The role of non-coding RNAs in myocarditis: a narrative review
Introduction
Myocarditis is an inflammatory disease of the myocardium that exhibits vast clinical heterogeneity, ranging from mild chest discomfort to cardiogenic shock. Precise diagnosis of myocarditis is difficult to achieve because of the high heterogeneity of its clinical presentation and the scarcity of endomyocardial biopsy (EMB) (1). To date, no specific blood test has been established to reliably diagnose myocarditis. Patients with mild myocarditis often recover without any treatment (2). However, in some cases, myocarditis progresses to dilated cardiomyopathy (DCM) and heart failure requiring heart transplantation, even after the use of major treatment methods, such as immunosuppressant, antiviral, and circulatory support (3,4). Therefore, exploration of novel diagnostic and therapeutic methods for myocarditis is important.
Non-coding RNAs (ncRNAs) are ribonucleic acids transcribed from DNA that are not translated into proteins. They include microRNAs (miRNAs), long non-coding RNAs (lncRNAs), circular RNAs (circRNAs), transfer RNAs, ribosomal RNAs, small nuclear RNAs, and piwi-interacting RNAs (5). Mounting evidence suggests that ncRNAs play a pivotal role in cardiovascular and inflammatory diseases (6-9). Therefore, future therapies may focus on these ncRNAs to reduce the severity of myocarditis. Furthermore, considering that some ncRNAs in blood are stable to degradation, have long half-lives, and can be measured by classical laboratory methods, they also hold great potential for the diagnosis of myocarditis. In this review, we focus on the roles of miRNAs, lncRNAs, and circRNAs in the pathogenesis of myocarditis and discuss the possibility of using ncRNAs as novel diagnostic markers and therapeutic targets for myocarditis. We present the following article in accordance with the Narrative Review reporting checklist (available at https://atm.amegroups.com/article/view/10.21037/atm-21-6116/rc).
Methods
Literature on ncRNAs and myocarditis published in PubMed up to September 2021 was extensively reviewed and analyzed using the following keywords: “non-coding RNAs and myocarditis”, “miRNAs and myocarditis”, “lncRNAs and myocarditis”, and “circRNAs and myocarditis”. The search strategy is summarized in Table 1.
Table 1
Items | Specification |
---|---|
Date of search | 14/9/2021 |
Databases and other sources searched | PubMed database |
Search terms used | “non-coding RNAs and myocarditis”, “miRNAs and myocarditis”, “lncRNAs and myocarditis”, “circRNAs and myocarditis |
Time frame | From December, 2010 to September, 2021 |
Inclusion and exclusion criteria | Published English-language literature on the role of non-coding RNAs in myocarditis. All study types were included |
Selection process | Wenhu Liu and Jing Hu searched the database independently. The corresponding author mediated any cases of disagreement between the two researchers |
Results
Animal models of myocarditis
Myocarditis is a cardiovascular disease caused by a variety of factors, most of which are associated with infections, immunity, drugs, and toxins (4,10,11). Myocarditis has been understood mainly through animal models, and several clinically relevant models have been developed over the years. Animal models of myocarditis with different etiologies are summarized in Table 2 (12).
Table 2
Model | Susceptible strains | Disease features | Advantages | Disadvantages |
---|---|---|---|---|
CVB3-induced myocarditis | A/J, BALB/c, ABY/SNJ | Acute myocarditis progressing to DCM | Recapitulates clinical pathogenesis of myocarditis caused by viruses | High-grade virus lab required; severe pancreatitis with clinical inconsistency; high mortality rate |
C57 BL/6 | Acute myocarditis | |||
Experimental autoimmune myocarditis | A/J, BALB/c, SW | Acute myocarditis progressing to DCM | High biosecurity, recapitulates virus-negative myocarditis; study of the progression to DCM | Non-clinical natural causes of morbidity |
Trypanosoma cruzi-induced myocarditis | BALB/c, C57BL/6, A/J, DBA-2, C3H/He | Chronic myocarditis | Recapitulates clinical pathogenesis of Chagas disease-induced myocarditis | Classical models for which there is no definitive model; a long time required to reach the chronic phase |
ICI-induced myocarditis | MRL, BALB/C, PD1-/- or PDL1-/- mice | Fulminant myocarditis or DCM | Study of ICI-induced myocarditis | High mortality rate; strict mouse strain restrictions |
BALB/c, C57BL/6, CTLA4-/- mice | Fulminant myocarditis | High mortality rate; systemic non-specific lymphoid proliferative disease with non-specific myocarditis | ||
C57BL/6, PD1-lg treatment, and cardiac radiation | Fulminant myocarditis | High mortality rate; the influence of radiation factors |
DCM, dilated cardiomyopathy; IPSCs, induced pluripotent stem cells; ICI, immune checkpoint inhibitor.
Coxsackievirus B3-induced myocarditis models
The most common animal model of viral myocarditis is the coxsackievirus B3 (CVB3)-induced myocarditis mouse model, which was first established in the 1950s (13,14). The CVB3-infected mouse model remains the animal model of choice for studying viral myocarditis because it closely resembles the heart injury observed in patients with myocarditis (15). The model is developed by intraperitoneal injection of mice with purified CVB3 viruses, which cause acute cardiac inflammation between days 7 and 14 after infection (16). Susceptible mouse strains, including A/J and BALB/c mice, develop chronic myocarditis; in contrast, C57 mice are resistant and develop only acute myocarditis (17). Due to the ability of the virus to induce multiorgan dysfunction, mice often also develop pancreatitis (18). Although pancreatitis may influence cardiotoxicity, this concept has been challenged by experiments in mice with pancreas-specific deletion of the coxsackievirus and adenovirus receptor, which showed that infection of the pancreas has very little effect on cardiac CVB3 infection and pathology (19). However, pancreatitis is rare in patients with myocarditis, and the high frequency of pancreatitis is a key limitation of this mouse model (20).
Experimental autoimmune myocarditis (EAM) models
Myocarditis has been linked to autoimmunity (21,22). Circulating heart-specific autoantibodies are often detected in patients with myocarditis or inflammatory cardiomyopathy (23). These cardiac-specific autoantibodies can recognize many cardiac antigens, particularly cardiac alpha-myosin heavy chain and beta-myosin heavy chain (24,25). EAM can be induced by subcutaneous injection of mice with cardiac myosin with adjuvant on days 0 and 7. In these mice, cardiac inflammation is evident at 21 days after injection, and DCM usually develops within 40 days after injection (26,27). The injection of cardiac myosin induces severe myocarditis and the production of high titers of anti-myosin autoantibodies in A/J, Swiss Webster, and BALB/c mice. In contrast, C57BL/6J and C57BL/10J mice are resistant to cardiac myosin-induced myocarditis and do not develop myosin-specific autoantibodies (28). A key advantage of this model is that it allows both autoimmunity and the transition from myocarditis to DCM to be studied. However, most transgenic and knockout mice have a C57 background, which limits the wide application of the EAM model (12).
Trypanosoma cruzi-induced myocarditis models
Trypanosoma cruzi (T. cruzi) infection, also known as Chagas disease, may cause myocarditis. Experimental Chagas heart disease has been successfully established in several mouse lines using various strains of T. cruzi, including the Colombian, Tulahuen, CL Brener, Y, and Sylvio X10 strains. However, no model has been generally accepted as the classical one because of the diversity of pathogenic Trypanosoma strains isolated from chagastic patients, insect vectors, and animal hosts (12,29,30).
Myocarditis models induced by immune checkpoint inhibitors (ICIs)
ICIs have become the treatment of choice for many cancers. Myocarditis induced by ICI therapy is rare but can have serious consequences (31,32). Cytotoxic T lymphocyte-associated protein 4 (CTLA-4) and programmed cell death protein 1 (PD-1) are the most common targets in animal models of ICI-induced myocarditis (33). In view of the extremely low incidence of myocarditis induced through the direct use of ICIs, the current animal models mostly adopt strategies targeting immune sites, in particular, the use of knockout mice for PD-1 and CTLA-4. Tivol et al. reported that CTLA-4-/- mice rapidly develop lymphoproliferative disease with multiorgan lymphocyte infiltration and tissue destruction, as well as severe myocarditis and pancreatitis (34). Similarly, PD-1-/-, PD-L1-/-, BALB/c, and MRL mice develop myocarditis and DCM (35-37). Du et al. (38) developed a technique to deliver cardiac irradiation in a mouse model while using PD-1 blockade, and histological examination of their model revealed significant inflammation in the heart.
The role of ncRNAs in myocarditis
Classification of ncRNAs
At present, the mechanism of myocarditis is still unclear. Myocarditis is believed to be caused by the damaging effects of factors that activate the immune system (39), and the role immune-induced myocardial injury plays in inducing myocarditis is becoming increasingly evident (10,40). With the completion of the Human Genome Project and the development of next-generation sequencing technologies, thousands of ncRNAs have been identified, some of which appear to be important regulators of the initiation and progression of myocarditis (41,42).
miRNAs are the most abundant ncRNAs and have lengths ranging between 18 and 25 nucleotides (43). They are generated in the nucleus from primary miRNAs (pri-miRNAs), which are sheared by the intranuclear nucleic acid endonuclease Drosha into miRNA precursors (pre-miRNAs). Pre-miRNAs are translocated into the cytoplasm, where they are cleaved by Dicer to produce mature miRNAs (44,45). Often, a single pre-miRNA gives rise to multiple mature miRNAs, which can bind to the 3' untranslated region of one or more mRNA molecules. Upon miRNA-mRNA binding, the RNA-induced silencing complex suppresses the expression of the target gene (46,47). Many studies have shown that miRNAs can regulate myocarditis by modulating the expression of target genes.
