Adeno-associated virus vector-mediated gene therapy for the treatment of ovarian cancer: a literature review
Introduction
Despite the fact that the adeno-associated virus (AAV) was discovered and described in 1965 (1,2), the first in vivo use of the recombinant adeno-associated virus (rAAV) only occurred in the late 1980s (3). The AAV was vectorized in 1984 for gene delivery in vitro (4). In 1994, it was discovered that rAAV gene expression can be sustained in mammalian tissues , the first to demonstrate that rAAVs can transduce cells of the CNS in vivo (5), the first use of AAV vectors for the treatment of cystic fibrosis was reported in 1996 (6). In 2008 (7), AAV gene therapy was found to be effective in Leber congenital amaurosis, and in 2012 (8), the first drug for AAV gene therapy was approved by the European Medicines Agency. Luxtuna, which treats Leber amaurosis caused by RPE65 gene mutations, was the first in vivo AAV gene therapy product to receive Food and Drug Administration (FDA) approval near the end of 2017 (9). Another AAV-mediated gene therapy product to treat spinal muscular atrophy received approval in 2019 (10). Other viruses, such as lentivirus and adenovirus, can also be vectorized for gene therapy. The retroviral family, including lentiviral vectors, have been introduced into stem cells (11), including pluripotent-induced stem cells. While it has a unique effect, lentiviruses have high cytotoxicity and poor infection rates in animals. Generally, it is the first choice for in vitro cell experiments. Compared with lentivirus and adenovirus, AAV has a small size, low immunogenicity, a high degree of lowness, and high infection efficiency and tissue tropism in vivo. In compared to other viruses, AAV has the highest safety profile, can infect a variety of hosts, can infect and almost all cells in division and stationary phase, can target various tissues and organs, has a low immunogenicity, induces a relatively mild immune response in animals, and can maintain its expression for an extended period of time. In addition, compared to other viruses like lentivirus and adenovirus, it has an extremely high infection effectiveness in vivo. Of course, it has limitations of its own, such as a short carrying capacity and a 4.7 kb total genome that can be used as a vector for gene transduction with certain fixation elements. Its genome is a single-linked DNA, so when it enters the cell to be expressed, it must first be replicated into a double strand. As a result, the expression is minimal and reaches its peak expression only after infection. Adenovirus or lentivirus, which are more capable of infecting isolated cells than AAV, are typically chosen for isolated cell investigations since of their greater ability to infect isolated cells than AAV. Thus, AVV has become the first choice for animal experiments.
Gene therapy as a clinical treatment has been the subject of much research in recent years. Through the use of vectors, therapeutic genes or regulatory components can be delivered to the target cells to replace or edit genes, or delete or insert genes through DNA repair. However, gene editing may result in the incorporation of the AAV (10). While surgery and chemotherapy have improved the prognosis of patients with tumors, recent studies have shown that gene therapy may be a novel treatment option for such patients. For instance, tumor growth in hepatocytes can be inhibited by using the AAV to mediate the CRISPR/Cas9 machinery (12). Li et al. identified a new therapeutic target for the treatment of iron-regulated liver cancer metastasis by using rAAV8 (a recombinant AAV serotype 8 vector) to target mouse hepatocytes and decrease the production of SPNS2 (a protein linked to tumor metastasis) (13). For the first time, researchers led by Madisen demonstrated a link between hepatocyte replication and liver damage in adult mice given the recombinant AAV (rAAV) gene and the development of hepatocellular carcinoma (14). Pinto et al. used rAAV-mediated short hairpin (sh)RNA to target basal-like breast cancer (BLBC), and intratumoral injections slowed the tumor growth in BLBC xenograft models (15). By combining the synergistic effect of programmed death-1 (PD1)-based vaccination and soluble PD1 (sPD1)-mediated immune checkpoint blockage, a rAAV was introduced into the tumor microenvironment (TME) of mesotheliomas, providing immune protection and treatment (16).
The gynecological ovarian cancer is frequently aggressive and prone to recurrence. Currently, chemotherapy and surgery are the most common treatment modalities, however, treatment resistance presents a major obstacle and novel therapeutic approaches are urgently needed. A promising technique is gene therapy, which can modify genetic flaws and annihilate malignant cells, while boosting immunity (17). A study by Xie et al. demonstrated that delivering AAVrh.10 (a rhesus serotype 10 adeno-associated viral vector) packed with bevacizumab to a peritoneal model of ovarian cancer resulted in a 90% reduction in tumor development and an 82% reduction in angiogenesis, as well as longer survival (18). Combining current therapeutic approaches with gene editing to precisely target genes, inhibit specific oncogenes to reduce tumor cell proliferation (19,20) or examine the sensitivity of ovarian cancer cells to medications with the help of gene editing, and combine immunotherapy (21) to improve survival rates are increasingly being used in ovarian cancer. A secure and efficient delivery mechanism is necessary to guarantee the efficacy of gene therapy. Thus, AAV-mediated gene therapy may be a promising therapeutic method in other disease models or disorders. We present the following article in accordance with the Narrative Review reporting checklist (available at https://atm.amegroups.com/article/view/10.21037/atm-22-4426/rc).
