The lncRNA TERC promotes gastric cancer cell proliferation, migration, and invasion by sponging miR-423-5p to regulate SOX12 expression
Introduction
Gastric cancer (GC) is a common type of malignancy and the 3rd leading cause of cancer-related mortality worldwide (1). The disease is associated with a significant economic burden, especially in China (2-4). In recent decades, the incidence of GC has decreased due to the development of effective screening technologies and methods for controlling Helicobacter pylori infection (5,6). Surgical resection, radiotherapy, chemotherapy, and combined therapy are currently the primary treatment options available for GC, and all have been reported to significantly improve the survival of patients with GC (7-10). However, the prognosis of patients with advanced-stage GC remains unsatisfactory, and the 5-year survival rate for patients with metastatic GC is ~30% (11). At present, the underlying molecular mechanism involved in GC development and progression remains unclear. Thus, further research to extend the current understanding of the molecular mechanisms of GC progression and identify novel therapies targeting metastasis in GC urgently needs to be conducted.
Long non-coding RNAs (lncRNAs) are non-coding RNA molecules >200 nucleotides in length that have limited protein-coding potential (12). Numerous previous studies have demonstrated that lncRNAs play regulatory roles in various biological processes, including the cell cycle, cell differentiation, apoptosis, migration, invasion, and cancer progression (13-17). There is accumulating evidence that lncRNAs may also act as competing endogenous RNAs (ceRNAs) that are able to adsorb microRNAs (miRNAs/miRs), and thus influence tumorigenesis (18,19). For example, lncRNA long intergenic non-protein coding RNA 2620 (BCRT1) was found to promote breast cancer progression by binding with miR-1303 (20). In bladder cancer, lncRNA cancer suspectibility 9 (CASC9) adsorbed miR-758-3p to induce cell proliferation and epithelial-mesenchymal transition (EMT) by regulating transforming growth factor-beta 2 (TGF-β2) expression (21). It has also been reported that the lncRNA Pvt1 oncogene (PVT1) promotes cell migration by sponging miR-30a and regulating snail family transcriptional repressor 1 expression in GC (22). Qu et al. (23) also demonstrated that lncRNA HOXA cluster antisense RNA 3 promoted GC progression by sponging miR-29a-3p, which subsequently regulated lymphotoxin β receptor expression and activated nuclear factor kappa B signaling.
We identified that the expression level of lncRNA telomerase RNA component (TERC) was consistently significantly up-regulated in GCs in the Gene Expression Omnibus (GEO) database via through bioinformatics analysis. The TERC is an important RNA component of telomerase, and the lncRNA TERC, a non-coding RNA, provides a template sequence for telomere synthesis (24). The lncRNA TERC has also been reported to alleviate the progression of osteoporosis by sponging miR-217 and upregulating RUNX family transcription factor 2 (RUNX2) expression (25). However, to the best of our knowledge, the biological functions of the lncRNA TERC in the progression of cancer, especially GC, remain largely unknown.
The current study sought to determine the expression levels of the lncRNA TERC in GC tissues and cell lines. In addition, the effects of the lncRNA TERC on GC cell proliferation, invasion, and migration were analyzed. We further predicted that the binding site between lncRNA TERC and miR-423-5p, and the binding site between miR-423-5p and sex determining region Y-box 12 (SOX12). Therefore, further mechanistic studies were performed to explore the role of the lncRNA TERC/miR-423-5p/SOX12 signaling axis in the progression of GC. Together, the findings of the present study may provide novel insights into the potential of the lncRNA TERC/miR-423-5p/SOX12 signaling axis as a treatment target for GC. We present the following article in accordance with the MDAR reporting checklist (available at https://atm.amegroups.com/article/view/10.21037/atm-22-3545/rc).
Methods
Patient samples
A total of 20 human GC and corresponding and adjacent normal tissues were obtained from patients admitted to Shaanxi Provincial People’s Hospital (Xi’an, China) between July 2021 and December 2021. The study was conducted in accordance with the Declaration of Helsinki (as revised in 2013). All participants provided written informed consent, and the study was approved by the Ethics Committee of Shaanxi Provincial People’s Hospital (No. 2021-186).
Bioinformatics analysis
Human GC gene expression data were obtained from the GEO data set, GSE63288. The data analysis was performed using the DEGseq package of R software (1.12.0; RStudio, Inc., Boston, MA, USA). Genes with a log2|fold change (FC)| >1 and a P<0.05 were considered differentially expressed genes. The binding sites between the lncRNA TERC and miR-423-5p were predicted using the starBase database (https://starbase.sysu.edu.cn/). The binding sites between miR-423-5p and SOX12 were predicted using the TargetScan 7.1 database (https://www.targetscan.org/vert_80/).
Cell lines and culture
The GES-1 human gastric mucosal epithelial cells and the NCI-N87, KATO3, Hs-746T, HGC-27, and SNU-1 human GC cell lines were obtained from Procell Life Science and Technology Co., Ltd. (Wuhan, China). The SNU-1, KATO3, and HGC-27 cell lines were cultured in Roswell Park Memorial Institute (RPMI)-1640 medium (Gibco; Thermo Fisher Scientific, Inc., Waltham, MA, USA) supplemented with 10% or 20% fetal bovine serum (FBS) (Gibco; Thermo Fisher Scientific, Inc.), and 1% penicillin-streptomycin solution. The GES-1, NCI-N87, and Hs-746T cell lines were cultured in Dulbecco’s Modified Eagle Medium (Gibco; Thermo Fisher Scientific, Inc.) supplemented with 10% FBS (Gibco; Thermo Fisher Scientific, Inc.), and 1% penicillin-streptomycin solution. All the cells were maintained at 37 ℃ in a 5% carbon dioxide humidified incubator.