Long ncRNAs comprise a class of ncRNAs with a length exceeding 200 nucleotides (48). Although not highly conserved in their sequence, most lncRNAs have a conserved secondary structure and subcellular localization, both of which are critical to their function (49). Unlike that of miRNAs, the mechanism of action of lncRNAs is poorly understood. Long ncRNAs can bind to other nucleic acids (e.g., DNA, mRNA, and miRNA) or certain proteins to participate in epigenetic, transcriptional, and post-transcriptional regulation of gene expression (50). A class of lncRNAs known as competing endogenous RNAs (ceRNAs) can act as molecular sponges for miRNAs, thereby antagonizing their molecular effects (51).
Covalently closed single-stranded circRNAs were first discovered as a viroid form of nucleic acid (52). Circular RNAs have no 5' cap or 3' poly(A) tail and are insensitive to nucleases, making them more stable than ordinary linear RNAs (53). Although many circRNAs have been identified, the mechanisms underlying their maturation and function, as well as their role in cardiovascular diseases, are poorly understood (54). The mechanisms of action of circRNAs are believed to fall into three main categories: ceRNA or miRNA sponges, translation into short polypeptides, and circRNA-protein interactions (55).
The role of ncRNAs in viral infection-induced myocarditis
Viral myocarditis is the most extensively studied form of myocarditis. The onset and progression of this disease are determined by the interaction between the virus and the host (56). The pathogenic mechanisms of viruses in myocarditis include the direct effects of the virus on the host cells and the immunopathological damage caused by the infection (57). Critically, many mechanisms underlying viral myocarditis have been described in animal models (58). Parvovirus B19 is commonly found in human myocarditis biopsies; however, an animal model of parvovirus B19-induced myocarditis has yet to be established. In contrast, animal models of CVB3-induced myocarditis are widely used (10,59). Multiple events in the pathogenesis of CVB3-induced myocarditis are regulated by ncRNAs (Figure 1).
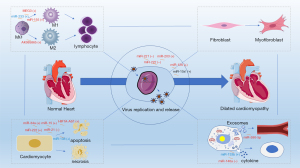
Many ncRNAs can directly regulate the apoptotic process in infected cardiomyocytes. In the early stage of viral infection, cells initiate the process of apoptosis to prevent viral invasion. The death of infected cells eliminates the environment needed for pathogen replication, which interrupts the viral replication process (60). However, in the later stages of viral proliferation, the release of progeny viruses can be promoted by triggering apoptosis, thereby accelerating the infection of nearby living cells (61). Current research on ncRNA in viral myocarditis focuses on the negative aspects of apoptosis. For instance, miR-222 is upregulated in CVB3-infected murine hearts and H9c2 cells; it regulates cardiomyocyte apoptosis and viability by modulating the expression of phosphatase and tensin homolog (PTEN) (62). PTEN is a 3'-lipid phosphatase which exerts a wide range of cardiac regulatory effects, mainly through the PI3K/Akt pathway (63). It regulates cell survival, cardiac hypertrophy, myocardial contractility, coronary angiogenesis, metabolism, and mechanotransduction (64). In cardiomyocytes, miR-34α promotes CVB3-induced apoptosis by regulating the expression of the silent information regulator T1 (SIRT1)-p53 pathway (65). Similar roles have been described for miR-15 with its targeting of nucleotide-binding oligomerization domain (NOD)-like receptor X1 (NLRX1) (66). Previous studies have showed that NLRX1 is an anti-apoptotic protein (67,68). The lncRNA hypoxia-inducible factor 1 alpha-antisense RNA 1 (HIF1A-AS1) binds to miR-138, which acts as a bridge in various ceRNA networks to promote apoptosis (69,70), and represses its expression, thereby modulating the nuclear factor kappa B (NF-κB) pathway. Reduction of the expression of lncRNA HIF1A-AS1 by siRNA was found to attenuate CVB3-induced oxidative stress and apoptosis in cardiomyocytes, and alleviate CVB3-induced myocardial inflammation (71). However, other ncRNAs can inhibit apoptosis. For example, upregulation of miR-21 expression followed by suppression of the MAP2K3/P38 signaling causes apoptosis evasion and attenuates cardiac injury after CVB3 infection (72,73). These observations show that ncRNAs can facilitate virus release and spread by regulating host cell apoptosis.
ncRNAs also regulate immune responses after CVB3 infection. CVB3 infection promotes immune damage in various cells in the heart. Given that cytokines, including interleukin (IL)-1, IL-2, IL-6, tumor necrosis factor (TNF)-α, and interferon (IFN)-γ, are elevated before inflammatory cell infiltration, cells intrinsic to the myocardium, such as cardiomyocytes and fibroblasts as well as innate immune cells, are thought to play a central role in their expression (74). Zhang et al. reported that overexpression of miR-133b in cardiomyocytes inhibited cardiomyocyte injury after viral infection, and suppressed the synthesis and release of the proinflammatory cytokines TNF-α and IL-6 (75). Another study found that miR-146a was upregulated in CVB3-infected fibroblasts and exerted anti-inflammatory effects through toll-like receptor 3 (TLR3) and TNF receptor-associated factor 6 (TRAF6) (76). In the subsequent stage, innate immune-related cells are recruited to the myocardium, where monocytes differentiate into macrophages after reaching the tissues. Of note, macrophages are central regulators of inflammatory diseases through their ability to orchestrate immunological networks. Polarization of macrophages toward the M1 (proinflammatory) or M2 (anti-inflammatory) phenotype is also a key factor regulating cardiac inflammation (77). Upregulation of lncRNA AK085865 has been observed in CVB3-infected C57 mouse hearts and murine bone marrow-derived macrophages after M2 polarization. The lncRNA AK085865 binds to IL enhancer-binding factor 2 (ILF2), thereby regulating the ILF2/ILF3 complex and subsequently, miR-192/IL-1 receptor-associated kinase 1 (IRAK1) function (78,79). IRAK1 is the main mediator of toll-like receptor 4 (TLR4) activation and binds to TRAF6, ultimately leading to the induction of NF-κB, which is essential for lipopolysaccharide-mediated inflammatory response in macrophages (80-82). Xue et al. found that silencing of the lncRNA MEG3 inhibited M1 polarization and promoted M2 polarization in macrophages, alleviating myocarditis. MEG3 regulates the expression of TRAF6 by inhibiting miR-223 (83). TRAF6—first identified as a mediator of IL-1 receptor-mediated activation of NF-κB—is critical for activating various immune cells, including macrophages (84,85). miR-223 can also regulate macrophage polarization and function, consequently affecting the progression of viral myocarditis (86). The role of miR-155 in inflammatory diseases has been extensively studied, and this miRNA has been proposed as a marker of inflammation (87). In Corsten et al.’s study, silencing of miR-155 in mice with viral myocarditis attenuated the infiltration of monocytes and macrophages in the heart by repressing the expression of the direct miR-155 target PU.1 (88). With the infiltration and activation of innate immune cells, acquired immune-related cells (especially T cells) are activated to produce cytokines and inflammatory factors (11). Nevertheless, there is a limited number of studies on this aspect. To sum up, regulating ncRNA expression can promote an inflammatory response mediated by immune cells or non-immune cells in CVB3 infection.
Further, ncRNAs can regulate CVB3 replication. A miRNA microarray analysis of hearts from mice infected with CVB3 revealed that miR-203 was one of the most upregulated genes in the infected hearts. miR-203 enhances the replication of CVB3 and the survival of infected HeLa cells by regulating the expression of zinc finger protein-148 (ZFP-148) (89). Silencing of ZFP-148 by siRNA has shown similar effects on CVB3 replication (89). miR-126 promotes CVB3 replication by targeting sprouty-related EVH1 domain-containing protein 1 (SPRED1) (90), which can regulate Ras-MAPK signaling by effectively inhibiting growth factor-mediated extracellular signal-regulated protein kinase (ERK) activation (91). Research has shown that the ERK1/2 signaling pathway is one of the important pillars of CVB3 infection (92). Also, in Ye et al.’s study, miR-126 sensitized cells to CVB3-induced cell death and increased viral progeny release to initiate new infections by targeting Wnt-responsive CDC42 homolog 1 (WRCH1) and low-density lipoprotein receptor-related protein 6 (LRP6), as well as by promoting β-catenin degradation (90). Corsten et al. reported that inhibition of miR-221/-222 increased cardiac injury and immune cell infiltration during acute viral myocarditis in vivo and increased viral replication on days 4 and 7 post infection in vitro and in vivo. Targets of miR-221/-222 include proinflammatory transcription factors (e.g., ETS1 and ETS2), IFN regulatory factor 2 (IRF2), CXC chemokines (e.g., CXCL12), anti-apoptotic proteins (e.g., BCL2L11), proapoptotic proteins (e.g., BMF), and T-cell thymocyte selection-associated HMG box (TOX) (93). All of these target genes have been implicated in playing roles in myocarditis (94-98). Interestingly, miRNAs can also directly regulate several viral functions. For instance, miR-10a* regulates the expression of the three dimensional-coding region of the CVB3 genome and enhances CVB3 replication (99). Further, high expression of miR-590-5p confirmed to be associated with viral replication was found in exosomes produced after viral infection of cells (100). However, the functional mechanism of this virus-mediated exosome release needs to be further explored. These findings confirmed that the expression of ncRNAs could promote viral replication and aggravate the severity of myocarditis in CVB3-induced myocarditis.