Methods
We searched PubMed for original research and review articles through 2022. Research terms include various combinations of “AAV”, “AAV and cancer”, “AAV and gene therapy”, “AAV and ovarian cancer”. Table 1 describes the details.
Table 1
Items | Specification |
---|---|
Date of search | July 1, 2022 to July 10, 2022 |
Databases and other sources searched | PubMed database |
Search terms used | “AAV”, “AAV and cancer”, “AAV and gene therapy”, “AAV and ovarian cancer” |
Timeframe | 1965–2022 |
Inclusion and exclusion criteria | English clinical trials, review articles were included; case reports, Editorials |
Selection process | All authors performed the literature selection independently, and papers were gathered to be screened. According to the relevance of the content, certain references which represented the general views and novel concepts and discoveries were selected |
AAV, Adeno-associated virus.
AAV and AAV vectors
AAV
The AAV is a member of the Parvoviridae family of viruses. It is 20–26 nm in diameter, with a 4.7 kb linear single-stranded DNA genome. AAVs are bare icosahedral particles that lack an envelope (Figure 1A). There are two inverted terminal repeats (ITRs) with a combined length of 0.3 kb near the endpoints of the genome. The ITRs primarily aid in the packaging and replication of viruses. The Cap and Rep genes are encoded in the middle region of the genome. Cap expression encodes the capsid proteins that give rise to different serotypes, which may selectively target certain tissues and organs (22) (Figure 1B). More than 100 AAV variations and at least 12 natural serotypes have been identified as potential gene delivery vectors thus far, with AAV2 and AAV9 being most frequently used. The tissue affinity, infection effectiveness, and expression time of various serotypes vary. The Rep protein is involved in replication and integration of the virus. When the Rep protein is present, the viral genome is easily integrated into a specific site called the adeno-associated virus integration site 1 (AAVS1) located on human chromosome 19. The AAV cannot replicate on its own and must be accompanied by helper viruses such as adenovirus, herpes simplex virus, or bean vaccine (Figure 2). AAV vectors have been shown to induce long-term expression of transgenes in mice (23), suggesting that AAV vectors can effectively transduce to a variety of target tissues in animal models, including the liver and retina, as well as the myocardium and central nervous system. A study has demonstrated that human prostate (PC3), breast cancer (T47D), and liver cancer (Huh7) cell lines can all be transfected by AAV serotype 6 (24).
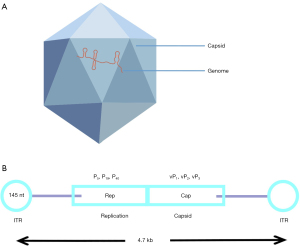
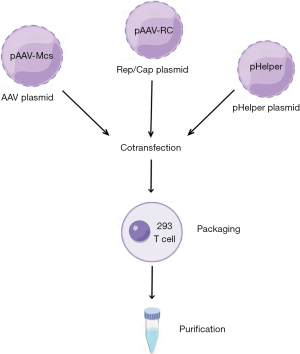
AAV vectors
A rAAV is one where the wild-type AAV genome sequence has been edited, such as by replacing the Rep and Cap sequences with the sequence to be inserted, for example, the promoter and the target gene (Figure 3). Qu et al. demonstrates that the expression of kallistatin using recombinant AAV9 inhibited the formation of lung tumors in mice (25). Alternatively, a single big target gene can be split into two or more fragments utilizing a dual vector approach, and each fragment is then packaged into a different AAV vector. Yang et al. used the AAV8-based dual vector method to reverse 10% of the hepatocyte mutations in newborn mice with Cas9-mediated metabolic liver illness (26). Yuan et al. created a rAAV9 dual-vector system to study the function of E3 ubiquitin ligase (HUWE1) in glioblastoma. When the rAAV9 dCas9-HUWE1 system was delivered to the mouse glioma xenotransplantation model in situ, tumor development was drastically diminished (27). This indicated that the system is an effective anti-glioma strategy for future research. The benefits of optimal AAV modification include increased AAV load to carry more target genes, expanded AAV capacity to transport big transgenes, and decreased immunogenicity and cytotoxicity.