Cell transfection
A total of 5×105 HGC-27 and SNU-1 cells/well were seeded into a 6-well plate overnight at 37 ℃. The cells were then cultured in serum-free RPMI-1640 medium for 2 h prior to transfection. The cells were subsequently transiently transfected with small interfering RNA. The sequences of small interfering RNA in TERC and miR-423-5p were listed in Table 1. They were transfected into cells by using Lipofectamine® 2000 reagent (Invitrogen; Thermo Fisher Scientific, Inc.) in accordance with the manufacturer’s protocol.
Table 1
Primer | Primer sequence |
---|---|
TERC | Forward: 5'-CCTGCCGCCTTCCACCGTTCATT-3' |
Reverse: 5'-GGGGACTCGCTCCGTTCCTCTTCC-3' | |
β-actin | Forward: 5'-CCCTGGAGAAGAGCTACGAG-3' |
Reverse: 5'-CGTACAGGTCTTTGCGGATG-3' | |
miR-423-5p | Loop primer: 5'-GTCGTATCCAGTGCAGGGTCCGAGGTATTCGCACTGGATACGACAAAGTCTC-3' |
Forward primer: 5'-TGCGCTGAGGGGCAGAGAGCGAG-3' | |
U6 | Forward: 5'-CGCTTCGGCAGCACATATAC-3' |
Reverse: 5'-AAATATGGAACGCTTCACGA-3' | |
si-TERC | 5'-CCTTCCACCGTTCATTCTA-3' |
si-NC | 5'-UUCUCCGAACGUGUCACGUTT-3' |
miR-423-5p mimic | 5'-UGAGGGGCAGAGAGCGAGACUUU-3' |
mimic-NC | 5'-UUCUCCGAACGUGUCACGUTT-3' |
PCR, polymerase chain reaction; TERC, telomerase RNA component; miR, microRNA; NC, negative control.
Cell Counting Kit-8 (CCK-8) assays
The proliferative ability of the HGC-27 and SNU-1 cells was measured using CCK-8 assays. Briefly, 5×103 HGC-27 and SNU-1 cells/well were seeded into a 96-well plate and incubated overnight. The cells were then transfected for a further 48 h. Following the transfection, 10 µL of CCK-8 solution was added to each well and incubated at 37 ℃ for an additional 4 h. The absorbance of each well was measured at a wavelength of 450 nm using a microplate reader (Flexstation® 3; Molecular Devices, LLC, San Jose, CA, USA). The experiments were performed in triplicate.
Cell migration, and invasion assays
The migratory and invasive abilities of the HGC-27 and SNU-1 cells were determined using Transwell plates (24-well inserts; Corning, NY, USA). Briefly, for the migration assays, 5×105 HGC-27 and SNU-1 cells/well were seeded into a 6-well plate and incubated overnight. The cells were transfected for 48 h, trypsinized and resuspended in serum-free RPMI-1640 medium at a density of 3×105 cells/mL. A volume of 200 µL of cell suspension was added to the upper chambers of the Transwell plate, while 800 µL of growth medium (RPMI-1640 medium supplemented with 10% or 20% FBS) was added to the lower chambers. Following incubation for 24 h, the migratory cells were stained with crystal violet and counted using an inverted microscope (ECLIPSE Ts2; Nikon Corporation; magnification, ×200). The invasion assays were performed as described, with a minor alteration—the upper chamber of the Transwell plate was pre-coated with 100 µL of Matrigel (1 mg/mL; Corning, Inc.).
Reverse transcription-quantitative polymerase chain reaction (RT-qPCR)
Total RNA was extracted from the GC tissues, adjacent normal tissues, HGC-27, and SNU-1 cells using TRIzol® reagent (Invitrogen; Thermo Fisher Scientific, Inc.). Total RNA was reverse transcribed into complementary DNA (cDNA) using random primers and Hiscript Reverse Transcriptase (GeneCopoeia Company, USA) for messenger RNA quantification. For miRNA quantification, the RT step was performed using an Oligo (dT) 18/miRNA loop and Hiscript Reverse Transcriptase. The primer sequences are listed in Table 1. The PCR reaction conditions were as follows: 10 minutes at 95 ℃, followed by 40 cycles of 15 seconds at 95 ℃, and 60 seconds at 60 ℃. The expression levels were quantified using the 2−ΔΔCq method (26).
Dual luciferase reporter assays
The binding relationships between the lncRNA TERC, miR-423-5p, and SOX12 were verified using dual luciferase reporter assays. Briefly, the cDNA fragments of the TERC and SOX12 containing the predicted miR-423-5p binding sites were inserted into the pYr-MirTarget luciferase reporter vector (Yingrun Biotechnologies Inc., China) to generate pYr-MirTarget-Homo SOX12-wild-type (WT) and pYr-MirTarget-Homo TERC-WT vectors, which are henceforth denoted as SOX12-WT and TERC-WT, respectively.
A mutant (MUT) site in the miR-423-5p binding site was also designed and cloned into the pYr-MirTarget luciferase reporter vector to generate pYr-MirTarget-Homo SOX12-MUT (SOX12-MUT) and pYr-MirTarget-Homo TERC-MUT (TERC-MUT) vectors. The TERC or SOX12 plasmids (WT or MUT) were co-transfected with the miR-423-5p mimic or mimic-negative control (NC) into 293T cells. Following 48 h of transfection, a dual luciferase reporter gene assay kit (Beyotime Institute of Biotechnology, Suzhou, China) was used to determine the relative luciferase activity.
RNA immunoprecipitation (RIP) assays
A RNA-binding protein immunoprecipitation kit (MilliporeSigma, Burlington, MA, USA) was used in accordance with the manufacturer’s protocol to determine the relationship between the lncRNA TERC and miR-423-5p. Anti-argonaute 2 (AGO2) (SAB4200085, MilliporeSigma) and control immunoglobulin G (IgG) (R9255, MilliporeSigma) antibodies were used to perform the RIP assays, and the expression levels of the lncRNA TERC and miR-423-5p were subsequently evaluated using qPCR.