In conclusion, the above-discussed studies suggest that ncRNA can regulate the occurrence and development of viral myocarditis from the aspects of viral replication, immune response, and apoptosis, and provide promising data on ncRNAs as effective options for therapeutic strategies in myocarditis. However, considering the complexity of ncRNA involvement in the pathogenesis of viral myocarditis, as well as the limited number of researchers and funding for myocarditis, the number of studies in this area is few. Moreover, the research methods and experimental technologies lag behind those of many other diseases and are often unable to keep up with the speed of technological developments relating to ncRNAs. Therefore, the literature on myocarditis and ncRNAs is still lacking, and more studies need to be conducted to evaluate the role of ncRNAs in viral myocarditis.
The role of ncRNAs in EAM
As mentioned above, since the EAM model is mainly used to study autoimmunity in the heart, its primary concern is the function and mechanism of immune cells (10). Several studies have shown that ncRNAs can influence the progression of autoimmune myocarditis by regulating the activity of immune cells (Table 3). In this model, dendritic cells (DCs) attenuate myocardial injury by blocking the antigen presentation process. DCs loaded with an immunogenic peptide derived from cardiac α-myosin heavy chain activate self-reactive CD4+ T cells, leading to the dysregulation of T helper cells and regulatory T cells, which are critical for the induction of EAM (108). In Wu et al.’s study, in vivo transfer of DCs overexpressing metastasis-associated lung adenocarcinoma transcript 1 (MALAT1) delayed the progression of autoimmune myocarditis, and MALAT1 promoted DC-SIGN expression by acting as a miR-155-5p sponge in the cytoplasm of DCs (107). As a member of the C-type lectin receptor (CLR) family, DC-SIGN has been proved to play an important role in mediating DC adhesion, migration, inflammation, activating primary T cells, triggering immune response, and participating in the immune escape of pathogens and tumor cells (109). In another study, circSnx5 was found to play a role in DC-driven immunity and tolerance by inhibiting the activation and enhancing the tolerogenic functions of DCs. Importantly, circSnx5 knockdown promoted DC activation and inflammation. Mechanistically, circSnx5 can act as a miR-544 sponge to attenuate miRNA-mediated inhibition of SOCS1 expression and inhibit nuclear translocation of PU.1 (106). Previous studies had demonstrated that SOCS1 and PU.1 are pivotal regulators in DC function (110,111).
Table 3
Name | Organ | Expression | Description | Mechanism | Reference |
---|---|---|---|---|---|
miR-590-3p | Heart | Downregulation | Overexpression of miR-590-3P improves cardiac function | Target genes: NF-KB | (101) |
miR-141-3p | Heart | Downregulation | miR-141-3p inhibits the expression of inflammation-related factors in the heart | Target genes: STAT4 | (102) |
miR-21a-5p | Heart | Upregulation | Silencing miR-21a-5p reduces cardiac inflammation and fibrosis | Unknown | (103) |
miR-155 | Heart, splenic CD4+ cells | Upregulation | miR155 promotes Th17 cell development and function | Unknown | (27) |
miR-142 | Circulating exosomes | Upregulation | miR-142 regulates immunometabolism in CD4+ T cells | Target genes: MBD2, SOCS1 | (104) |
miR-98 | B cells in the heart | Upregulation | Blocking miR-98 reduces myocarditis | Unknown | (105) |
CircSnx5 | DC stimulated with lipopolysaccharide | Upregulation | Transplantation of circSnx5-overexpressing DCs attenuates experimental autoimmune myocarditis | CeRNA: CircSnx5-miR-544-SOCS1/PU.1 | (106) |
MALAT1 | DC stimulated with lipopolysaccharide | Upregulation | In vivo transfer of DCs overexpressing MALAT1 delays the progression of myocarditis | CeRNA: MALAT1-miR155-5p-DC-SIGN | (107) |
NF-KB, nuclear factor kappa B; STAT4, signal transducer and activator of transcription 4; MBD2, methyl-binding domain protein 2; SOCS1, suppressor of cytokine signaling 1; CircSnx5, cirRNA circSnx5; Snx5, sorting nexin 5; ceRNA, competing endogenous RNA; PU.1, transcription factor PU.1; MALAT1, lncRNA metastasis-associated lung adenocarcinoma transcript 1; DC-SIGN, dendritic cell-specific ICAM (intercellular adhesion molecule)-grabbing non-integrin.
The role of B cells in myocarditis is poorly understood except in one aspect. Autoantibodies are known to be a key factor in B-cell production and to contribute to the pathogenesis of myocarditis (112). In Chen et al.’s study, mice immunized with alpha-myosin heavy chain (MyHC-α) showed a reduced frequency of IL-10+ B cells in the heart. B cells isolated from the hearts of mice with myocarditis showed higher expression of miR-98 and lower levels of IL-10. Exposure to TNF-α upregulated miR-98, and miR-98 overexpression inhibited IL-10 expression in B cells. Blockade of miR-98 or transplantation of B10 cells prevented experimental myocarditis (105).
Evidence suggests that CD4+ cells play a central role in autoimmune diseases, although non-pathogenic IL-10+ Th17 cells have also been described (113,114). In mice with EAM, miR-155 was found to be upregulated and to regulate the balance of Th17/Treg cells. miR-155 also promoted the differentiation of CD4+ cells into Th17 cells and the production of Th17-stimulating cytokines in DCs (27). Additionally, in Sun et al.’s study, the injection of serum exosomes from mice with EAM induced global CD4+ T cell activation and cardiac inflammation. Mechanistically, circulating exosomes from mice with EAM were selectively loaded with abundant miR-142, which was confirmed by luciferase assays to regulate CD4+ T cell dysfunction and glycolytic reprogramming by targeting methyl-CpG binding domain protein 2 (MBD2) and suppressor of cytokine signaling 1 (SOCS1) (104). Further, other studies have reported that miR-590-3p and miR-141-3p can also play a role in EAM by reducing cardiac inflammation; however, more detailed mechanisms have yet to be elucidated (101,102).
ncRNA replacement therapy has been hampered for a long time due to the lack of robust nonviral delivery methods for in vivo administration (115). To overcome this challenge, several explorations have been undertaken. For example, systemic treatment consisting of antagomiR-21a-5p formulated with polymeric nanoparticles based on low molecular weight polyethyleneimine was found to significantly reduce myocardial inflammation and fibrosis (103). This study suggests that ncRNAs may provide new targets for treating autoimmune myocarditis. However, most studies on ncRNAs in the EAM model have focused on the replacement therapy of ncRNAs, such as DC adoptive transfer or exosome therapy. There is a lack of research on the mechanism of ncRNAs in EAM, especially on Th17 cells, as the key cells leading to EAM, and further research is needed to understand the specific mechanisms of the therapeutic effects of ncRNAs.
The role of ncRNAs in myocarditis caused by T. cruzi infection
Although the function of ncRNAs in the hearts of trypanosome-infected individuals is not well understood, some studies have suggested that ncRNAs may play a role in T. cruzi-induced myocarditis. A genome-wide mRNA and miRNA analysis demonstrated that some differentially expressed miRNAs in the hearts of mice infected with T. cruzi type I were significantly associated with clinically relevant parameters of parasitemia and electrocardiographic changes (116,117). Bioinformatics analysis to integrate the overall miRNA and mRNA expression profiles of myocardial tissues from patients with chronic Chagas disease cardiomyopathy showed that miRNAs modulated the expression of multiple genes that regulate many pathophysiological processes of chronic Chagas disease cardiomyopathy. These miRNAs also regulated the expression of genes associated with cardiomyopathy-related processes, including fibrosis, hypertrophy, myocarditis, and arrhythmias (118). In a cohort of patients with chronic Chagas disease cardiomyopathy, the miRNAs miR-19a-3p, miR-21-5p, and miR-29b-3p were found to be upregulated. Receiver operating characteristic (ROC) analysis was performed to evaluate the sensitivity, specificity, and accuracy of these miRNAs; the areas under the ROC curves for miR-19a-3p, miR-21-5p, and miR-29b-3p were 0.77, 0.54 and 0.70, respectively. Moreover, these miRNAs were positively correlated with cardiac dysfunction and fibrosis, and negatively correlated with ejection fraction and left ventricular strain (119). These findings suggest that ncRNAs play an important role in trypanosome-induced myocarditis. Still, most studies are focused on the differential expression of these ncRNAs discovered by sequencing or correlation analysis with clinical practice. The roles and mechanisms of these ncRNAs in the T. cruzi-induced model are still unknown and need to be further studied.