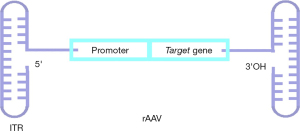
Asavarut and colleagues combined the benefits of phage and rAAV technology to create transmorphic phage/AAV (TPA) particles. These can effectively target tumor cells in vitro and in vivo to deliver immunotherapy genes, while simultaneously having no negative effects on healthy tissues (28). Kuklik et al. developed a bispecific antibody-based gene delivery method in the absence of a new tissue-specific AAV vector, suggesting that redirecting AAVs to particular target receptors are possible (29).
AAV and cancer
Gene therapy technologies
There is an extremely small possibility that AAV vectors can introduce mutations into the recipient’s genome. Chew et al. introduced AAV-CRISPR-Cas 9 in mice to establish that it elicited a host response but, in contrast to other delivery systems, it did not significantly harm cells in vivo (30). ZFN (Zinc-finger nuclease), TALEN (transcription activator-like effector nuclease), and CRISPR/Cas9 [clustered regularly interspaced short palindromic repeats (CRISPR)/CRISPR-associated (Cas) 9] technologies are the three main gene editing techniques. In recent years, CRISPR/Cas9 gene editing technology has also been used in research and clinical trials to study disorders. The CRISPR/Cas9 system developed from the long-term evolution of bacteria and archaea, and is an adaptive immune defense system. Compared to other gene editing technologies, CRISPR simplifies the process as it does not require the assembly of different modules or cloning, rather, it only requires changing the first 20 bases to target different sites. The single guide RNA (sgRNA) guides the Cas9 protein to a specific DNA site, where it recognizes the protospacer adjacent motif (PAM) sequence and cuts three to four bases upstream of it to establish a DNA double-strand break (DSB). This triggers the cell’s inherent repair mechanisms, such as non-homologous end joining (NHEJ) and homologous recombination repair (HDR), to achieve gene knockout or precise gene insertion. Considering that HDR requires a proper template, which is typically absent in naturally occurring gene changes, NHEJ is usually more effective (31). At the site of the break, NHEJ joins the two broken DNA ends together. A few base deletions or insertions may result in frameshift mutations and loss of gene function if the break is in an exon. Fu et al. demonstrated the dynamic competition between the editing outcomes following gene editing using the CRISPR-Cas9 ribonucleoprotein in combination with AAV donors (32).
AAV-mediated gene therapy
Gene editing, gene amplification, and gene suppression can all be accomplished by gene therapy, either in vivo or in vitro. Through a vector, such as a virus, in vivo gene therapy attempts to directly transfer a target gene into the patient. Traditional in vivo gene therapy employs a non-integrating vector. The transferred gene exists independently in the host cell’s nucleus and divides slowly or not at all in order to achieve the continuous expression of the relevant gene. Direct injection into the body has the advantage of ease of use. Recent advances in the study of genetic diseases and cancers have been made thanks to the discovery of AAV as a gene therapy vector, including hemophilia A (33), Duchenne muscular dystrophy (34), tumors (35), HCC (36,37), myocardial infarction (38), and so on. Drugs for AAV-mediated gene therapy, including Glybera, Luxturna, and Zolgensma, have been commercialized and utilized in patients (39). There is evidence that AAV-mediated gene editing can affect the phenotype of phenylketonuria (PKU) in congenital metabolic liver disease, and vectors have been frequently used in in vivo gene therapy (40). Merlin is a tumor suppressor protein. In xenotransplantation experiments, Yoshinaga et al. directly injected merlin into nude mice using AAV1 and noticed that the tumor disappeared within 10 weeks (41). Taniai et al. used an AAV8 vector in conjunction with small interfering (si)RNA and shRNA technology to inhibit acid ceramidase (AC) in a pancreatic cancer xenotransplantation model in mice. The AVV8 vector-mediated AC inhibition significantly slowed tumor growth and increased overall survival time (42). In another study, Liu et al. generated and isolated exosomes from AAV-packed HEK 293T cells (AAVExo). Exosomes, which can be secreted by a number of cells in both normal and abnormal conditions, are regarded as good drug delivery vehicles. Exosomes and AAV work together to improve AAV's capacity to penetrate cells, distribute AAV to target cells, and shield carriers of AAV from antibodies that can neutralize it. Compared to the standard AAV vector, AAVExo dramatically increased the efficiency of gene transfer in lung cancer-related cell lines and animal models. Moreover, it displays impressive resistance to antibody neutralization. This suggested that a new vector can be created using exosomes that include AAV to enhance the gene transfer in lung cancer cells (43). Since the presence of the blood brain barrier is a challenge in the treatment of central nervous system diseases, Chen et al. hypothesized that the AAV vector may be an excellent gene delivery mechanism for treating central nervous system diseases (44). Nawaz et al. directly infected T cells by injecting AAV carrying the chimeric antigen receptor (CAR) molecule into the abdominal cavity of mice, thereby facilitating the cytotoxic activity of CAR-T cells. The typical CAR-T cell preparation process was simplified by using the AAV-CAR virus vector, which also lowers the cost of treatment and offers a new, practical, and effective way to deliver CAR cell therapy (45).