Western blotting
The relative protein expression levels were examined using western blotting as previously described (27). Briefly, total protein was extracted from tissue samples and HGC-27 and SNU-1 cells using ristocetin-induced platelet aggregation lysis buffer (Beyotime Institute of Biotechnology) supplemented with 1% protease inhibitor cocktail (Sigma-Aldrich; Merck KGaA). Proteins were separated by sodium dodecyl-sulfate polyacrylamide gel electrophoresis and then transferred to nitrocellulose membranes (MilliporeSigma). After blocking with 5% non‐fat milk for 2 h at room temperature, the membranes were incubated with the following primary antibodies at 4 ℃ overnight: anti-N‐cadherin (cat. no. 13116, 1:1,000 dilution), anti-E‐cadherin (cat. no. 3195, 1:1,000 dilution), anti-matrix metallopeptidase 9 (MMP9; cat. no. 13667, 1:1,000 dilution), anti-proliferating cell nuclear antigen (PCNA; cat. no. 13110, 1:1,000 dilution), and anti-β-actin (cat. no. 4970, 1:1,000 dilution), which were purchased from Cell Signaling Technology, Inc. (Beverly, MA, USA). Anti-SOX12 (cat. no. 13116, 1:1,000 dilution) was purchased from Proteintech (Chicago, IL, USA). Following the primary antibody incubation, the membranes were incubated with the appropriate secondary antibodies (Cell Signaling Technology, Inc., cat. no. 7074, 1:1,000 dilution) for 2 h at room temperature. The protein bands were visualized using an enhanced chemiluminescence (ECL) kit (Pierce; Thermo Fisher Scientific, Inc.).
Statistical analysis
All the data are presented as the mean ± standard deviation. All the experiment were replicated 3 times. The statistical analysis was performed using GraphPad Prism 6.0 software (GraphPad Software, Inc., San Diego, CA, USA). The statistical differences between the groups were determined using a Student’s t-test or one-way analysis of variance followed by a Dunnett’s post-hoc test. A P value <0.05 indicated a statistically significant difference.
Results
LncRNA TERC expression levels are upregulated in GC tissues and cell lines and associated with a poor prognosis
The present study first identified differentially expressed lncRNAs in GC using the GSE63288 data set from the GEO database. Volcano plot (see Figure 1A) and heat map (see Figure 1B) showed differentially expressed lncRNAs identified from the GEO database. The identified upregulated lncRNAs that were thought to play important roles in GC progression were subsequently further analyzed. Among the lncRNAs, the expression levels of the lncRNA TERC were found to be consistently significantly upregulated in GC in the GEO database. Thus, GC and adjacent normal tissues (n=20) were collected and the expression levels of the lncRNA TERC were determined using RT-qPCR. As Figure 1C shows, lncRNA TERC expression was significantly more upregulated in GC tissues than adjacent normal tissues. Additionally, the expression levels of the lncRNA TERC in human gastric mucosal epithelial cells and 5 GC cell lines were analyzed. Compared to the GES-1 cells, the expression levels of the lncRNA TERC were significantly upregulated in the SNU-1, HGC-27, and KATO3 cells (see Figure 1D). Additionally, as Figure 1E shows, higher lncRNA TERC expression levels were found to be associated with shorter overall survival in patients with GC. Collectively, these results suggested that lncRNA TERC expression may be upregulated in GC tissues and poor survival outcomes may be associated with lncRNA TERC expression levels in GC.
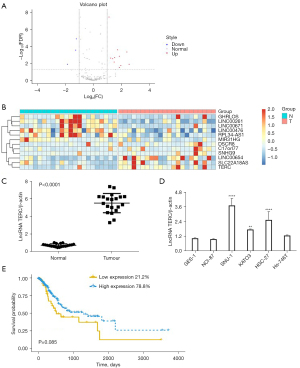
Knockdown of the lncRNA TERC inhibits GC cell proliferation, migration, and invasion
To explore the biological function of the lncRNA TERC in GC, the effects of the lncRNA TERC on the proliferation, migration, and invasion of the HGC-27 and SNU-1 cells were investigated. The si-TERC was used to knockdown the expression levels of the lncRNA TERC in the HGC-27 and SNU-1 cells, and the interference efficiency of the lncRNA TERC was detected using RT-qPCR. Compared to the si-NC group, the expression levels of the lncRNA TERC were significantly downregulated in the HGC-27 and SNU-1 cells in the si-TERC group (see Figure 2A). The lncRNA TERC knockdown also significantly reduced the proliferation of the HGC-27 and SNU-1 cells (see Figure 2B). Moreover, the migratory and invasive abilities of the HGC-27 and SNU-1 cells were significantly more reduced in the si-TERC group than the si-NC group (see Figure 2C-2F). EMT plays an important role in cancer invasiveness and metastasis (28,29). Thus, the effect of the lncRNA TERC on EMT-related markers, such as N-cadherin and E-cadherin, was also analyzed using western blotting. As Figure 3A-3C show, the expression levels of N-cadherin were significantly more downregulated in the si-TERC group, while the expression levels of E-cadherin were more upregulated compared to those in the si-NC group. Additionally, the protein expression levels of MMP9 and PCNA, which are closely associated with tumor metastasis and cell proliferation, were investigated (30,31). The results revealed that the lncRNA TERC knockdown significantly downregulated the protein expression levels of N-cadherin, MMP9 and PCNA in the HGC-27 and SNU-1 cells (see Figure 3A,3D,3E), while significantly upregulated the levels of E-cadherin (see Figure 3B). These data indicated that the lncRNA TERC may regulate the proliferation, migration, and invasion of GC cells by regulating EMT and the protein expression levels of MMP9 and PCNA.