The role of ncRNAs in myocarditis caused by ICIs
Immune checkpoint inhibitors have become the treatment of choice for many cancers. However, ICIs may cause adverse cardiovascular effects, including different forms of cardiotoxicity, such as myocarditis, acute coronary syndrome, and proximal membrane disease (120,121). However, the exact mechanisms by which ICIs cause myocarditis are still unclear, and studying ncRNAs may provide new insights. PD-1 inhibitors induce M1 macrophage polarization and myocardial inflammation by modulating the miR-34a/Krüppel-like factor 4 (KLF4) signaling pathway (122). Notably, miR-34a was found to be highly expressed in macrophage-derived exosomes treated with PD-1 inhibitors, with treatment of cardiomyocytes with these exosomes triggering cardiomyocyte senescence (123). Most studies have focused on the efficacy of ncRNAs in patients with ICI-treated tumors (124-127), and the concomitant cardiac damage has been poorly studied. Further, although a few reports have suggested that modulation of ncRNAs in vivo has a beneficial effect in combating ICI-induced cardiotoxicity, none of them used clinically relevant models. This situation highlights the need for new studies to evaluate the therapeutic effect of ncRNAs in models of ICI-induced cardiotoxicity.
The potential of ncRNAs in the diagnosis and treatment of myocarditis
The potential of ncRNAs in the treatment of myocarditis
As mentioned above, ncRNAs can be involved in regulating multiple links in the occurrence and development of myocarditis, such as apoptosis of cardiomyocytes, replication of viruses, and regulation of immune cells. Therefore, ncRNAs may serve as potential therapeutic targets for myocarditis. At present, miRNAs are the most studied ncRNAs, and mimics or inhibitors of miRNAs can be designed to reduce myocardial inflammation and damage. Clinical trials of miRNA-based therapies are already underway for other diseases, including mesothelioma, T-cell leukemia/lymphoma, and hepatitis C infection (128). Therefore, these therapies may become reliable treatments for myocarditis in the future.
Therapeutics based on ncRNAs may hold promise for the treatment of myocarditis, but there are currently some issues that must be addressed. First, one ncRNA may target multiple molecules simultaneously, which leads to the possibility that some ncRNAs may exert opposite or unpredictable effects. Second, a major barrier to implementing miRNA-based therapies in clinical practice is the lack of efficient delivery systems that can protect RNA molecules from nuclease degradation and deliver them to cardiac tissue without adverse effects. Encouragingly, delivery systems based on lipid and lipid-like materials, polymers, conjugates, and 3D scaffolds have been developed to address this problem (129). Moreover, exosomes containing ncRNAs may serve as natural medicines for myocarditis. Third, ncRNAs are poorly conserved, and there are considerable differences between mouse body structures and those of humans. Consequently, ncRNAs with functions in mice may not work in humans. Therefore, there is a long way to go before ncRNA-based therapy can become a clinical application.
The potential of ncRNAs in the diagnosis and prognosis of myocarditis
For patients with acute myocarditis, the typical symptoms and signs at presentation include chest pain, dyspnea, fatigue, palpitations, syncope, and cardiogenic shock, but these are still non-specific (130). Electrocardiograms, laboratory tests, echocardiography, and cardiac magnetic resonance have low predictive value in myocarditis, and EMB—the gold standard for myocarditis diagnosis—is not a routine practice due to its invasive nature and potential complications (131,132). Moreover, available biomarkers, such as troponins and N-terminal pro-brain natriuretic peptide (NT-proBNP), lack sufficient specificity and are widely changed during myocardial injury and cardiac dysfunction (133). Therefore, novel biomarkers with higher sensitivity and specificity in diagnosing myocarditis are urgently needed.
Several studies have been conducted to assess the value of ncRNAs in diagnosing viral myocarditis (Table 4). Studies of cardiac tissues from patients with viral myocarditis found that miR-146b, miR-214, miR-155, and miR-148a were significantly upregulated, which suggests their potential as diagnostic markers, but corresponding clinical analysis is lacking (134,135). Noninvasive diagnostic methods for myocarditis are also lacking. Circulating ncRNAs hold great potential as diagnostic biomarkers of myocarditis due to their presence in the blood (142,143). For example, miR-721, a miRNA produced by TH17 cells, was found to be increased in the plasma of patients and mice with myocarditis but not in those with myocardial infarction, suggesting its potential use as a diagnostic marker for acute myocarditis (136). In another study of 23 patients with acute myocarditis, five miRNAs (miR-30a, miR-181d, miR-125a, miR-155, and miR-21) were found to be differentially expressed in serum exosomes, but insufficient clinical information limited their use as biomarkers for the diagnosis of viral myocarditis (137).
Table 4
Name | Organ | Expression | Sample size | Biomarker type | Reference |
---|---|---|---|---|---|
miR-146b, miR-214 | Heart | Upregulation | VM/CON: 8/8 | Diagnostic | (134) |
miR-155, miR-148a | Heart | Upregulation | VM/CON: 6/6 | Diagnostic | (135) |
miR-721 | Peripheral blood | Upregulation | M/MI/CON: 42/90/80 | Diagnostic | (136) |
miR-30a, miR-181d, miR-125a | Circulating exosomes | Upregulation | VM/CON: 23/12 | Diagnostic | (137) |
miR-155, miR-21 | Circulating exosomes | Downregulation | VM/CON: 23/12 | Diagnostic | (137) |
miR-155, miR-320a | Circulating exosomes | Upregulation | FM/NFM/CON: 99/32/105 | Diagnostic | (138) |
miR-4763-3p | Peripheral blood | Upregulation | FM/CON: 20/10 | Diagnostic | (139) |
miR-155, miR-133a | Heart | Upregulation | iCMP/non-inflammatory DCM: 76/22 | Prognostic | (140) |
miR-208a | Peripheral blood | Upregulation | M acute phase/M chronic phase: 8/8 | Prognostic | (141) |
VM, viral myocarditis; CON, control; iCMP, inflammatory cardiomyopathy; DCM, dilated cardiomyopathy; M, myocarditis; MI, myocardial infarction; FM, fulminant myocarditis; NFM, non-fulminant myocarditis.
Fulminant myocarditis is a rare and serious form of myocarditis that presents as a severe and sudden attack, with symptoms typically lasting for less than 2 weeks. It can lead to cardiogenic shock and electrical instability requiring inotropic or mechanical circulatory support (144). Given the rapid progression of this potentially fatal condition, diagnostic indicators of fulminant myocarditis are of high clinical importance (145). The serum exosome levels of miR-155 and miR-320a have been suggested as noninvasive indicators of fulminant myocarditis. Notably, a multivariate logistic regression model combining the levels of miR-155 and miR-320a could accurately predict fulminant myocarditis, providing areas under the ROC curves that were significantly higher than those provided by individual miRNAs, C-reactive protein, or cardiac troponin (138). Transcriptomic analyses of circulating miRNAs revealed that miR-4763-3p was upregulated in patients with fulminant myocarditis and restored to a normal level after proper treatment. The miR-4763-3p level was negatively correlated with LVEF (R=−0.66, P=0.03) and was positively correlated with the concentration of interferon-β. Also, miR-4763-3p had an area under the ROC curve of 0.85 (95% confidence interval: 0.68 to 1.00), suggesting that miR-4763-3p may represent a highly specific diagnostic biomarker for fulminant myocarditis (139).
DCM may be the result of myocarditis or the first presentation of heart failure, and carries an increased risk of cardiac death and heart transplantation (10). Unfortunately, no established clinical markers are available to identify the risk of adverse outcomes in patients with myocarditis (131). Research has started to consider ncRNAs as a prognostic marker of myocarditis, and relevant exploration has been carried out. For example, a study by Besler et al. using EMB found that patients with inflammatory cardiomyopathy (iCMP) had increased levels of miR-155 and miR-133a compared to patients with non-inflammatory DCM. Additionally, the levels of miR-133a (R=0.73; P<0.01) and miR-155 (R=0.63; P<0.01) were correlated with inflammatory cell counts in patients with iCMP. More importantly, the Kaplan-Meier curve of adverse cardiovascular outcomes (cardiovascular death, ventricular tachycardia, and hospitalizations for heart failure) at a mean follow-up of 38 months showed that patients with low miR-133a levels had a worse cardiovascular outcome than those with high miR-133a levels, suggesting that miR-133a may be a potential prognostic biomarker for human iCMP (140). A prospective study of cardiac and inflammation-associated plasma miRNA levels in eight children with viral myocarditis showed that the circulating levels of miR-208a and miR-21 were significantly elevated in the acute phase of the disease compared to the subacute and chronic phases. Moreover, miR-208b levels during the subacute phase were correlated with systolic left ventricular function during the chronic/resolution phase, which could evidence prognostic significance for left ventricular functional recovery in patients with myocarditis (141).
Although the above findings suggest that ncRNAs may play an important role in the clinical diagnosis and prognosis of myocarditis, most studies involved limited sample sizes. Therefore, large prospective clinical trials are required to confirm the value of ncRNAs as minimally invasive biomarkers for the diagnosis and prognosis of myocarditis.
