The pattern of brain metabolism in chronic steno-occlusive cerebral artery disease
Introduction
Dispersion of gray matter and white matter and abnormal hemodynamic changes are common in patients with chronic stenosis cerebral artery disease (1,2). Due to insufficient collateral circulation through the circle of Willis, perfusion pressure in the occluded arterial region may drop below the lower limit of automatic regulation. Diffusion increases and decreases in regional cerebral blood flow (RCBF) occur when the maximally dilated arterial bed cannot maintain RCBF at normal levels (3). It is not easy to capture these abnormal changes with conventional magnetic resonance imaging (MRI).
Magnetic resonance spectroscopy (MRS) is useful for obtaining metabolic information in either preclinical or clinical applications (4). In the MRS, metabolites determine chemical shifts [parts per million (ppm)], and each metabolite can be individually identified and quantified. The increase/decrease of several of these metabolites is indicative of a certain condition and, therefore, can be used to distinguish different pathological conditions. So far, MRS has been used in a variety of pathological conditions, such as cerebral ischemic injury, brain tumors, and multiple sclerosis. However, the study of MRS in chronic occlusive cerebrovascular disease is still limited. The purpose of this study was to use MRS to study the metabolic changes of gray matter and white matter in patients with chronic stenosis occlusive middle cerebral artery (MCA) disease. We present the following article in accordance with the MDAR reporting checklist (available at https://atm.amegroups.com/article/view/10.21037/atm-22-3993/rc).
Methods
Participants
In this study, 34 patients were collected from outpatients and inpatients in Qilu Hospital of Shandong University with unilateral chronic steno-occlusive MCA disease (22 men, 12 women; age range, 28 to 65 years; mean age, 47 years) were included. Among them, 12 patients had dizziness and mild cognitive impairment (MCI) and 16 patients had transient ischemic attacks (TIA). The clinical symptoms and history of all participants are presented in Table 1. In the patient group, the normal-appearing gray and white matter or lacunar infarcts (<3 mm in diameter) were confirmed using the axial T2-weighted fluid-attenuated inversion recovery imaging (FLAIR). To evaluate the ischemic effects on the territory of ipsilateral (affected hemisphere) MCA, only patients with unilateral Ml portion of MCA severe stenosis (stenosis >70%) or occlusion with or without ipsilateral internal carotid artery (ICA) stenosis or occlusion were enrolled in the present study. The exclusion criteria were as follows: grade 2 or 3 hypersignal in deep white matter or periventricular hypersignal on T2-weighted FLAIR, as described in previous study (5); and occlusion or stenosis of more than 30% of the MCAs in the contralateral (unaffected hemisphere). The study was conducted in accordance with the Declaration of Helsinki (as revised in 2013). The study was approved by ethics board of Qilu Hospital of Shandong University (No. 2021005) and informed consent was taken from all the patients.
Table 1
Symptoms and history | Dizziness and MCI | TIA | HBP | DM | HBP and DM | RA | SLE | No related history and symptoms |
---|---|---|---|---|---|---|---|---|
Number of patients | 12 | 16 | 14 | 8 | 3 | 3 | 2 | 6 |
Duration | >1 W | <1 W | >3 Y | >3 Y | >3 Y | <1 Y | >6 M | * |
*, N/A. MCI, mild cognitive impairment; TIA, transient ischemic attack; HBP, high blood pressure; DM, diabetes mellitus; RA, rheumatic arthritis; SLE, systemic lupus erythematosus; W, week; Y, year; M, month.