One of the processes used in in vitro gene therapy involves isolating the patient’s own cells, transferring the therapeutic gene inside the cell, and then returning the edited cells to the patient. An integrated vector is typically used in in vitro therapy to insert the gene into the genome of the precursor cell or stem cell. This process is safe because it is primarily carried out in vitro.
The application of AAV in ovarian research and treatment
Ovarian cancer is the fifth most lethal malignancy in women. Ovarian cancer includes tumors originating from the epithelium, sex cord mesenchyme, and germ cells. Early diagnosis is challenging due to the lack of specific clinical symptoms and indications. Since the majority of diagnoses occur at the advanced stages of disease, the five-year survival rate is often poor. Available treatment options mainly include surgery, platinum-based chemotherapy, and anti-angiogenic medications. While these medicines are generally well tolerated, the majority of patients will experience some side effects during the first few months of treatment and tumors are prone to recur.
AAV vectors and other vector-mediated gene editing approaches have been used to eradicate tumors and cancer cells. Stegelmeier et al. investigated gene therapies expressing different AAV antiangiogenic agents in a mouse model of advanced ovarian epithelial cancer. All three AAV gene therapy modalities significantly prolonged the survival time, with the longest prolongation of survival being 2 months observed with AAV-bevacizumab monotherapy (46). To evaluate the expression of AAV-mediated angiogenic proteins and the survival ability of mouse models of epithelial ovarian cancer (EOC), Yu et al. injected AAV vectors expressing secretory thrombospondin-1 type I repeats (3TSR), CD47 binding peptide, or 3TSR+CD47 into the abdominal cavity of mice. The AAV10-mediated 3TSR expression vector significantly reduced the formation of primary and secondary lesions 90 days after tumor implantation in an orthotopic syngeneic mouse model of advanced EOC, and significantly increased survival (47). To detect the role of the leucine rich repeat containing 4 (LRRC4) gene in EOC metastasis, Zhao et al. used a 3D cell culture system and the orthotopic ovarian xenotransplantation model infected with AAV9 containing LRRC4 to confirm invasion and metastasis in vitro and in vivo (48). In terms of immunity, Mirandola et al. used rAAV and p38 kinase to block transduced dendritic cells and effectively produce antigen-specific effector T cells (49). To take advantage of the overexpression characteristics of epithelial cell adhesion molecule (EpCAM) in specific cancer types, some researchers have developed an anti-EpCAM antibody chimeric to an AAV2 vector and evaluated the anti-EpCAM-AAV2/shEGFR vector in mice carrying OVCAR3 (an ovarian cancer cell line). A negative control [phosphate buffered saline (PBS)], natural AAV2/ shEGFR vector (a vector for targeted silencing of EGFR genes), anti-EPCAM-AAV2/ shEGFR VR vector, and anti-EpCAM-AAV2/scEGFR vector (a control vector) were intravenously injected into mice, and tumor growth was monitored regularly. Compared with the experimental group, there was no significant difference between EpCAM-AAV2/scEGFR mice, while the group of mice injected with anti-EpCAM-AAV2/shEGFR showed a significant delay in tumor growth. Mice injected with PBS showed significant tumor-specific accumulation in EpCAM-positive mice, but no adverse accumulation in the liver (50).
Discussion
AAV vectors are currently the most effective gene delivery vector, and it is also the only delivery vector that has been approved by the FDA for introducing CRISPR gene editing technology directly into the human body. However, like other novel treatments, there are some challenges at present, such as immunogenicity and hepatotoxicity (51,52). Furthermore, its efficacy, safety, and other issues remain unclear. In addition, the production and use of viral vectors are expensive, and the cost of each treatment with approved therapies exceeds $850,000 to $1.6 million (53,54). The delivery efficiency of most AAV-mediated gene therapy is low, due to the weak tendency to tumor tissues and the prevalence of AAV neutralizing antibodies in human serum (55). With regards to the efficacy and safety, research by Ahmed et al. showed that pre-existing immunity to AAV1 in vivo does not change the anti-tumor efficacy mediated by AAV1 (56). However, some studies have suggested that AAV vectors are a cancer risk factor. For example, La Bella et al. conducted a study on 109 cases of AAV-positive HCC and showed that wild AAV infection is indirectly related to human HCC (57). A recent report on gene therapy in hemophilia A showed that the vector genome was found to be integrated in liver tissues of a dog model (58). Indeed, there has been much focus on the toxicity of carriers. Qin et al. compared the low copy number of the virus genome between tumor tissues and corresponding adjacent non-tumor tissues in a large number of cancer patients. They found that most cancer patients were AAV positive, and to some extent, this confirmed the lack of causality between AAV and cancer (including Hepatocellular carcinoma), and provided further insights into the ongoing controversy surrounding whether AAV is a cancer risk factor (59). Gene therapy is still in the exploratory stages, and it remains to be determined whether cancer therapy in mouse models will be effective in the human environment.