Conclusions
Although there has been great progress in research on myocarditis in recent years, great challenges remain in the diagnosis and treatment of the condition. Accumulating evidence suggests that ncRNAs play a pivotal role in myocarditis (Figure 2) and that targeting ncRNAs may represent a novel therapeutic approach for myocarditis. However, the clinical application of ncRNAs in myocarditis is hindered by an insufficient understanding of ncRNAs and the technical limitations of ncRNA oligonucleotide in targeting the design and in vivo delivery methods. The good news is that some therapeutic clinical trials of ncRNAs have begun and have achieved varying degrees of success. Improvement of oligonucleotide chemistry and delivery methods is continuously being pursued to accelerate the clinical application of ncRNAs. Notably, circulating ncRNAs can be used as diagnostic biomarkers for myocarditis due to their unique biochemical properties, overcoming the need for invasive diagnostic methods. However, larger patient cohort studies are needed to validate these findings and assess the prognostic potential of circulating ncRNAs. Also, although most studies on the role of ncRNAs in myocarditis have focused on miRNAs, lncRNAs and circRNAs may also be a treasure trove worth mining, which requires more attention in future research. With the rapid development of bio-sequencing technologies, ncRNA research is expected to provide new directions for the diagnosis and treatment of myocarditis.
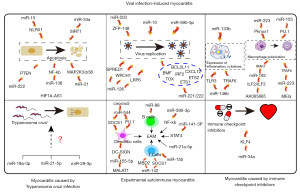
Acknowledgments
Funding: This work was supported by the National Natural Science Foundation of China (No. 82070400).
Footnote
Reporting Checklist: The authors have completed the Narrative Review reporting checklist. Available at https://atm.amegroups.com/article/view/10.21037/atm-21-6116/rc
Peer Review File: Available at https://atm.amegroups.com/article/view/10.21037/atm-21-6116/prf
Conflicts of Interest: All authors have completed the ICMJE uniform disclosure form (available at https://atm.amegroups.com/article/view/10.21037/atm-21-6116/coif). The authors have no conflicts of interest to declare.
Ethical Statement: The authors are accountable for all aspects of the work in ensuring that questions related to the accuracy or integrity of any part of the work are appropriately investigated and resolved.
Open Access Statement: This is an Open Access article distributed in accordance with the Creative Commons Attribution-NonCommercial-NoDerivs 4.0 International License (CC BY-NC-ND 4.0), which permits the non-commercial replication and distribution of the article with the strict proviso that no changes or edits are made and the original work is properly cited (including links to both the formal publication through the relevant DOI and the license). See: https://creativecommons.org/licenses/by-nc-nd/4.0/.
References
- Kindermann I, Barth C, Mahfoud F, et al. Update on myocarditis. J Am Coll Cardiol 2012;59:779-92. [Crossref] [PubMed]
- Caforio AL, Calabrese F, Angelini A, et al. A prospective study of biopsy-proven myocarditis: prognostic relevance of clinical and aetiopathogenetic features at diagnosis. Eur Heart J 2007;28:1326-33. [Crossref] [PubMed]
- Maron BJ, Towbin JA, Thiene G, et al. Contemporary definitions and classification of the cardiomyopathies: an American Heart Association Scientific Statement from the Council on Clinical Cardiology, Heart Failure and Transplantation Committee; Quality of Care and Outcomes Research and Functional Genomics and Translational Biology Interdisciplinary Working Groups; and Council on Epidemiology and Prevention. Circulation 2006;113:1807-16. [Crossref] [PubMed]
- Caforio AL, Pankuweit S, Arbustini E, et al. Current state of knowledge on aetiology, diagnosis, management, and therapy of myocarditis: a position statement of the European Society of Cardiology Working Group on Myocardial and Pericardial Diseases. Eur Heart J 2013;34:2636-48, 2648a-2648d.
- ENCODE Project Consortium. An integrated encyclopedia of DNA elements in the human genome. Nature 2012;489:57-74. [Crossref] [PubMed]
- Fang Y, Xu Y, Wang R, et al. Recent advances on the roles of LncRNAs in cardiovascular disease. J Cell Mol Med 2020;24:12246-57. [Crossref] [PubMed]
- Colpaert RMW, Calore M. Epigenetics and microRNAs in cardiovascular diseases. Genomics 2021;113:540-51. [Crossref] [PubMed]
- Tang Y, Bao J, Hu J, et al. Circular RNA in cardiovascular disease: Expression, mechanisms and clinical prospects. J Cell Mol Med 2021;25:1817-24. [Crossref] [PubMed]
- Zhang C, Xiong Y, Zeng L, et al. The Role of Non-coding RNAs in Viral Myocarditis. Front Cell Infect Microbiol 2020;10:312. [Crossref] [PubMed]
- Tschöpe C, Ammirati E, Bozkurt B, et al. Myocarditis and inflammatory cardiomyopathy: current evidence and future directions. Nat Rev Cardiol 2021;18:169-93. [Crossref] [PubMed]
- Heymans S, Eriksson U, Lehtonen J, et al. The Quest for New Approaches in Myocarditis and Inflammatory Cardiomyopathy. J Am Coll Cardiol 2016;68:2348-64. [Crossref] [PubMed]
- Błyszczuk P. Myocarditis in Humans and in Experimental Animal Models. Front Cardiovasc Med 2019;6:64. [Crossref] [PubMed]
- Rabin ER, Hassan SA, Jenson AB, et al. Coxsackie virus B3 myocarditis in mice. An electron microscopic, immunofluorescent and virus-assay study. Am J Pathol 1964;44:775-97. [PubMed]
- Grodums EI, Dempster G. Myocarditis in experimental Coxsackie B-3 infection. Can J Microbiol 1959;5:605-15. [Crossref] [PubMed]
- Fairweather D, Rose NR. Models of coxsackievirus-B3-induced myocarditis: recent advances. Drug Discovery Today: Disease Models 2004;1:381-6. [Crossref]
- Fairweather D, Rose NR. Coxsackievirus-induced myocarditis in mice: a model of autoimmune disease for studying immunotoxicity. Methods 2007;41:118-22. [Crossref] [PubMed]
- Rose NR. Viral myocarditis. Curr Opin Rheumatol 2016;28:383-9. [Crossref] [PubMed]
- Lasrado N, Gangaplara A, Massilamany C, et al. Attenuated strain of CVB3 with a mutation in the CAR-interacting region protects against both myocarditis and pancreatitis. Sci Rep 2021;11:12432. [Crossref] [PubMed]
- Kallewaard NL, Zhang L, Chen JW, et al. Tissue-specific deletion of the coxsackievirus and adenovirus receptor protects mice from virus-induced pancreatitis and myocarditis. Cell Host Microbe 2009;6:91-8. [Crossref] [PubMed]
- Pinkert S, Pryshliak M, Pappritz K, et al. Development of a new mouse model for coxsackievirus-induced myocarditis by attenuating coxsackievirus B3 virulence in the pancreas. Cardiovasc Res 2020;116:1756-66. [Crossref] [PubMed]
- Bockstahler M, Fischer A, Goetzke CC, et al. Heart-Specific Immune Responses in an Animal Model of Autoimmune-Related Myocarditis Mitigated by an Immunoproteasome Inhibitor and Genetic Ablation. Circulation 2020;141:1885-902. [Crossref] [PubMed]
- Metzger TC, Anderson MS. Myocarditis: a defect in central immune tolerance? J Clin Invest 2011;121:1251-3. [Crossref] [PubMed]
- Caforio AL, Goldman JH, Haven AJ, et al. Circulating cardiac-specific autoantibodies as markers of autoimmunity in clinical and biopsy-proven myocarditis. The Myocarditis Treatment Trial Investigators. Eur Heart J 1997;18:270-5. [Crossref] [PubMed]
- Caforio AL, Grazzini M, Mann JM, et al. Identification of alpha- and beta-cardiac myosin heavy chain isoforms as major autoantigens in dilated cardiomyopathy. Circulation 1992;85:1734-42. [Crossref] [PubMed]
- Wang ZH, Liao YH, Yuan J, et al. Continued Elevation of Plasma IL-4 and IL-17 Predicts the Progression from VMC to DCM. Dis Markers 2020;2020:9385472. [Crossref] [PubMed]