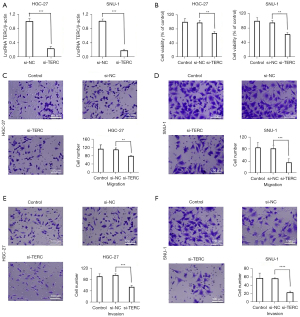
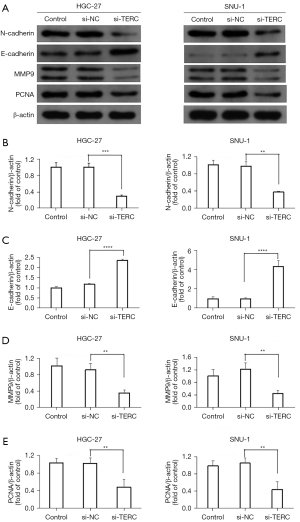
LncRNA TERC functions as a molecular sponge for miR-423-5p in GC
There is increasing evidence that the lncRNA TERC acts as a ceRNA to regulate the biological function of miRNAs (20-23). Using the starBase database (https://starbase.sysu.edu.cn/), miR-423-5p was identified as a potential target of the lncRNA TERC (see Figure 4A). To further examine the binding relationship between the lncRNA TERC and miR-423-5p, the expression levels of miR-423-5p in GC tissues and adjacent normal tissues (n=20) were analyzed using RT-qPCR. The results revealed that miR-423-5p was significantly more downregulated in the GC tissues than the normal tissues (see Figure 4B). To verify the binding relationship between the lncRNA TERC and miR-423-5p, a dual luciferase reporter assay was performed. The results showed that the overexpression of miR-423-5p significantly decreased the relative luciferase activity of the TERC-WT group, while the relative luciferase activity was unaltered in the TERC-MUT group (see Figure 4C). Moreover, the RIP analysis demonstrated that the anti-AGO2 antibody could pulldown the lncRNA TERC (see Figure 4D), and revealed the enrichment of lncRNA TERC, miR-423-5p, and SOX12 in IgG or AGO2 pulled-down RNA products in HGC-27 and SNU-1 cells (see Figure S1). The RIP analysis also revealed that the overexpression of miR-423-5p led to the substantial upregulation of lncRNA TERC expression in the RIP-AGO2 group compared to the RIP-IgG + miR-423-5p mimic or RIP-AGO2 + mimic-NC groups (see Figure 4D). Additionally, lncRNA TERC knockdown significantly upregulated the expression levels of miR-423-5p in the HGC-27 and SNU-1 cells (see Figure 4E,4F). These results suggested that miR-423-5p may be a direct target of the lncRNA TERC, and lncRNA TERC expression may be negatively associated with miR-423-5p expression.
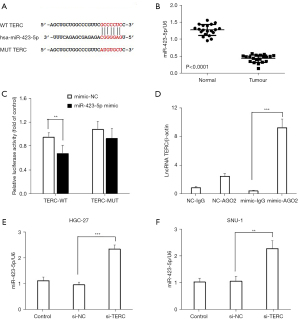
Overexpression of miR-423-5p inhibits GC cell proliferation, migration, and invasion
To further confirm the biological function of miR-423-5p in GC, the effects of miR-423-5p on the proliferation, migration, and invasion of the HGC-27 and SNU-1 cells were determined. As Figure 5A,5B show, the cell viability in miR-423-5p mimic group was significantly reduced compared to mimic-NC group. The migratory and invasive abilities of the HGC-27 and SNU-1 cells were also examined using Transwell assays. The results revealed that the migration of the HGC-27 and SNU-1 cells was significantly impaired in the miR-423-5p mimic group compared to the mimic-NC group (see Figure 5C,5D). And the invasion was also significantly impaired in the miR-423-5p mimic group compared to the mimic-NC group in the HGC-27 and SNU-1 cells (see Figure 5E,5F). These results indicated that miR-423-5p may play crucial roles in the proliferation, migration, and invasion of GC cells.
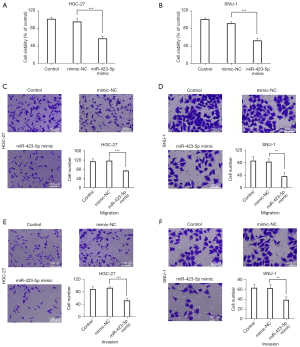
SOX12 is a downstream target of miR-423-5p and is negatively regulated by miR-423-5p in GC
Using the online software TargetScan Human 7.1, SOX12 was identified as a candidate target gene of miR-423-5p (see Figure 6A). To further validate the binding association between miR-423-5p and SOX12, the expression levels of SOX12 in GC tissues and adjacent normal tissues (n=20) were first analyzed using western blotting and RT-qPCR. As Figure 6B,6C show, SOX12 expression levels were significantly more upregulated in the GC tissues than the adjacent normal tissues. Subsequently, SOX12-WT or SOX12-MUT luciferase reporter vectors were constructed, and a dual luciferase reporter assay was performed. The results revealed that the overexpression of miR-423-5p significantly attenuated the relative luciferase activity in the SOX12-WT group, while the relative luciferase activity was unaltered in the SOX12-MUT group (see Figure 6D). Further, the effect of miR-423-5p on SOX12 expression was examined in the HGC-27 and SNU-1 cells using western blotting. The protein expression level in miR-423-5p mimic group was significantly reduced compared to mimic-NC group in the HGC-27 and SNU-1 cells (see Figure 6E,6F). These results indicated that miR-423-5p may negatively regulate SOX12 expression in GC cells.
