% protease inhibitor cocktail. The homogenates were then centrifuged (12,000 ×g, 15 min, 4 ℃) and the supernatants were collected. The protein concentration in the supernatants was determined using Bradford’s colorimetric method. Next, aliquots of the supernatants containing equal amounts of protein (40 µg) were separated by sodium dodecyl sulfate-polyacrylamide gel electrophoresis, after which the protein bands on the gel were transferred to a polyvinylidene difluoride membrane. After blocking the membrane with tris buffered saline with tween containing 5% non-fat dry milk for 2 h, the membrane was cut into 5 pieces based on the size of the target proteins. The pieces were then incubated with primary antibodies [anti-TGF-β1, anti-Smad3, anti-Smad4, anti-Smad7, and anti-glyceraldehyde 3-phosphate dehydrogenase (GAPDH) rabbit polyclonal antibodies] (Hanzhou Huaan Biotechnology Co., Ltd., Hanzhou, China) at dilutions of 1:250 for 1 h at room temperature. After 3 washes with TBST, all the membranes were incubated with horseradish peroxidase-conjugated goat anti-rabbit secondary immunoglobulin G antibodies (Hanzhou Huaan Biotechnology Co., Ltd., Hanzhou, China) for 1 h at room temperature. GAPDH was used as the loading control. Immunodetection was achieved using an enhanced chemiluminescence light-detecting kit (Amersham, GE Healthcare, Chicago, IL, USA). Following their normalization to the GAPDH level, the densitometric data of the target proteins were measured using an ImageScanner II densitometer and ImageQuant TL analysis software (GE Healthcare).
Enzyme immunoassay
The rat serum concentrations of the cytokines TNF-α, interleukin (IL)-1β, IL-6 and IFN-γ were determined with the enzyme-linked immunosorbent assay using commercial kits (Chengdu Zhengneng Biotechnology Co., Ltd., Chengdu, China).
Statistical analysis
Statistical comparisons were performed using SPSS version 23.0 software (International Business Machines Corporation, USA). All the quantitative data are presented as the mean ± standard deviation. Statistical analyses were performed using a one-way analysis of variance. For non-parametric data, the Kruskal-Wallis test was performed. Differences with a P value <0.05 were considered statistically significant.
Results
The DAI and histologic scores changed in the colitic rats
Among all the groups, there were significant differences in the DAI scores from day 1 to day 7 (P<0.05), but there were no significant differences from day 8 to day 10 (P>0.05; see Table 1). On day 1, the TNBS colitis, FMT, prednisone, and TGF-β1 inhibitor groups all showed significantly higher DAI scores than the Normal group. However, the scores were significantly decreased in the respective groups after the different treatments (see Figure 1A).
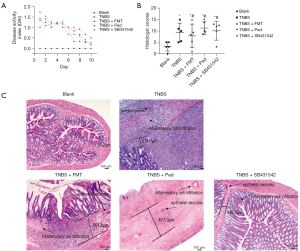
The histopathological changes in the intestinal tissues were examined using HES staining. The histologic scores for the Normal, TNBS colitis, FMT, prednisone, and TGF-β1 inhibitor groups were 3.20±2.05, 9.30±4.15, 7.90±5.16, 11.30±2.80, and 10.10±4.13, respectively (see Figure 1B,1C). There were significant differences in the histologic scores among all the groups (P<0.05).
FMT inhibited the TGF-β1/Smad signaling pathway
To investigate whether the TGF-β1/Smad signaling pathway plays an important role in the FMT alleviation of experimentally induced colitis, a Western blot analysis was conducted to detect the expression levels of these signaling proteins in the intestinal tissues. In the TNBS colitis group, the expression level of TGF-β1 was approximately 1.56-fold higher than that in the Normal group; while the expression levels of Smad3, Smad4, and Smad7 were approximately 0.62-, 0.49-, and 0.47-fold lower (see Figure 2A,2B). However, after FMT treatment, the TGF-β1 expression level decreased significantly by approximately 0.72-fold relative to the level in the TNBS colitis group, whereas the Smad3, Smad4, and Smad7 expression levels increased by approximately 1.21, 1.40, and 1.18 folds, respectively. Similarly, SB431542 inhibited the expression of TGF-β1 and promoted the expression of Smad3, Smad4, and Smad7. These data strongly suggest that FMT inhibits the TGF-β1/Smad signaling pathway to attenuate inflammation.