- Blyszczuk P, Müller-Edenborn B, Valenta T, et al. Transforming growth factor-β-dependent Wnt secretion controls myofibroblast formation and myocardial fibrosis progression in experimental autoimmune myocarditis. Eur Heart J 2017;38:1413-25. [PubMed]
- Yan L, Hu F, Yan X, et al. Inhibition of microRNA-155 ameliorates experimental autoimmune myocarditis by modulating Th17/Treg immune response. J Mol Med (Berl) 2016;94:1063-79. [Crossref] [PubMed]
- Neu N, Rose NR, Beisel KW, et al. Cardiac myosin induces myocarditis in genetically predisposed mice. J Immunol 1987;139:3630-6. [PubMed]
- Bustamante JM, Sanchez-Valdez F, Padilla AM, et al. A modified drug regimen clears active and dormant trypanosomes in mouse models of Chagas disease. Sci Transl Med 2020;12:eabb7656. [Crossref] [PubMed]
- Sanches TL, Cunha LD, Silva GK, et al. The use of a heterogeneously controlled mouse population reveals a significant correlation of acute phase parasitemia with mortality in Chagas disease. PLoS One 2014;9:e91640. [Crossref] [PubMed]
- Palaskas NL, Segura A, Lelenwa L, et al. Immune checkpoint inhibitor myocarditis: elucidating the spectrum of disease through endomyocardial biopsy. Eur J Heart Fail 2021;23:1725-35. [Crossref] [PubMed]
- Esposito R, Fedele T, Orefice S, et al. An Emergent Form of Cardiotoxicity: Acute Myocarditis Induced by Immune Checkpoint Inhibitors. Biomolecules 2021;11:785. [Crossref] [PubMed]
- Baik AH, Tsai KK, Oh DY, et al. Mechanisms and clinical manifestations of cardiovascular toxicities associated with immune checkpoint inhibitors. Clin Sci (Lond) 2021;135:703-24. [Crossref] [PubMed]
- Tivol EA, Borriello F, Schweitzer AN, et al. Loss of CTLA-4 leads to massive lymphoproliferation and fatal multiorgan tissue destruction, revealing a critical negative regulatory role of CTLA-4. Immunity 1995;3:541-7. [Crossref] [PubMed]
- Nishimura H, Okazaki T, Tanaka Y, et al. Autoimmune dilated cardiomyopathy in PD-1 receptor-deficient mice. Science 2001;291:319-22. [Crossref] [PubMed]
- Lucas JA, Menke J, Rabacal WA, et al. Programmed death ligand 1 regulates a critical checkpoint for autoimmune myocarditis and pneumonitis in MRL mice. J Immunol 2008;181:2513-21. [Crossref] [PubMed]
- Wang J, Okazaki IM, Yoshida T, et al. PD-1 deficiency results in the development of fatal myocarditis in MRL mice. Int Immunol 2010;22:443-52. [Crossref] [PubMed]
- Du S, Zhou L, Alexander GS, et al. PD-1 Modulates Radiation-Induced Cardiac Toxicity through Cytotoxic T Lymphocytes. J Thorac Oncol 2018;13:510-20. [Crossref] [PubMed]
- Goetzke CC, Althof N, Neumaier HL, et al. Mitigated viral myocarditis in A/J mice by the immunoproteasome inhibitor ONX 0914 depends on inhibition of systemic inflammatory responses in CoxsackievirusB3 infection. Basic Res Cardiol 2021;116:7. [Crossref] [PubMed]
- Khawaja A, Bromage DI. The innate immune response in myocarditis. Int J Biochem Cell Biol 2021;134:105973. [Crossref] [PubMed]
- Kalayinia S, Goodarzynejad H, Maleki M, et al. Next generation sequencing applications for cardiovascular disease. Ann Med 2018;50:91-109. [Crossref] [PubMed]
- Chatterjee S, Gupta SK, Bär C, et al. Noncoding RNAs: potential regulators in cardioncology. Am J Physiol Heart Circ Physiol 2019;316:H160-8. [Crossref] [PubMed]
- Lagos-Quintana M, Rauhut R, Lendeckel W, et al. Identification of novel genes coding for small expressed RNAs. Science 2001;294:853-8. [Crossref] [PubMed]
- Morris G, O'Brien D, Henshall DC. Opportunities and challenges for microRNA-targeting therapeutics for epilepsy. Trends Pharmacol Sci 2021;42:605-16. [Crossref] [PubMed]
- Ghildiyal M, Zamore PD. Small silencing RNAs: an expanding universe. Nat Rev Genet 2009;10:94-108. [Crossref] [PubMed]
- Ha M, Kim VN. Regulation of microRNA biogenesis. Nat Rev Mol Cell Biol 2014;15:509-24. [Crossref] [PubMed]
- Ryu J, Ahn Y, Kook H, et al. The roles of non-coding RNAs in vascular calcification and opportunities as therapeutic targets. Pharmacol Ther 2021;218:107675. [Crossref] [PubMed]
- Derrien T, Johnson R, Bussotti G, et al. The GENCODE v7 catalog of human long noncoding RNAs: analysis of their gene structure, evolution, and expression. Genome Res 2012;22:1775-89. [Crossref] [PubMed]
- Diederichs S. The four dimensions of non-coding RNA conservation. Trends Genet 2014;30:121-3. [Crossref] [PubMed]
- Kopp F, Mendell JT. Functional Classification and Experimental Dissection of Long Noncoding RNAs. Cell 2018;172:393-407. [Crossref] [PubMed]
- Zhang H, Liu B, Shi X, et al. Long noncoding RNAs: Potential therapeutic targets in cardiocerebrovascular diseases. Pharmacol Ther 2021;221:107744. [Crossref] [PubMed]
- Sanger HL, Klotz G, Riesner D, et al. Viroids are single-stranded covalently closed circular RNA molecules existing as highly base-paired rod-like structures. Proc Natl Acad Sci U S A 1976;73:3852-6. [Crossref] [PubMed]
- He AT, Liu J, Li F, et al. Targeting circular RNAs as a therapeutic approach: current strategies and challenges. Signal Transduct Target Ther 2021;6:185. [Crossref] [PubMed]
- Ward Z, Pearson J, Schmeier S, et al. Insights into circular RNAs: their biogenesis, detection, and emerging role in cardiovascular disease. RNA Biol 2021;18:2055-72. [Crossref] [PubMed]
- Zhou WY, Cai ZR, Liu J, et al. Circular RNA: metabolism, functions and interactions with proteins. Mol Cancer 2020;19:172. [Crossref] [PubMed]
- Lasrado N, Reddy J. An overview of the immune mechanisms of viral myocarditis. Rev Med Virol 2020;30:1-14. [Crossref] [PubMed]
- Zhao L, Fu Z. Roles of Host Immunity in Viral Myocarditis and Dilated Cardiomyopathy. J Immunol Res 2018;2018:5301548. [Crossref] [PubMed]
- Fung G, Luo H, Qiu Y, et al. Myocarditis. Circ Res 2016;118:496-514. [Crossref] [PubMed]
- Kühl U, Pauschinger M, Noutsias M, et al. High prevalence of viral genomes and multiple viral infections in the myocardium of adults with "idiopathic" left ventricular dysfunction. Circulation 2005;111:887-93. [Crossref] [PubMed]
- Lai Y, Wang M, Cheng A, et al. Regulation of Apoptosis by Enteroviruses. Front Microbiol 2020;11:1145. [Crossref] [PubMed]
- Zhu P, Chen S, Zhang W, et al. Essential Role of Non-Coding RNAs in Enterovirus Infection: From Basic Mechanisms to Clinical Prospects. Int J Mol Sci 2021;22:2904. [Crossref] [PubMed]
- Zhang X, Gao X, Hu J, et al. ADAR1p150 Forms a Complex with Dicer to Promote miRNA-222 Activity and Regulate PTEN Expression in CVB3-Induced Viral Myocarditis. Int J Mol Sci 2019;20:407. [Crossref] [PubMed]
- Mukherjee R, Vanaja KG, Boyer JA, et al. Regulation of PTEN translation by PI3K signaling maintains pathway homeostasis. Mol Cell 2021;81:708-723.e5. [Crossref] [PubMed]
- Oudit GY, Penninger JM. Cardiac regulation by phosphoinositide 3-kinases and PTEN. Cardiovasc Res 2009;82:250-60. [Crossref] [PubMed]
- Jiang D, Li M, Yu Y, et al. microRNA-34a aggravates coxsackievirus B3-induced apoptosis of cardiomyocytes through the SIRT1-p53 pathway. J Med Virol 2019;91:1643-51. [Crossref] [PubMed]
- Tong R, Jia T, Shi R, et al. Inhibition of microRNA-15 protects H9c2 cells against CVB3-induced myocardial injury by targeting NLRX1 to regulate the NLRP3 inflammasome. Cell Mol Biol Lett 2020;25:6. [Crossref] [PubMed]
- Stokman G, Kors L, Bakker PJ, et al. NLRX1 dampens oxidative stress and apoptosis in tissue injury via control of mitochondrial activity. J Exp Med 2017;214:2405-20. [Crossref] [PubMed]
- Hu B, Ding GY, Fu PY, et al. NOD-like receptor X1 functions as a tumor suppressor by inhibiting epithelial-mesenchymal transition and inducing aging in hepatocellular carcinoma cells. J Hematol Oncol 2018;11:28. [Crossref] [PubMed]