The MRI and MRS scans were performed using a 3.0T MR scanner with a quadrature birdcage head coil (GE SIGNA EXITE II3T MRI, General Electric Company, the US) and maximal gradient strength of 45 mT/m. The scanning protocol was as follows: T1-weighted transverse spin-echo sequence [repetition time (TR), 550 ms; echo time (TE), 9 ms; the number of excitations (NEX), 2]; T2-weighted transverse fast spin-echo sequence (TR, 4,600 ms; TE, 102 ms; NEX, 2); and T2-weighted FLAIR transverse fast spin-echo sequence (TR, 7,500 ms; TE, 120 ms; NEX, 2). The sections were 5 mm thick, and the matrix was 256×192. T2-weighted sagittal fast spin-echo sequence (TR, 4,600 ms; TE, 102 ms; NEX, 2; section thickness, 3 mm), axial diffusion-weighted imaging (DWI) echo-planar sequence (TR, 5,000 ms; TE, 66.5 ms; NEX, 4; section thickness, 5 mm, b=1,000 s/mm2), and 3 dimensional (3D)-time-of-flight magnetic resonance angiography (3D-TOF-MRA) (TR, 3.2 ms; TE, 22.5 ms; NEX, 1; section thickness, 1 mm). Specifically, we applied multi-voxel 1H-MRS with point-resolved spectroscopy sequence (PRESS) combined with outer volume suppression [field of vision (FOV), 240×240 mm; TR, 1,500 ms; TE, 135 ms; NEX, 1; flip angle, 90; NEX, 4; section thickness,12 mm; nominal voxel size of 10×10×12 mm; scan time, 5.6 min]. All spectra were pre-scanned using automated software on the GE SIGNA EXITE II3T MRI software 4.2, which adjusted radiofrequency (RF), transmission power, water suppression pulse power, and magnetic field uniformity.
Measurements
Two experienced neuroradiologists assessed the brain parenchymal signals on conventional MRI, DWI, and cerebral artery stenosis or occlusion on magnetic resonance angiography (MRA) or digital subtraction angiography (DSA). A consensus was reached by discussion. The volume of interest (VOI) with a voxel size of 2 cm3 was established by the PRESS volume selection gradients, which was located at the ipsilateral and contralateral corona radiata, lenticular nucleus, and thalamus. Automatic data analysis software (General Electric Medical Systems, Milwaukee, WI, USA) was used to measure the area of major peaks, including N-acetylaspartic acid (NAA), creatine (Cr), choline (Cho), lactic acid (Lac), NAA/Cr, and Cho/Cr values. To avoid confounding factors, lacunar infarcts were excluded in the region of interest (ROI). A reference database was established by collecting NAA, Cr, Cho, NAA/Cr, and Cho/Cr values of the bilateral corona radiata, lenticular nucleus, and thalamus.
Statistical analysis
Metabolite concentration of the ipsilateral and contralateral corona radiata, lenticular nucleus, and thalamus were analyzed using the software SPSS 13.0 (IBM Corp., Chicago, IL, USA). We used 2-tailed paired t-tests to compare the mean values for NAA, Cr, Cho, NAA/Cr, and Cho/Cr at the ipsilateral and contralateral corona radiata, lenticular nucleus, and thalamus. A P value <0.05 was considered statistically significant.
Results
In this study, MRA and/or DSA showed unilateral M1 portion of MCA severe stenosis (≥70% diameter reduction) in 11 patients (32.35%), and unilateral M1 portion of MCA occlusion in 25 patients (73.53%). On MRA or DSA, a few distal branches of the ipsilateral MCA were demonstrated. Conventional MRI confirmed normal brain parenchyma in 19 patients and subcortical lacunar infarcts (<3 mm in diameter) in 17 patients.
The present study showed that mean NAA values were significantly lower across all regions, but Cho and Cho/Cr were significantly higher at the contralateral corona radiata and lentiform nucleus (P<0.05). Mean Cr and NAA/Cr values were significantly lower at the ipsilateral corona radiata than the contralateral corona radiata (P<0.05), but no differences were observed at the bilateral lenticular nucleus. Mean NAA, Cr, Cho, NAA/Cr, and Cho/Cr values showed no significant differences at the ipsilateral and contralateral thalamus (Figure 1). No patient showed Lac peaks at the bilateral corona radiata, lenticular nucleus, and thalamus. All statistical results regarding these metabolites concentration are presented in Table 2 and Figures 2,3.