Ovarian cancer is a leading cause of female gynecological cancer-related deaths worldwide. Due to the lack of effective detection of early cancer, it is usually diagnosed in its advanced stages. At present, the treatment of ovarian cancer has evolved from a single method to the use of surgery, chemotherapy, targeted immunotherapy, and other methods. However, treatment is usually accompanied by some adverse events. The current status of treatment is not optimistic, and the tumor prognosis is poor and patients are likely to relapse. In ovarian cancer-related investigations, AAV’s effectiveness to build a double-stranded DNA and to translate and transcribe the foreign genome in an in vitro environment is very low since its genome is single-stranded DNA. Local injections or specific promoters can be used to express the exogenous genes, even if they infect other tissues but are not expressed in those tissues due to the presence of specific promoters, to solve the issue of expressing particular exogenous sequences in ovarian tissues without affecting other organs. Second, because of a variety of in vivo regulatory variables, the environment in organisms is complicated, and viral infection of cells results in the non-expression or low level of expression of foreign genes carried by the virus. New developments in gene editing technology, particularly when the CRISPR system successfully edited eukaryotic genomes for the first time, have sparked a worldwide obsession with the technique. The use of gene editing technologies to control specific genes through targeted knockout, insertion, and substitution as well as to eliminate the issue of viral vectors randomly integrating into chromosomes has advanced both clinical medicine and the study of life. According to our knowledge, AAV-mediated gene therapy has produced positive outcomes in a number of disease states, and some of these outcomes have made their way from the lab to clinical trials, considerably enhancing the field of translational medicine. The number of studies on AAV-mediated gene therapy for ovarian cancer has decreased recently, and there are more opportunities for ovarian cancer research, despite the fact that the disease's treatment still has a lot of unresolved issues. To provide patients with the disease more hope, people will eventually build a secure and effective gene editing system thanks to ongoing in-depth research on AAV-mediated gene therapy and ongoing AAV optimization. Thus, research into the use of AAV-mediated gene therapy in tumors is of great clinical significance for the future management of patients with ovarian cancer.
Conclusions
AAV serotypes have been widely used in gene therapy because of its affinity to different tissues, stable integration, and long-term expression. However, there are also some challenges. Detailed investigation into the role of AAVs in disease gene therapy suggests that it has great potential for future use. Herein, we reviewed the current research to provide an update on the role of AAV in tumor research, especially in ovarian cancer.
Acknowledgments
Funding: This work was supported by grants from the Health and wellness Industry Research Program Project in Gansu Province (No. GSWSKY2021-076) and the Gansu provincial natural science grant (No. 21JR1RA012).
Footnote
Reporting Checklist: The authors have completed the Narrative Review reporting checklist. Available at https://atm.amegroups.com/article/view/10.21037/atm-22-4426/rc
Conflicts of Interest: All authors have completed the ICMJE uniform disclosure form (available at https://atm.amegroups.com/article/view/10.21037/atm-22-4426/coif). The authors have no conflicts of interest to declare.
Ethical Statement: The authors are accountable for all aspects of the work in ensuring that questions related to the accuracy or integrity of any part of the work are appropriately investigated and resolved.
Open Access Statement: This is an Open Access article distributed in accordance with the Creative Commons Attribution-NonCommercial-NoDerivs 4.0 International License (CC BY-NC-ND 4.0), which permits the non-commercial replication and distribution of the article with the strict proviso that no changes or edits are made and the original work is properly cited (including links to both the formal publication through the relevant DOI and the license). See: https://creativecommons.org/licenses/by-nc-nd/4.0/.