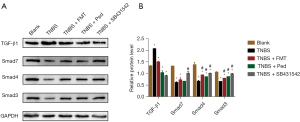
FMT affected the proinflammatory cytokines TNF-α, IL-1β, IL-6 but not IFN-γ
It has been previously reported that TNBS exposure leads to a significant increase in the levels of pro-inflammatory cytokines in intestinal tissues (14,15). In this study, the concentrations of TNF-α, IL-1β, IL-6 and IFN-γ were 6.36, 5.44, 5.23 and 6.68 times higher in the TNBS colitis group than that in the Normal group, respectively (see Figure 3). However, after the FMT, prednisone, and TGF-β1 inhibitor interventions in the colitic rats, the concentrations of proinflammatory cytokines TNF-α, IL-1β and IL-6 in the 3 respective groups were significantly decreased (see Figure 3A-3C). Notably, the difference in IFN-γ concentrations between the TNBS colitis and FMT groups was not statistically significant, suggesting that the expression of this cytokine was not affected by the FMT intervention (see Figure 3D).
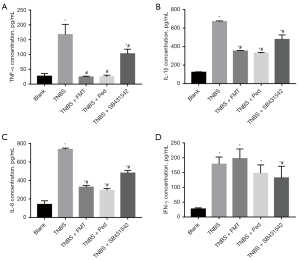
Discussion
In recent years, the therapeutic strategies for IBD have shifted away from general immunosuppressive treatment towards pathway-based approaches (16). These specific pathway-based drugs, such as anti-TNF, IL-12, and IL-23, Janus kinase inhibitors, and antisense oligonucleotides against inhibitors of the immunosuppressive cytokine TGF-β1, are fairly effective, and will increase the therapeutic options for patients with IBD (17-21). FMT has been proven to be highly successful in the treatment of recurrent and antibiotic refractory Clostridioides difficile infections, with a cure rate of up to 90% (22-24). Thus, FMT has become a promising therapeutic approach to correct IBD-related dysbiosis (25,26).
FMT has been proven to be efficacious in treating IBD; however, its mechanism of action remains unclear. Recently, several researchers have focused on studying the mechanism. For example, Zhang et al. (27) found that FMT controlled experimentally induced ulcerative colitis by improving T helper 1 (Th1)/T helper 2 (Th2) and T helper cell 17 (Th17)/regulatory cells (Treg) imbalance through the regulation of the intestinal microbiota. Another study suggested that FMT could increase the abundance of the gut bacteria that produce short-chain fatty acids, which in turn decreased proinflammatory cytokine production by suppressing the nuclear factor-kappa B signaling pathway (28). In this study, we found that both the DAI and histologic scores were significantly decreased after the FMT intervention, indicating that the transplanted fecal microbiota could effectively prevent TNBS-induced colitis. The signaling molecule TGF-β1 was downregulated, whereas Smad3 was upregulated, after FMT treatment in the colitic rats, which strongly suggests that FMT inhibits the TGF-β1/Smad signaling pathway to attenuate inflammation.
As a well-known pro-inflammatory cytokine, TNF-α induces the apoptosis of epithelial cells, disrupts the epithelial barrier, and prolongs the inflammatory reaction (29). After the FMT intervention, the concentration of TNF-α was significantly decreased in the colitic rats, which provides further evidence that this treatment inhibits the TGF-β1/Smad signaling pathway to attenuate inflammation. However, in this study, the other pro-inflammatory cytokine IFN-γ was not significantly affected by the FMT intervention.
Conclusions
In conclusion, our study verified that FMT significantly reduced the DAI and histologic scores of rats with experimentally induced colitis. Additionally, FMT was found to inhibit the TGF-β1/Smad signaling pathway to attenuate inflammation. These findings can help to optimize the clinical use of this therapeutic option for patients with IBD.
Acknowledgments
We would like to thank Zhangying Jiang (Department of Pathology, Fuzhou Traditional Chinese Medicine Hospital, Fuzhou, China) for analyzing the histologic scores.