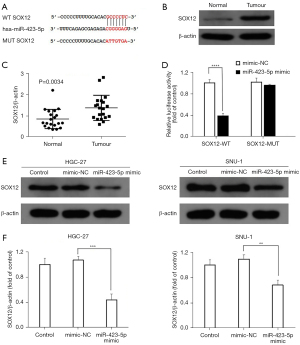
Discussion
The results of the present study revealed that lncRNA TERC expression was more upregulated in GC tissues and cell lines than adjacent normal tissues and gastric mucosal epithelial cells. High lncRNA TERC expression was also found to be associated with the poor prognosis of patients with GC. In addition, the lncRNA TERC knockdown significantly inhibited the proliferation, migration, and invasion of the HGC-27 and SNU-1 GC cell lines. The functional mechanistic studies further revealed that the lncRNA TERC regulated the expression of the SOX12 protein via miR-423-5p in GC. These data suggested that the lncRNA TERC may function as an oncogene in GC.
An increasing number of studies have suggested that lncRNAs are closely associated with the occurrence and progression of various types of cancer (32,33). For example, it was previously reported that lncRNA PVT1 was involved in the pathogenesis of human colorectal cancer (34), and lncRNA small nucleolar RNA host gene 3 (SNHG3) induced proliferation, migration, invasion and EMT in bladder cancer cells (35). Huang et al. (36) reported that lncRNA AK023391 promoted cell proliferation and invasion by targeting the phosphatidylinositol-3-kinase and protein kinase B signaling pathway in GC. Wu et al. (37) demonstrated that lncRNA SNHG11 promoted cell proliferation, migration, invasion, and EMT in GC. Consistent with these findings, the results of the present study showed that lncRNA TERC expression was more upregulated in GC tissues and the HGC-27 and SNU-1 cell lines than the adjacent normal tissues and the GES-1 gastric mucosal epithelial cells.
Upregulated lncRNA TERC expression was also found to be associated with the poor prognosis of patients with GC. Further, the results of the current study demonstrated that the knockdown of the lncRNA TERC significantly increased the proliferation, migration, and invasion of the HGC-27 and SNU-1 GC cell lines. Numerous previous studies have reported that PCNA, EMT, and MMP9 play important roles in the proliferation, migration, and invasion of cancer cells (38-43). Notably, in the present study, the results demonstrated that the lncRNA TERC knockdown significantly upregulated the expression levels of the EMT-related marker, E-cadherin, and downregulated the expression levels of PCNA, MMP9, and N-cadherin in the HGC-27 and SNU-1 GC cell lines. These results indicated that the lncRNA TERC may play a key role in GC progression.
MiRNAs are a class of non-coding RNAs, 18–25 nucleotides in length, which have been found to be involved in the regulation of tumorigenesis and cancer progression (44). It was previously reported that miR-3622a increased the proliferation and invasion of bladder cancer cells by decreasing ceramine synthase 2 expression (45). Further, miR-325-3p was discovered to promote breast cancer cell proliferation, invasion, and EMT by targeting S100 calcium binding protein A2 (46). The miR-200a/205 has been reported to be involved in the EMT process in GC cells (47). MiR-216a inhibits the metastasis of GC cells by regulating the EMT process by targeting the JAK2/STAT3 signaling pathway (48). Tang et al. (49) also reported that the overexpression of miR-423-5p significantly inhibited the proliferation, colony formation, and invasion of the ovarian cancer A2780s (also known as A2780) and A2780cp (cisplatin resistant) cell lines. The findings of the present study revealed that the expression levels of miR-423-5p were significantly more downregulated in the GC tissues than the adjacent normal tissues. The overexpression of miR-423-5p also significantly reduced the proliferation, migration, and invasion of the HGC-27 and SNU-1 GC cell lines. These data suggested that miR-423-5p may play a significant role in the regulation of GC progression.
There is increasing evidence that lncRNAs act as endogenous sponges to modulate miRNA expression and biological functions (20-23). For example, lncRNA BCRT1 promoted breast cancer progression by sponging miR-1303, thereby modulating the expression of polypyrimidine tract binding protein 3 (20). It was also reported that lncRNA CASC9 induced bladder cancer cell proliferation and EMT by sponging miR‑758‑3p, thereby upregulating TGF-β2 expression (21). Du et al. (50) found that lncRNA long intergenic non-protein coding RNA 319 acted as the sponge for miR-423-5p, which subsequently upregulated nucleus accumbens associated 1 expression and promoted the proliferation, migration, and invasion of ovarian cancer cells. Lin et al. (51) also demonstrated that lncRNA PVT1 acted as a ceRNA to sponge miR-423-5p and promoted thyroid cancer cell proliferation and invasion by upregulating p21 (RAC1) activated kinase 3 expression.
In the present study, an online prediction tool analysis identified target binding sites between the lncRNA TERC and miR-423-5p. The binding relationship between the lncRNA TERC and miR-423-5p was further determined using dual luciferase reporter and RIP assays. The results revealed that miR-423-5p significantly reduced the relative luciferase activity in the TERC-WT group, while the relative luciferase activity was unaltered in the TERC-MUT group. The results of the RIP assays also demonstrated that the overexpression of miR-423-5p significantly upregulated lncRNA TERC expression in the RIP-AGO2 group compared to the RIP-IgG + miR-423-5p mimic or RIP-AGO2 + mimic-NC groups. Additionally, the lncRNA TERC knockdown significantly upregulated miR-423-5p expression in the HGC-27 and SNU-1 cells. These results suggested that the lncRNA TERC may promote GC progression by sponging miR-423-5p.