References
- Atchison RW, Casto BC, Hammon WM. Adenovirus-associ-ated defective virus particles. Science 1965;149:754-6. [Crossref] [PubMed]
- Hoggan MD, Blacklow NR, Rowe WP. Studies of small DNA viruses found in various adenovirus preparations: physical, biological, and immunological characteristics. Proc Natl Acad Sci U S A 1966;55:1467-74. [Crossref] [PubMed]
- Samulski RJ, Chang LS, Shenk T. A recombinant plasmid from which an infectious adeno-associated virus genome can be excised in vitro and its use to study viral replication. J Virol 1987;61:3096-101. [Crossref] [PubMed]
- Hermonat PL, Muzyczka N. Use of adeno-associated virus as a mammalian DNA cloning vector: transduction of neomycin resistance into mammalian tissue culture cells. Proc Natl Acad Sci U S A 1984;81:6466-70. [Crossref] [PubMed]
- Kaplitt MG, Leone P, Samulski RJ, et al. Long-term gene expression and phenotypic correction using adeno-associated virus vectors in the mammalian brain. Nat Genet 1994;8:148-54. [Crossref] [PubMed]
- Flotte T, Carter B, Conrad C, et al. A phase I study of an adeno-associated virus-CFTR gene vector in adult CF patients with mild lung disease. Hum Gene Ther 1996;7:1145-59. [Crossref] [PubMed]
- Bainbridge JW, Smith AJ, Barker SS, et al. Effect of gene therapy on visual function in Leber's congenital amaurosis. N Engl J Med 2008;358:2231-9. [Crossref] [PubMed]
- Ylä-Herttuala S. Endgame: glybera finally recommended for approval as the first gene therapy drug in the European union. Mol Ther 2012;20:1831-2. [Crossref] [PubMed]
- Rodrigues GA, Shalaev E, Karami TK, et al. Pharmaceutical Development of AAV-Based Gene Therapy Products for the Eye. Pharm Res 2018;36:29. [Crossref] [PubMed]
- Keeler AM, Flotte TR. Recombinant Adeno-Associated Virus Gene Therapy in Light of Luxturna (and Zolgensma and Glybera): Where Are We, and How Did We Get Here? Annu Rev Virol 2019;6:601-21. [Crossref] [PubMed]
- Naldini L, Trono D, Verma IM. Lentiviral vectors, two decades later. Science 2016;353:1101-2. [Crossref] [PubMed]
- Loesch R, Caruso S, Paradis V, et al. Deleting the β-catenin degradation domain in mouse hepatocytes drives hepatocellular carcinoma or hepatoblastoma-like tumor growth. J Hepatol 2022;77:424-35. [Crossref] [PubMed]
- Li M, Tang Y, Wang D, et al. Sphingosine-1-phosphate transporter spinster homolog 2 is essential for iron-regulated metastasis of hepatocellular carcinoma. Mol Ther 2022;30:703-13. [Crossref] [PubMed]
- Madisen L, Garner AR, Shimaoka D, et al. Transgenic mice for intersectional targeting of neural sensors and effectors with high specificity and performance. Neuron 2015;85:942-58. [Crossref] [PubMed]
- Pinto C, Silva G, Ribeiro AS, et al. Evaluation of AAV-mediated delivery of shRNA to target basal-like breast cancer genetic vulnerabilities. J Biotechnol 2019;300:70-7. [Crossref] [PubMed]
- Tan Z, Chiu MS, Yan CW, et al. Eliminating mesothelioma by AAV-vectored, PD1-based vaccination in the tumor microenvironment. Mol Ther Oncolytics 2021;20:373-86. [Crossref] [PubMed]
- Shuvalov O, Petukhov A, Daks A, et al. Current genome editing tools in gene therapy: new approaches to treat cancer. Curr Gene Ther 2015;15:511-29. [Crossref] [PubMed]
- Xie Y, Hicks MJ, Kaminsky SM, et al. AAV-mediated persistent bevacizumab therapy suppresses tumor growth of ovarian cancer. Gynecol Oncol 2014;135:325-32. [Crossref] [PubMed]
- Wolf D, Fiegl H, Zeimet AG, et al. High RIG-I expression in ovarian cancer associates with an immune-escape signature and poor clinical outcome. Int J Cancer 2020;146:2007-18. [Crossref] [PubMed]
- Liu Q, Liu H, Li L, et al. ATAD2 predicts poor outcomes in patients with ovarian cancer and is a marker of proliferation. Int J Oncol 2020;56:219-31. [PubMed]