Funding: This work was supported by the Fuzhou Science and Technology Project (grant No. 2019-SZ-33) and the Fuzhou Traditional Chinese Medicine Hospital In-House Project (grant No. 2020-Ynky-33).
Footnote
Reporting Checklist: The authors have completed the ARRIVE reporting checklist. Available at https://atm.amegroups.com/article/view/10.21037/atm-22-3227/rc
Data Sharing Statement: Available at https://atm.amegroups.com/article/view/10.21037/atm-22-3227/dss
Conflicts of Interest: All authors have completed the ICMJE uniform disclosure form (available at https://atm.amegroups.com/article/view/10.21037/atm-22-3227/coif). The authors have no conflicts of interest to declare.
Ethical Statement: The authors are accountable for all aspects of the work in ensuring that questions related to the accuracy or integrity of any part of the work are appropriately investigated and resolved. This study was approved by the Animal Ethics Committee of Fuzhou Traditional Chinese Medicine Hospital (No. 2020-05-01-01). All animal care procedures were carried out in accordance with national policies for animal health and wellbeing.
Open Access Statement: This is an Open Access article distributed in accordance with the Creative Commons Attribution-NonCommercial-NoDerivs 4.0 International License (CC BY-NC-ND 4.0), which permits the non-commercial replication and distribution of the article with the strict proviso that no changes or edits are made and the original work is properly cited (including links to both the formal publication through the relevant DOI and the license). See: https://creativecommons.org/licenses/by-nc-nd/4.0/.
References
- Garcia-Carbonell R, Yao SJ, Das S, et al. Dysregulation of Intestinal Epithelial Cell RIPK Pathways Promotes Chronic Inflammation in the IBD Gut. Front Immunol 2019;10:1094. [Crossref] [PubMed]
- Longo S, Chieppa M, Cossa LG, et al. New Insights into Inflammatory Bowel Diseases from Proteomic and Lipidomic Studies. Proteomes 2020;8:18. [Crossref] [PubMed]
- Meier J, Sturm A. Current treatment of ulcerative colitis. World J Gastroenterol 2011;17:3204-12. [PubMed]
- de Jong MC, van Dam RM, Maas M, et al. The liver-first approach for synchronous colorectal liver metastasis: a 5-year single-centre experience. HPB (Oxford) 2011;13:745-52. [Crossref] [PubMed]
- Feng S, Dai Z, Liu AB, et al. Intake of stigmasterol and β-sitosterol alters lipid metabolism and alleviates NAFLD in mice fed a high-fat western-style diet. Biochim Biophys Acta Mol Cell Biol Lipids 2018;1863:1274-84. [Crossref] [PubMed]
- Kangsamaksin T, Chaithongyot S, Wootthichairangsan C, et al. Lupeol and stigmasterol suppress tumor angiogenesis and inhibit cholangiocarcinoma growth in mice via downregulation of tumor necrosis factor-α. PLoS One 2017;12:e0189628. [Crossref] [PubMed]
- Weingarden AR, Vaughn BP. Intestinal microbiota, fecal microbiota transplantation, and inflammatory bowel disease. Gut Microbes 2017;8:238-52. [Crossref] [PubMed]
- Blanchaert C, Strubbe B, Peeters H. Fecal microbiota transplantation in ulcerative colitis. Acta Gastroenterol Belg 2019;82:519-28. [PubMed]
- Troncone E, Marafini I, Stolfi C, et al. Transforming Growth Factor-β1/Smad7 in Intestinal Immunity, Inflammation, and Cancer. Front Immunol 2018;9:1407. [Crossref] [PubMed]
- Marafini I, Zorzi F, Codazza S, et al. TGF-Beta signaling manipulation as potential therapy for IBD. Curr Drug Targets 2013;14:1400-4. [Crossref] [PubMed]
- Li B, Yin GF, Wang YL, et al. Impact of fecal microbiota transplantation on TGF-β1/Smads/ERK signaling pathway of endotoxic acute lung injury in rats. 3 Biotech 2020;10:52.