The SOX transcription factor family comprises 20 members in vertebrates, which play an important role in cell differentiation, tumorigenesis, and embryonic development (52-54). SOX12 is a member of the SOXC family and has been reported to promote multiple malignant processes in various types of cancer (55-58). For example, it was previously demonstrated that SOX12 mediated cell proliferation and metastasis by regulating asparagine synthesis in colorectal cancer (55). Another study also found that miR-370 inhibited cell proliferation, migration, and invasion by downregulating SOX12 expression in bladder cancer (56). Ge et al. (59) reported that lncRNA long intergenic non-protein coding RNA 2908 promoted cell proliferation by regulating the miR-663a/SOX12 signaling axis in pancreatic cancer. Du et al. (60) also found that SOX12 promoted cell migration, invasion, and metastasis by upregulating MMP7 and insulin-like growth factor 1 expression in GC.
The results of the present study revealed that SOX12 was more upregulated in GC tissues than matched adjacent normal tissues. An online prediction tool analysis also identified target sites between miR-423-5p and SOX12. To further determine the binding relationship between miR-423-5p and SOX12, dual luciferase reporter assays were performed in the 293T cells. The results illustrated that the overexpression of miR-423-5p significantly attenuated the relative luciferase activity in the SOX12-WT group, while the relative luciferase activity was unaltered in the SOX12-MUT group. Further, the overexpression of miR-423-5p significantly downregulated the expression levels of SOX12 in the HGC-27 and SNU-1 cells. These data indicated that miR-423-5p may inhibit GC progression by downregulating SOX12 expression. In our next experiment, we will further confirm the associations between EMT relative proteins (such as N-cadherin, E-cadherin, MMP9, and PCNA) and miR-423-5p and SOX12, to further verify whether overexpression of miR-423-5p and knockdown of SOX12 can significantly upregulate the expression level of E-cadherin and downregulate the expression levels of PCNA, MMP9 and N-cadherin in HGC-27 and SNU-1 GC cell lines.
In conclusion, the findings of the current study indicated that lncRNA TERC expression may be significantly upregulated in GC tissues and cells and closely associated with a poor prognosis in patients with GC. The lncRNA TERC was discovered to promote the proliferation, invasion, and migration of GC cells. Further mechanistic studies revealed that the lncRNA TERC promoted cell proliferation, invasion, and migration by acting as a natural sponge of miR-335-5p and affecting SOX12 expression. Thus, these findings suggested that the lncRNA TERC may act as an oncogene in GC, and it may represent a promising prognostic biomarker and novel therapeutic target for the disease.
Acknowledgments
Funding: This work was financially supported by the Technology Talent Support Program of Shaanxi Provincial People’s Hospital (No. 2021JY-48), the Technology Talent Support Program of Shaanxi Provincial People’s Hospital (No. 2021LJ-02), the Province Key R&D Program of Shaanxi Province (No. 2021ZDLSF01-07), and Medical Research Development Fund of Beijing Kangmeng Charity Foundation (No. B21122FN).
Footnote
Reporting Checklist: The authors have completed the MDAR reporting checklist. Available at https://atm.amegroups.com/article/view/10.21037/atm-22-3545/rc
Data Sharing Statement: Available at https://atm.amegroups.com/article/view/10.21037/atm-22-3545/dss
Conflicts of Interest: All authors have completed the ICMJE uniform disclosure form (available at https://atm.amegroups.com/article/view/10.21037/atm-22-3545/coif). The authors have no conflicts of interest to declare.
Ethical Statement: The authors are accountable for all aspects of the work in ensuring that questions related to the accuracy or integrity of any part of the work are appropriately investigated and resolved. The study was conducted in accordance with the Declaration of Helsinki (as revised in 2013). All participants provided written informed consent, and the study was approved by the Ethics Committee of Shaanxi Provincial People’s Hospital (No. 2021-186).
Open Access Statement: This is an Open Access article distributed in accordance with the Creative Commons Attribution-NonCommercial-NoDerivs 4.0 International License (CC BY-NC-ND 4.0), which permits the non-commercial replication and distribution of the article with the strict proviso that no changes or edits are made and the original work is properly cited (including links to both the formal publication through the relevant DOI and the license). See: https://creativecommons.org/licenses/by-nc-nd/4.0/.
References
- Dai Q, Zhang T, Pan J, et al. LncRNA UCA1 promotes cisplatin resistance in gastric cancer via recruiting EZH2 and activating PI3K/AKT pathway. J Cancer 2020;11:3882-92. [Crossref] [PubMed]