- Yahata T, Mizoguchi M, Kimura A, et al. Programmed cell death ligand 1 disruption by clustered regularly interspaced short palindromic repeats/Cas9-genome editing promotes antitumor immunity and suppresses ovarian cancer progression. Cancer Sci 2019;110:1279-92. [Crossref] [PubMed]
- Li C, Samulski RJ. Engineering adeno-associated virus vectors for gene therapy. Nat Rev Genet 2020;21:255-72. [Crossref] [PubMed]
- Zhong L, Li B, Mah CS, et al. Next generation of adeno-associated virus 2 vectors: point mutations in tyrosines lead to high-efficiency transduction at lower doses. Proc Natl Acad Sci U S A 2008;105:7827-32. [Crossref] [PubMed]
- Sayroo R, Nolasco D, Yin Z, et al. Development of novel AAV serotype 6 based vectors with selective tropism for human cancer cells. Gene Ther 2016;23:18-25. [Crossref] [PubMed]
- Qu W, Zhao J, Wu Y, et al. Recombinant Adeno-associated Virus 9-mediated Expression of Kallistatin Suppresses Lung Tumor Growth in Mice. Curr Gene Ther 2021;21:72-80. [Crossref] [PubMed]
- Yang Y, Wang L, Bell P, et al. A dual AAV system enables the Cas9-mediated correction of a metabolic liver disease in newborn mice. Nat Biotechnol 2016;34:334-8. [Crossref] [PubMed]
- Yuan Y, Wang LH, Zhao XX, et al. The E3 ubiquitin ligase HUWE1 acts through the N-Myc-DLL1-NOTCH1 signaling axis to suppress glioblastoma progression. Cancer Commun (Lond) 2022;42:868-86. [Crossref] [PubMed]
- Asavarut P, Waramit S, Suwan K, et al. Systemically targeted cancer immunotherapy and gene delivery using transmorphic particles. EMBO Mol Med 2022;14:e15418. [Crossref] [PubMed]
- Kuklik J, Michelfelder S, Schiele F, et al. Development of a Bispecific Antibody-Based Platform for Retargeting of Capsid Modified AAV Vectors. Int J Mol Sci 2021;22:8355. [Crossref] [PubMed]
- Chew WL, Tabebordbar M, Cheng JK, et al. A multifunctional AAV-CRISPR-Cas9 and its host response. Nat Methods 2016;13:868-74. [Crossref] [PubMed]
- Wang D, Zhang F, Gao G. CRISPR-Based Therapeutic Genome Editing: Strategies and In Vivo Delivery by AAV Vectors. Cell 2020;181:136-50. [Crossref] [PubMed]
- Fu YW, Dai XY, Wang WT, et al. Dynamics and competition of CRISPR-Cas9 ribonucleoproteins and AAV donor-mediated NHEJ, MMEJ and HDR editing. Nucleic Acids Res 2021;49:969-85. [Crossref] [PubMed]
- Long BR, Veron P, Kuranda K, et al. Early Phase Clinical Immunogenicity of Valoctocogene Roxaparvovec, an AAV5-Mediated Gene Therapy for Hemophilia A. Mol Ther 2021;29:597-610. [Crossref] [PubMed]
- Pickar-Oliver A, Gough V, Bohning JD, et al. Full-length dystrophin restoration via targeted exon integration by AAV-CRISPR in a humanized mouse model of Duchenne muscular dystrophy. Mol Ther 2021;29:3243-57. [Crossref] [PubMed]
- Boddu PC, Gupta AK, Kim JS, et al. Generation of scalable cancer models by combining AAV-intron-trap, CRISPR/Cas9, and inducible Cre-recombinase. Commun Biol 2021;4:1184. [Crossref] [PubMed]
- Meumann N, Schmithals C, Elenschneider L, et al. Hepatocellular Carcinoma Is a Natural Target for Adeno-Associated Virus (AAV) 2 Vectors. Cancers (Basel) 2022;14:427. [Crossref] [PubMed]
- Lv T, Xiong X, Yan W, et al. Targeting of GSDMD sensitizes HCC to anti-PD-1 by activating cGAS pathway and downregulating PD-L1 expression. J Immunother Cancer 2022;10:e004763. [Crossref] [PubMed]
- Liu S, Li K, Wagner Florencio L, et al. Gene therapy knockdown of Hippo signaling induces cardiomyocyte renewal in pigs after myocardial infarction. Sci Transl Med 2021;13:eabd6892. [Crossref] [PubMed]
- Gernoux G, Gruntman AM, Blackwood M, et al. Muscle-Directed Delivery of an AAV1 Vector Leads to Capsid-Specific T Cell Exhaustion in Nonhuman Primates and Humans. Mol Ther 2020;28:747-57. [Crossref] [PubMed]
- Villiger L, Grisch-Chan HM, Lindsay H, et al. Treatment of a metabolic liver disease by in vivo genome base editing in adult mice. Nat Med 2018;24:1519-25. [Crossref] [PubMed]