- Wang J, Wu J, Wang L, et al. The LINC00152/miR-138 Axis Facilitates Gastric Cancer Progression by Mediating SIRT2. J Oncol 2021;2021:1173869. [Crossref] [PubMed]
- Zhuo X, Bai K, Wang Y, et al. Long-chain noncoding RNA-GAS5/hsa-miR-138-5p attenuates high glucose-induced cardiomyocyte damage by targeting CYP11B2. Biosci Rep 2021;41:BSR20202232. [Crossref] [PubMed]
- Cao H, Yang B, Zhao Y, et al. The pro-apoptosis and pro-inflammation role of LncRNA HIF1A-AS1 in Coxsackievirus B3-induced myocarditis via targeting miR-138. Cardiovasc Diagn Ther 2020;10:1245-55. [Crossref] [PubMed]
- He J, Yue Y, Dong C, et al. MiR-21 confers resistance against CVB3-induced myocarditis by inhibiting PDCD4-mediated apoptosis. Clin Invest Med 2013;36:E103-11. [Crossref] [PubMed]
- He F, Xiao Z, Yao H, et al. The protective role of microRNA-21 against coxsackievirus B3 infection through targeting the MAP2K3/P38 MAPK signaling pathway. J Transl Med 2019;17:335. [Crossref] [PubMed]
- Yajima T. Viral myocarditis: potential defense mechanisms within the cardiomyocyte against virus infection. Future Microbiol 2011;6:551-66. [Crossref] [PubMed]
- Zhang Y, Sun L, Sun H, et al. Overexpression of microRNA-133b reduces myocardial injuries in children with viral myocarditis by targeting Rab27B gene. Cell Mol Biol (Noisy-le-grand) 2017;63:80-6. [Crossref] [PubMed]
- Fei Y, Chaulagain A, Wang T, et al. MiR-146a down-regulates inflammatory response by targeting TLR3 and TRAF6 in Coxsackievirus B infection. RNA 2020;26:91-100. [Crossref] [PubMed]
- Lafuse WP, Wozniak DJ, Rajaram MVS. Role of Cardiac Macrophages on Cardiac Inflammation, Fibrosis and Tissue Repair. Cells 2020;10:51. [Crossref] [PubMed]
- Zhang Y, Li X, Kong X, et al. Long non-coding RNA AK085865 ablation confers susceptibility to viral myocarditis by regulating macrophage polarization. J Cell Mol Med 2020;24:5542-54. [Crossref] [PubMed]
- Zhang Y, Li X, Wang C, et al. lncRNA AK085865 Promotes Macrophage M2 Polarization in CVB3-Induced VM by Regulating ILF2-ILF3 Complex-Mediated miRNA-192 Biogenesis. Mol Ther Nucleic Acids 2020;21:441-51. [Crossref] [PubMed]
- Mahmoud IS, Hatmal MM, Abuarqoub D, et al. 1,4-Naphthoquinone Is a Potent Inhibitor of IRAK1 Kinases and the Production of Inflammatory Cytokines in THP-1 Differentiated Macrophages. ACS Omega 2021;6:25299-310. [Crossref] [PubMed]
- Samir P, Place DE, Malireddi RKS, et al. TLR and IKK Complex-Mediated Innate Immune Signaling Inhibits Stress Granule Assembly. J Immunol 2021;207:115-24. [Crossref] [PubMed]
- Giriwono PE, Shirakawa H, Ohsaki Y, et al. Geranylgeraniol Suppresses the Expression of IRAK1 and TRAF6 to Inhibit NFκB Activation in Lipopolysaccharide-Induced Inflammatory Responses in Human Macrophage-Like Cells. Int J Mol Sci 2019;20:2320. [Crossref] [PubMed]
- Xue YL, Zhang SX, Zheng CF, et al. Long non-coding RNA MEG3 inhibits M2 macrophage polarization by activating TRAF6 via microRNA-223 down-regulation in viral myocarditis. J Cell Mol Med 2020;24:12341-54. [Crossref] [PubMed]
- Walsh MC, Lee J, Choi Y. Tumor necrosis factor receptor- associated factor 6 (TRAF6) regulation of development, function, and homeostasis of the immune system. Immunol Rev 2015;266:72-92. [Crossref] [PubMed]
- Wang J, Wu X, Jiang M, et al. Mechanism by which TRAF6 Participates in the Immune Regulation of Autoimmune Diseases and Cancer. Biomed Res Int 2020;2020:4607197. [Crossref] [PubMed]
- Gou W, Zhang Z, Yang C, et al. MiR-223/Pknox1 axis protects mice from CVB3-induced viral myocarditis by modulating macrophage polarization. Exp Cell Res 2018;366:41-8. [Crossref] [PubMed]
- Pasca S, Jurj A, Petrushev B, et al. MicroRNA-155 Implication in M1 Polarization and the Impact in Inflammatory Diseases. Front Immunol 2020;11:625. [Crossref] [PubMed]
- Corsten MF, Papageorgiou A, Verhesen W, et al. MicroRNA profiling identifies microRNA-155 as an adverse mediator of cardiac injury and dysfunction during acute viral myocarditis. Circ Res 2012;111:415-25. [Crossref] [PubMed]
- Hemida MG, Ye X, Zhang HM, et al. MicroRNA-203 enhances coxsackievirus B3 replication through targeting zinc finger protein-148. Cell Mol Life Sci 2013;70:277-91. [Crossref] [PubMed]
- Ye X, Hemida MG, Qiu Y, et al. MiR-126 promotes coxsackievirus replication by mediating cross-talk of ERK1/2 and Wnt/β-catenin signal pathways. Cell Mol Life Sci 2013;70:4631-44. [Crossref] [PubMed]
- Lorenzo C, McCormick F. SPRED proteins and their roles in signal transduction, development, and malignancy. Genes Dev 2020;34:1410-21. [Crossref] [PubMed]
- Luo H, Yanagawa B, Zhang J, et al. Coxsackievirus B3 replication is reduced by inhibition of the extracellular signal-regulated kinase (ERK) signaling pathway. J Virol 2002;76:3365-73. [Crossref] [PubMed]
- Corsten MF, Heggermont W, Papageorgiou AP, et al. The microRNA-221/-222 cluster balances the antiviral and inflammatory response in viral myocarditis. Eur Heart J 2015;36:2909-19. [Crossref] [PubMed]
- Ebihara T, Taniuchi I. Transcription Factors in the Development and Function of Group 2 Innate Lymphoid Cells. Int J Mol Sci 2019;20:1377. [Crossref] [PubMed]
- Thygesen SJ, Stacey KJ. IRF1 and IRF2 regulate the non-canonical inflammasome. EMBO Rep 2019;20:e48891. [Crossref] [PubMed]
- Zarak-Crnkovic M, Kania G, Jaźwa-Kusior A, et al. Heart non-specific effector CD4+ T cells protect from postinflammatory fibrosis and cardiac dysfunction in experimental autoimmune myocarditis. Basic Res Cardiol 2019;115:6. [Crossref] [PubMed]
- Janssens R, Struyf S, Proost P. The unique structural and functional features of CXCL12. Cell Mol Immunol 2018;15:299-311. [Crossref] [PubMed]
- Jangalwe S, Kapoor VN, Xu J, et al. Cutting Edge: Early Attrition of Memory T Cells during Inflammation and Costimulation Blockade Is Regulated Concurrently by Proapoptotic Proteins Fas and Bim. J Immunol 2019;202:647-51. [Crossref] [PubMed]
- Tong L, Lin L, Wu S, et al. MiR-10a* up-regulates coxsackievirus B3 biosynthesis by targeting the 3D-coding sequence. Nucleic Acids Res 2013;41:3760-71. [Crossref] [PubMed]
- Germano JF, Sawaged S, Saadaeijahromi H, et al. Coxsackievirus B infection induces the extracellular release of miR-590-5p, a proviral microRNA. Virology 2019;529:169-76. [Crossref] [PubMed]
- Zhao S, Yang G, Liu PN, et al. miR-590-3p Is a Novel MicroRNA in Myocarditis by Targeting Nuclear Factor Kappa-B in vivo. Cardiology 2015;132:182-8. [Crossref] [PubMed]
- Pan A, Tan Y, Wang Z, et al. STAT4 silencing underlies a novel inhibitory role of microRNA-141-3p in inflammation response of mice with experimental autoimmune myocarditis. Am J Physiol Heart Circ Physiol 2019;317:H531-40. [Crossref] [PubMed]
- Mirna M, Paar V, Topf A, et al. A new player in the game: treatment with antagomiR-21a-5p significantly attenuates histological and echocardiographic effects of experimental autoimmune myocarditis. Cardiovasc Res 2022;118:556-72. [Crossref] [PubMed]
- Sun P, Wang N, Zhao P, et al. Circulating Exosomes Control CD4+ T Cell Immunometabolic Functions via the Transfer of miR-142 as a Novel Mediator in Myocarditis. Mol Ther 2020;28:2605-20. [Crossref] [PubMed]
- Chen X, Dong S, Zhang N, et al. MicroRNA-98 plays a critical role in experimental myocarditis. Int J Cardiol 2017;229:75-81. [Crossref] [PubMed]
- Chen Q, Mang G, Wu J, et al. Circular RNA circSnx5 Controls Immunogenicity of Dendritic Cells through the miR-544/SOCS1 Axis and PU.1 Activity Regulation. Mol Ther 2020;28:2503-18. [Crossref] [PubMed]