- Hu Y, Hu D, Li W, et al. Neoadjuvant chemotherapy brings more survival benefits than postoperative chemotherapy for resectable gastric cancer: a Meta-analysis of randomized controlled trials. J BUON 2019;24:201-14. [PubMed]
- Chen W, Zheng R, Baade PD, et al. Cancer statistics in China, 2015. CA Cancer J Clin 2016;66:115-32. [Crossref] [PubMed]
- Van Cutsem E, Sagaert X, Topal B, et al. Gastric cancer. Lancet 2016;388:2654-64. [Crossref] [PubMed]
- Choi KS, Suh M. Screening for gastric cancer: the usefulness of endoscopy. Clin Endosc 2014;47:490-6. [Crossref] [PubMed]
- Hashim D, Boffetta P, La Vecchia C, et al. The global decrease in cancer mortality: trends and disparities. Ann Oncol 2016;27:926-33. [Crossref] [PubMed]
- Falzone L, Salomone S, Libra M. Evolution of Cancer Pharmacological Treatments at the Turn of the Third Millennium. Front Pharmacol 2018;9:1300. [Crossref] [PubMed]
- Leiting JL, Grotz TE. Advancements and challenges in treating advanced gastric cancer in the West. World J Gastrointest Oncol 2019;11:652-64. [Crossref] [PubMed]
- Tan Z. Recent Advances in the Surgical Treatment of Advanced Gastric Cancer: A Review. Med Sci Monit 2019;25:3537-41. [Crossref] [PubMed]
- Shinde A, Novak J, Amini A, et al. The evolving role of radiation therapy for resectable and unresectable gastric cancer. Transl Gastroenterol Hepatol 2019;4:64. [Crossref] [PubMed]
- Yamashita K, Sakuramoto S, Nemoto M, et al. Trend in gastric cancer: 35 years of surgical experience in Japan. World J Gastroenterol 2011;17:3390-7. [Crossref] [PubMed]
- Martens-Uzunova ES, Böttcher R, Croce CM, et al. Long noncoding RNA in prostate, bladder, and kidney cancer. Eur Urol 2014;65:1140-51. [Crossref] [PubMed]
- Sun M, Nie FQ, Wang ZX, et al. Involvement of lncRNA dysregulation in gastric cancer. Histol Histopathol 2016;31:33-9. [PubMed]
- Zhang Y, Su X, Kong Z, et al. An androgen reduced transcript of LncRNA GAS5 promoted prostate cancer proliferation. PLoS One 2017;12:e0182305. [Crossref] [PubMed]
- Liu HT, Fang L, Cheng YX, et al. LncRNA PVT1 regulates prostate cancer cell growth by inducing the methylation of miR-146a. Cancer Med 2016;5:3512-9. [Crossref] [PubMed]
- Zhang L, Kang W, Lu X, et al. LncRNA CASC11 promoted gastric cancer cell proliferation, migration and invasion in vitro by regulating cell cycle pathway. Cell Cycle 2018;17:1886-900. [Crossref] [PubMed]
- Zheng W, Li J, Zhou X, et al. The lncRNA XIST promotes proliferation, migration and invasion of gastric cancer cells by targeting miR-337. Arab J Gastroenterol 2020;21:199-206. [Crossref] [PubMed]
- Ding Y, Li M, Tayier T, et al. Bioinformatics analysis of lncRNA-associated ceRNA network in melanoma. J Cancer 2021;12:2921-32. [Crossref] [PubMed]
- Wang D, Chen F, Zeng T, et al. Comprehensive biological function analysis of lncRNAs in hepatocellular carcinoma. Genes Dis 2021;8:157-67. [Crossref] [PubMed]
- Liang Y, Song X, Li Y, et al. LncRNA BCRT1 promotes breast cancer progression by targeting miR-1303/PTBP3 axis. Mol Cancer 2020;19:85. [Crossref] [PubMed]
- Zhang Z, Chen F, Zhan H, et al. lncRNA CASC9 sponges miR-758-3p to promote proliferation and EMT in bladder cancer by upregulating TGF-β2. Oncol Rep 2021;45:265-77. [Crossref] [PubMed]
- Wang L, Xiao B, Yu T, et al. lncRNA PVT1 promotes the migration of gastric cancer by functioning as ceRNA of miR-30a and regulating Snail. J Cell Physiol 2021;236:536-48. [Crossref] [PubMed]
- Qu F, Zhu B, Hu YL, et al. LncRNA HOXA-AS3 promotes gastric cancer progression by regulating miR-29a-3p/LTβR and activating NF-κB signaling. Cancer Cell Int 2021;21:118. [Crossref] [PubMed]
- Gala K, Khattar E. Long non-coding RNAs at work on telomeres: Functions and implications in cancer therapy. Cancer Lett 2021;502:120-32. [Crossref] [PubMed]
- Gao GC, Yang DW, Liu W. LncRNA TERC alleviates the progression of osteoporosis by absorbing miRNA-217 to upregulate RUNX2. Eur Rev Med Pharmacol Sci 2020;24:526-34. [PubMed]
- Livak KJ, Schmittgen TD. Analysis of relative gene expression data using real-time quantitative PCR and the 2(-Delta Delta C(T)) Method. Methods 2001;25:402-8. [Crossref] [PubMed]
- Kang X, Wang H, Li Y, et al. Alantolactone induces apoptosis through ROS-mediated AKT pathway and inhibition of PINK1-mediated mitophagy in human HepG2 cells. Artif Cells Nanomed Biotechnol 2019;47:1961-70. [Crossref] [PubMed]
- Heldin CH, Vanlandewijck M, Moustakas A. Regulation of EMT by TGFβ in cancer. FEBS Lett 2012;586:1959-70. [Crossref] [PubMed]
- Vu T, Datta PK. Regulation of EMT in Colorectal Cancer: A Culprit in Metastasis. Cancers (Basel) 2017;9:171. [Crossref] [PubMed]
- Jiang Y, Zhang Y, Chu F, et al. Circ_0032821 acts as an oncogene in cell proliferation, metastasis and autophagy in human gastric cancer cells in vitro and in vivo through activating MEK1/ERK1/2 signaling pathway. Cancer Cell Int 2020;20:74. [Crossref] [PubMed]
- Chen S, Wang J. HAND2-AS1 inhibits invasion and metastasis of cervical cancer cells via microRNA-330-5p-mediated LDOC1. Cancer Cell Int 2019;19:353. [Crossref] [PubMed]