- Senol A, Isler M, Sutcu R, et al. Kefir treatment ameliorates dextran sulfate sodium-induced colitis in rats. World J Gastroenterol 2015;21:13020-9. [Crossref] [PubMed]
- Amamou A, Rouland M, Yaker L, et al. Dietary salt exacerbates intestinal fibrosis in chronic TNBS colitis via fibroblasts activation. Sci Rep 2021;11:15055. [Crossref] [PubMed]
- He J, Song Y, Li G, et al. Fbxw7 increases CCL2/7 in CX3CR1hi macrophages to promote intestinal inflammation. J Clin Invest 2019;129:3877-93. [Crossref] [PubMed]
- Gougeon PY, Lourenssen S, Han TY, et al. The pro-inflammatory cytokines IL-1β and TNFα are neurotrophic for enteric neurons. J Neurosci 2013;33:3339-51. [Crossref] [PubMed]
- Bamias G, Pizarro TT, Cominelli F. Pathway-based approaches to the treatment of inflammatory bowel disease. Transl Res 2016;167:104-15. [Crossref] [PubMed]
- Argollo M, Fiorino G, Hindryckx P, et al. Novel therapeutic targets for inflammatory bowel disease. J Autoimmun 2017;85:103-16. [Crossref] [PubMed]
- Alimohammadi N, Koosha F, Rafeian-Kopaei M. Current, New and Future Therapeutic Targets in Inflammatory Bowel Disease: A Systematic Review. Curr Pharm Des 2020;26:2668-75. [Crossref] [PubMed]
- Sukocheva OA, Lukina E, McGowan E, et al. Sphingolipids as mediators of inflammation and novel therapeutic target in inflammatory bowel disease. Adv Protein Chem Struct Biol 2020;120:123-58. [Crossref] [PubMed]
- Wehkamp J, Stange EF. Recent advances and emerging therapies in the non-surgical management of ulcerative colitis. F1000Res 2018;7:eF1000 Faculty Rev-1207.
- Atreya R, Neumann H, Neufert C, et al. In vivo imaging using fluorescent antibodies to tumor necrosis factor predicts therapeutic response in Crohn's disease. Nat Med 2014;20:313-8. [Crossref] [PubMed]
- Staley C, Hamilton MJ, Vaughn BP, et al. Successful Resolution of Recurrent Clostridium difficile Infection using Freeze-Dried, Encapsulated Fecal Microbiota; Pragmatic Cohort Study. Am J Gastroenterol 2017;112:940-7. [Crossref] [PubMed]
- Staley C, Kaiser T, Vaughn BP, et al. Durable Long-Term Bacterial Engraftment following Encapsulated Fecal Microbiota Transplantation To Treat Clostridium difficile Infection. mBio 2019;10:01586-19. [Crossref] [PubMed]
- Quraishi MN, Widlak M, Bhala N, et al. Systematic review with meta-analysis: the efficacy of faecal microbiota transplantation for the treatment of recurrent and refractory Clostridium difficile infection. Aliment Pharmacol Ther 2017;46:479-93. [Crossref] [PubMed]
- Hong Y, Zhao J, Chen YR, et al. Spinal anesthesia alleviates dextran sodium sulfate-induced colitis by modulating the gut microbiota. World J Gastroenterol 2022;28:1239-56. [Crossref] [PubMed]
- D Goldenberg S. Merrick B. The role of faecal microbiota transplantation: looking beyond Clostridioides difficile infection. Ther Adv Infect Dis 2021;8:2049936120981526. [PubMed]
- Zhang L, Ma X, Liu P, et al. Treatment and mechanism of fecal microbiota transplantation in mice with experimentally induced ulcerative colitis. Exp Biol Med (Maywood) 2021;246:1563-75. [Crossref] [PubMed]
- Zhang W, Zou G, Li B, et al. Fecal Microbiota Transplantation (FMT) Alleviates Experimental Colitis in Mice by Gut Microbiota Regulation. J Microbiol Biotechnol 2020;30:1132-41. [Crossref] [PubMed]
- Zhang Z, Xu J, Liu B, et al. Ponicidin inhibits pro-inflammatory cytokine TNF-α-induced epithelial-mesenchymal transition and metastasis of colorectal cancer cells via suppressing the AKT/GSK-3β/Snail pathway. Inflammopharmacology 2019;27:627-38. [Crossref] [PubMed]
(English Language Editor: L. Huleatt)