- Wu J, Zhang H, Zheng Y, et al. The Long Noncoding RNA MALAT1 Induces Tolerogenic Dendritic Cells and Regulatory T Cells via miR155/Dendritic Cell-Specific Intercellular Adhesion Molecule-3 Grabbing Nonintegrin/IL10 Axis. Front Immunol 2018;9:1847. [Crossref] [PubMed]
- Van der Borght K, Scott CL, Martens L, et al. Myocarditis Elicits Dendritic Cell and Monocyte Infiltration in the Heart and Self-Antigen Presentation by Conventional Type 2 Dendritic Cells. Front Immunol 2018;9:2714. [Crossref] [PubMed]
- Zhou T, Chen Y, Hao L, et al. DC-SIGN and immunoregulation. Cell Mol Immunol 2006;3:279-83. [PubMed]
- Kobayashi T, Yoshimura A. Keeping DCs awake by putting SOCS1 to sleep. Trends Immunol 2005;26:177-9. [Crossref] [PubMed]
- Chopin M, Lun AT, Zhan Y, et al. Transcription Factor PU.1 Promotes Conventional Dendritic Cell Identity and Function via Induction of Transcriptional Regulator DC-SCRIPT. Immunity 2019;50:77-90.e5. [Crossref] [PubMed]
- Hua X, Song J. Immune cell diversity contributes to the pathogenesis of myocarditis. Heart Fail Rev 2019;24:1019-30. [Crossref] [PubMed]
- Paiva IA, Badolato-Corrêa J, Familiar-Macedo D, et al. Th17 Cells in Viral Infections-Friend or Foe? Cells 2021;10:1159. [Crossref] [PubMed]
- Hueber W, Sands BE, Lewitzky S, et al. Secukinumab, a human anti-IL-17A monoclonal antibody, for moderate to severe Crohn's disease: unexpected results of a randomised, double-blind placebo-controlled trial. Gut 2012;61:1693-700. [Crossref] [PubMed]
- Ibrahim AF, Weirauch U, Thomas M, et al. MicroRNA replacement therapy for miR-145 and miR-33a is efficacious in a model of colon carcinoma. Cancer Res 2011;71:5214-24. [Crossref] [PubMed]
- Ferreira LRP, Ferreira FM, Laugier L, et al. Integration of miRNA and gene expression profiles suggest a role for miRNAs in the pathobiological processes of acute Trypanosoma cruzi infection. Sci Rep 2017;7:17990. [Crossref] [PubMed]
- Navarro IC, Ferreira FM, Nakaya HI, et al. MicroRNA Transcriptome Profiling in Heart of Trypanosoma cruzi-Infected Mice: Parasitological and Cardiological Outcomes. PLoS Negl Trop Dis 2015;9:e0003828. [Crossref] [PubMed]
- Laugier L, Ferreira LRP, Ferreira FM, et al. miRNAs may play a major role in the control of gene expression in key pathobiological processes in Chagas disease cardiomyopathy. PLoS Negl Trop Dis 2020;14:e0008889. [Crossref] [PubMed]
- Nonaka CKV, Macêdo CT, Cavalcante BRR, et al. Circulating miRNAs as Potential Biomarkers Associated with Cardiac Remodeling and Fibrosis in Chagas Disease Cardiomyopathy. Int J Mol Sci 2019;20:4064. [Crossref] [PubMed]
- Patel RP, Parikh R, Gunturu KS, et al. Cardiotoxicity of Immune Checkpoint Inhibitors. Curr Oncol Rep 2021;23:79. [Crossref] [PubMed]
- Johnson DB, Balko JM, Compton ML, et al. Fulminant Myocarditis with Combination Immune Checkpoint Blockade. N Engl J Med 2016;375:1749-55. [Crossref] [PubMed]
- Xia W, Zou C, Chen H, et al. Immune checkpoint inhibitor induces cardiac injury through polarizing macrophages via modulating microRNA-34a/Kruppel-like factor 4 signaling. Cell Death Dis 2020;11:575. [Crossref] [PubMed]
- Xia W, Chen H, Chen D, et al. PD-1 inhibitor inducing exosomal miR-34a-5p expression mediates the cross talk between cardiomyocyte and macrophage in immune checkpoint inhibitor-related cardiac dysfunction. J Immunother Cancer 2020;8:e001293. [Crossref] [PubMed]
- Shek D, Read SA, Akhuba L, et al. Non-coding RNA and immune-checkpoint inhibitors: friends or foes? Immunotherapy 2020;12:513-29. [Crossref] [PubMed]
- Huang XY, Zhang PF, Wei CY, et al. Circular RNA circMET drives immunosuppression and anti-PD1 therapy resistance in hepatocellular carcinoma via the miR-30-5p/snail/DPP4 axis. Mol Cancer 2020;19:92. [Crossref] [PubMed]
- Liu C, Liu R, Wang B, et al. Blocking IL-17A enhances tumor response to anti-PD-1 immunotherapy in microsatellite stable colorectal cancer. J Immunother Cancer 2021;9:e001895. Erratum in: J Immunother Cancer 2021;9: PMID: 33462141; PMCID: PMC7813395. [Crossref] [PubMed]
- Chen DL, Sheng H, Zhang DS, et al. The circular RNA circDLG1 promotes gastric cancer progression and anti-PD-1 resistance through the regulation of CXCL12 by sponging miR-141-3p. Mol Cancer 2021;20:166. [Crossref] [PubMed]
- Kara G, Calin GA, Ozpolat B. RNAi-based therapeutics and tumor targeted delivery in cancer. Adv Drug Deliv Rev 2022;182:114113. [Crossref] [PubMed]
- Momin MY, Gaddam RR, Kravitz M, et al. The Challenges and Opportunities in the Development of MicroRNA Therapeutics: A Multidisciplinary Viewpoint. Cells 2021;10:3097. [Crossref] [PubMed]
- Kociol RD, Cooper LT, Fang JC, et al. Recognition and Initial Management of Fulminant Myocarditis: A Scientific Statement From the American Heart Association. Circulation 2020;141:e69-92. [Crossref] [PubMed]
- Piccirillo F, Watanabe M, Di Sciascio G. Diagnosis, treatment and predictors of prognosis of myocarditis. A narrative review. Cardiovasc Pathol 2021;54:107362. [Crossref] [PubMed]
- Ammirati E, Frigerio M, Adler ED, et al. Management of Acute Myocarditis and Chronic Inflammatory Cardiomyopathy: An Expert Consensus Document. Circ Heart Fail 2020;13:e007405. [Crossref] [PubMed]
- Paneni F, Volpe M. Exploring RNA biomarkers in patients with acute myocarditis. Eur Heart J 2021;42:3425-6. [Crossref] [PubMed]
- Chen ZG, Liu H, Zhang JB, et al. Upregulated microRNA-214 enhances cardiac injury by targeting ITCH during coxsackievirus infection. Mol Med Rep 2015;12:1258-64. [Crossref] [PubMed]
- Bao JL, Lin L. MiR-155 and miR-148a reduce cardiac injury by inhibiting NF-κB pathway during acute viral myocarditis. Eur Rev Med Pharmacol Sci 2014;18:2349-56. [PubMed]
- Blanco-Domínguez R, Sánchez-Díaz R, de la Fuente H, et al. A Novel Circulating MicroRNA for the Detection of Acute Myocarditis. N Engl J Med 2021;384:2014-27. [Crossref] [PubMed]
- Fan KL, Li MF, Cui F, et al. Altered exosomal miR-181d and miR-30a related to the pathogenesis of CVB3 induced myocarditis by targeting SOCS3. Eur Rev Med Pharmacol Sci 2019;23:2208-15. [PubMed]
- Zhang Y, Li X, Wang D, et al. Serum exosome microRNA panel as a noninvasive biomarker for molecular diagnosis of fulminant myocarditis. Mol Ther Methods Clin Dev 2021;20:142-51. [Crossref] [PubMed]
- Nie X, He M, Wang J, et al. Circulating miR-4763-3p Is a Novel Potential Biomarker Candidate for Human Adult Fulminant Myocarditis. Mol Ther Methods Clin Dev 2020;17:1079-87. [Crossref] [PubMed]
- Besler C, Urban D, Watzka S, et al. Endomyocardial miR-133a levels correlate with myocardial inflammation, improved left ventricular function, and clinical outcome in patients with inflammatory cardiomyopathy. Eur J Heart Fail 2016;18:1442-51. [Crossref] [PubMed]
- Goldberg L, Tirosh-Wagner T, Vardi A, et al. Circulating MicroRNAs: a Potential Biomarker for Cardiac Damage, Inflammatory Response, and Left Ventricular Function Recovery in Pediatric Viral Myocarditis. J Cardiovasc Transl Res 2018;11:319-28. [Crossref] [PubMed]
- Fernández-Ruiz I. Promising biomarker for the early, non-invasive diagnosis of myocarditis. Nat Rev Cardiol 2021;18:612. [Crossref] [PubMed]
- Eichhorn C, Bière L, Schnell F, et al. Myocarditis in Athletes Is a Challenge: Diagnosis, Risk Stratification, and Uncertainties. JACC Cardiovasc Imaging 2020;13:494-507. [Crossref] [PubMed]
- Moslehi JJ, Brinkley DM, Meijers WC. Fulminant Myocarditis: Evolving Diagnosis, Evolving Biology, Evolving Prognosis. J Am Coll Cardiol 2019;74:312-4. [Crossref] [PubMed]
- Hang W, Chen C, Seubert JM, et al. Fulminant myocarditis: a comprehensive review from etiology to treatments and outcomes. Signal Transduct Target Ther 2020;5:287. [Crossref] [PubMed]