- Jiang MC, Ni JJ, Cui WY, et al. Emerging roles of lncRNA in cancer and therapeutic opportunities. Am J Cancer Res 2019;9:1354-66. [PubMed]
- Peng WX, Koirala P, Mo YY. LncRNA-mediated regulation of cell signaling in cancer. Oncogene 2017;36:5661-7. [Crossref] [PubMed]
- Wu H, Wei M, Jiang X, et al. lncRNA PVT1 Promotes Tumorigenesis of Colorectal Cancer by Stabilizing miR-16-5p and Interacting with the VEGFA/VEGFR1/AKT Axis. Mol Ther Nucleic Acids 2020;20:438-50. [Crossref] [PubMed]
- Dai G, Huang C, Yang J, et al. LncRNA SNHG3 promotes bladder cancer proliferation and metastasis through miR-515-5p/GINS2 axis. J Cell Mol Med 2020;24:9231-43. [Crossref] [PubMed]
- Huang Y, Zhang J, Hou L, et al. LncRNA AK023391 promotes tumorigenesis and invasion of gastric cancer through activation of the PI3K/Akt signaling pathway. J Exp Clin Cancer Res 2017;36:194. [Crossref] [PubMed]
- Wu Q, Ma J, Wei J, et al. lncRNA SNHG11 Promotes Gastric Cancer Progression by Activating the Wnt/β-Catenin Pathway and Oncogenic Autophagy. Mol Ther 2021;29:1258-78. [Crossref] [PubMed]
- Saitoh M. Involvement of partial EMT in cancer progression. J Biochem 2018;164:257-64. [Crossref] [PubMed]
- Duan Y, Fang Z, Shi Z, et al. Knockdown of lncRNA CCEPR suppresses colorectal cancer progression. Exp Ther Med 2019;18:3534-42. [Crossref] [PubMed]
- Wang F, Zhu W, Yang R, et al. LncRNA ZEB2-AS1 contributes to the tumorigenesis of gastric cancer via activating the Wnt/β-catenin pathway. Mol Cell Biochem 2019;456:73-83. [Crossref] [PubMed]
- Napoli S, Scuderi C, Gattuso G, et al. Functional Roles of Matrix Metalloproteinases and Their Inhibitors in Melanoma. Cells 2020;9:1151. [Crossref] [PubMed]
- Ye X, Ling B, Xu H, et al. Clinical significance of high expression of proliferating cell nuclear antigen in non-small cell lung cancer. Medicine (Baltimore) 2020;99:e19755. [Crossref] [PubMed]
- Ribatti D, Tamma R, Annese T. Epithelial-Mesenchymal Transition in Cancer: A Historical Overview. Transl Oncol 2020;13:100773. [Crossref] [PubMed]
- Lin S, Gregory RI. MicroRNA biogenesis pathways in cancer. Nat Rev Cancer 2015;15:321-33. [Crossref] [PubMed]
- Fu S, Luan T, Jiang C, et al. miR-3622a promotes proliferation and invasion of bladder cancer cells by downregulating LASS2. Gene 2019;701:23-31. [Crossref] [PubMed]
- Wang H, Hu X, Yang F, et al. miR-325-3p Promotes the Proliferation, Invasion, and EMT of Breast Cancer Cells by Directly Targeting S100A2. Oncol Res 2021;28:731-44. [Crossref] [PubMed]
- Mirzaei S, Baghaei K, Parivar K, et al. The expression level changes of microRNAs 200a/205 in the development of invasive properties in gastric cancer cells through epithelial-mesenchymal transition. Eur J Pharmacol 2019;857:172426. [Crossref] [PubMed]
- Tao Y, Yang S, Wu Y, et al. MicroRNA-216a inhibits the metastasis of gastric cancer cells by targeting JAK2/STAT3-mediated EMT process. Oncotarget 2017;8:88870-81. [Crossref] [PubMed]
- Tang X, Zeng X, Huang Y, et al. miR-423-5p serves as a diagnostic indicator and inhibits the proliferation and invasion of ovarian cancer. Exp Ther Med 2018;15:4723-30. [Crossref] [PubMed]
- Du W, Feng Z, Sun Q. LncRNA LINC00319 accelerates ovarian cancer progression through miR-423-5p/NACC1 pathway. Biochem Biophys Res Commun 2018;507:198-202. [Crossref] [PubMed]
- Lin QY, Qi QL, Hou S, et al. LncRNA PVT1 Acts as a Tumor Promoter in Thyroid Cancer and Promotes Tumor Progression by Mediating miR-423-5p-PAK3. Cancer Manag Res 2020;12:13403-13. [Crossref] [PubMed]
- Castillo SD, Sanchez-Cespedes M. The SOX family of genes in cancer development: biological relevance and opportunities for therapy. Expert Opin Ther Targets 2012;16:903-19. [Crossref] [PubMed]
- She ZY, Yang WX. SOX family transcription factors involved in diverse cellular events during development. Eur J Cell Biol 2015;94:547-63. [Crossref] [PubMed]
- Hou L, Srivastava Y, Jauch R. Molecular basis for the genome engagement by Sox proteins. Semin Cell Dev Biol 2017;63:2-12. [Crossref] [PubMed]
- Du F, Chen J, Liu H, et al. SOX12 promotes colorectal cancer cell proliferation and metastasis by regulating asparagine synthesis. Cell Death Dis 2019;10:239. [Crossref] [PubMed]
- Wang Y, Ma DL, Yu CH, et al. MicroRNA-370 suppresses SOX12 transcription and acts as a tumor suppressor in bladder cancer. Eur Rev Med Pharmacol Sci 2020;24:2303-12. [PubMed]
- Wang L, Hu F, Shen S, et al. Knockdown of SOX12 expression inhibits the proliferation and metastasis of lung cancer cells. Am J Transl Res 2017;9:4003-14. [PubMed]
- Penzo-Méndez AI. Critical roles for SoxC transcription factors in development and cancer. Int J Biochem Cell Biol 2010;42:425-8. [Crossref] [PubMed]
- Ge JN, Yan D, Ge CL, et al. LncRNA C9orf139 can regulate the growth of pancreatic cancer by mediating the miR-663a/Sox12 axis. World J Gastrointest Oncol 2020;12:1272-87. [Crossref] [PubMed]
- Du F, Feng W, Chen S, et al. Sex determining region Y-box 12 (SOX12) promotes gastric cancer metastasis by upregulating MMP7 and IGF1. Cancer Lett 2019;452:103-18. [Crossref] [PubMed]
(English Language Editor: L. Huleatt)