- Prabhakar S, Beauchamp RL, Cheah PS, et al. Gene replacement therapy in a schwannoma mouse model of neurofibromatosis type 2. Mol Ther Methods Clin Dev 2022;26:169-80. [Crossref] [PubMed]
- Taniai T, Shirai Y, Shimada Y, et al. Inhibition of acid ceramidase elicits mitochondrial dysfunction and oxidative stress in pancreatic cancer cells. Cancer Sci 2021;112:4570-9. [Crossref] [PubMed]
- Liu B, Li Z, Huang S, et al. AAV-Containing Exosomes as a Novel Vector for Improved Gene Delivery to Lung Cancer Cells. Front Cell Dev Biol 2021;9:707607. [Crossref] [PubMed]
- Chen W, Yao S, Wan J, et al. BBB-crossing adeno-associated virus vector: An excellent gene delivery tool for CNS disease treatment. J Control Release 2021;333:129-38. [Crossref] [PubMed]
- Nawaz W, Huang B, Xu S, et al. AAV-mediated in vivo CAR gene therapy for targeting human T-cell leukemia. Blood Cancer J 2021;11:119. [Crossref] [PubMed]
- Stegelmeier AA, Santry LA, Guilleman MM, et al. AAV-Vectored Expression of the Vascular Normalizing Agents 3TSR and Fc3TSR, and the Anti-Angiogenic Bevacizumab Extends Survival in a Murine Model of End-Stage Epithelial Ovarian Carcinoma. Biomedicines 2022;10:362. [Crossref] [PubMed]
- Yu DL, Stegelmeier AA, Chow N, et al. AAV-mediated expression of 3TSR inhibits tumor and metastatic lesion development and extends survival in a murine model of epithelial ovarian carcinoma. Cancer Gene Ther 2020;27:356-67. [Crossref] [PubMed]
- Zhao C, She X, Zhang Y, et al. LRRC4 Suppresses E-Cadherin-Dependent Collective Cell Invasion and Metastasis in Epithelial Ovarian Cancer. Front Oncol 2020;10:144. [Crossref] [PubMed]
- Mirandola L, Chiriva-Internati M, Bresalier R, et al. A novel method for efficient generation of antigen-specific effector T-cells using dendritic cells transduced with recombinant adeno-associated virus and p38 kinase blockade. J Transl Med 2019;17:424. [Crossref] [PubMed]
- Lee S, Ahn HJ. Anti-EpCAM-conjugated adeno-associated virus serotype 2 for systemic delivery of EGFR shRNA: Its retargeting and antitumor effects on OVCAR3 ovarian cancer in vivo. Acta Biomater 2019;91:258-69. [Crossref] [PubMed]
- Ronzitti G, Gross DA, Mingozzi F. Human Immune Responses to Adeno-Associated Virus (AAV) Vectors. Front Immunol 2020;11:670. [Crossref] [PubMed]
- Feldman AG, Parsons JA, Dutmer CM, et al. Subacute Liver Failure Following Gene Replacement Therapy for Spinal Muscular Atrophy Type 1. J Pediatr 2020;225:252-258.e1. [Crossref] [PubMed]
- Darrow JJ. Luxturna: FDA documents reveal the value of a costly gene therapy. Drug Discov Today 2019;24:949-54. [Crossref] [PubMed]
- Dean R, Jensen I, Cyr P, et al. An updated cost-utility model for onasemnogene abeparvovec (Zolgensma®) in spinal muscular atrophy type 1 patients and comparison with evaluation by the Institute for Clinical and Effectiveness Review (ICER). J Mark Access Health Policy 2021;9:1889841. [Crossref] [PubMed]
- Weber T. Anti-AAV Antibodies in AAV Gene Therapy: Current Challenges and Possible Solutions. Front Immunol 2021;12:658399. [Crossref] [PubMed]
- Ahmed SG, Maguire CA, Cao SA, et al. Schwannoma Gene Therapy via Adeno-Associated Viral Vector Delivery of Apoptosis-Associated Speck-like Protein Containing CARD (ASC): Preclinical Efficacy and Safety. Int J Mol Sci 2022;23:819. [Crossref] [PubMed]
- La Bella T, Imbeaud S, Peneau C, et al. Adeno-associated virus in the liver: natural history and consequences in tumour development. Gut 2020;69:737-47. [Crossref] [PubMed]
- Nguyen GN, Everett JK, Kafle S, et al. A long-term study of AAV gene therapy in dogs with hemophilia A identifies clonal expansions of transduced liver cells. Nat Biotechnol 2021;39:47-55. [Crossref] [PubMed]
- Qin W, Xu G, Tai PWL, et al. Large-scale molecular epidemiological analysis of AAV in a cancer patient population. Oncogene 2021;40:3060-71. [Crossref] [PubMed]