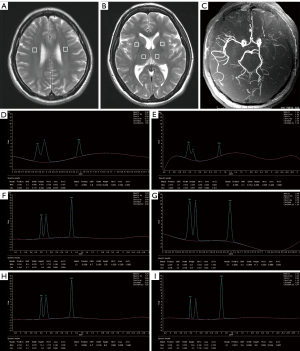
Table 2
Metabolites | Corona radiata | Lenticular nucleus | Thalamus | ||||||||
---|---|---|---|---|---|---|---|---|---|---|---|
C | I | P value | C | I | P value | C | I | P value | |||
Cho | 94,740±11,312 | 104,268±16,909 | 0.01 | 73,778±5,969 | 76,769±5,872 | 0.04 | 75,011±6,623 | 76,750±6,927 | 0.29 | ||
Cr | 84,393±10,422 | 78,541±7,910 | 0.01 | 73,611±4,567 | 72,613±4,611 | 0.37 | 71,930±4,626 | 71,163±4,510 | 0.49 | ||
NAA | 182,814±27,980 | 160,711±38,941 | 0.01 | 134,227±9,975 | 128,671±2,027 | 0.04 | 130,842±13,004 | 128,037±12,361 | 0.37 | ||
NAA/Cr | 2.35±0.44 | 1.92±0.48 | <0.01 | 1.83±0.18 | 1.78±0.20 | 0.26 | 1.83±0.20 | 1.80±0.19 | 0.67 | ||
Cho/Cr | 1.20±0.19 | 1.31±0.25 | 0.04 | 1.83±0.17 | 1.68±0.19 | 0.02 | 1.05±0.12 | 1.09±0.12 | 0.18 |
*, data are the mean ± SD. C, contralateral; I, ipsilateral; Cho, choline; NAA, N-acetyl-aspartate; Cr, creatine.
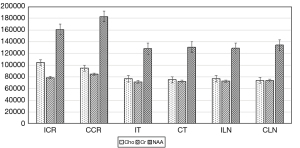
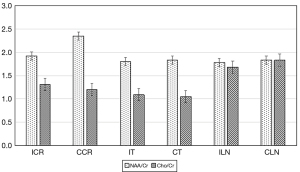
Discussion
The corona radiata is the farthest white matter perfusion area of the cortical branch of the MCA and the anterior cerebral artery (ACA), and the deep watershed of the distal parenchyma through the small artery. The central putamen and lateral segment of the globus pallidus of the lenticular nucleus and the lateral posterior thalamic nucleus of the thalamus are supplied by the central branches of the MCA (6,7). Tsuchida et al. showed that carotid artery occlusion disease leads to ischemic changes in gray matter and white matter due to hemodynamic impairment, and that the oval center may be at least as susceptible to ischemic changes due to MCA occlusion as the cortical gray matter (8). Positron emission tomography (PET) has been widely used to evaluate oxygen metabolism in brain tissue and is suitable for evaluating oxygen metabolism in gray matter (9). By contrast, MRI of white matter can be performed easily (8). Using MRI, we evaluated the corona radiata, lenticular nucleus, and thalamus of patients with unilateral chronic steno-occlusive MCA disease. In order to apply accurate spectra at the ipsilateral radiographic crown, an ROI was placed at the level of the lateral ventricle body, thus avoiding perfusion of the white matter region at the farthest end of the cortical branch of the ACA. We excluded the evaluation of metabolism at the cortical gray matter using MRS as it is thin and easily contaminated by cerebrospinal fluid (CSF).
Since the MRS parameters are affected by age, individual, magnetic field homogeneity, and temperature, we used the hemispheric ratios to determine the relationship between MRS measurements. The use of hemispherical ratios exploits the fundamental symmetry of the brain to improve sensitivity in identifying local diseases (10). Previous study has focused on metabolic diffusion, hemodynamics, and pathological changes in patients with unilateral stenosis occlusive carotid artery disease, but the hemodynamic status of the contralateral cerebral hemisphere in severe carotid artery stenosis cannot be assumed to be normal due to the possibility of interhemispheric shunt (11). To prevent the spectroscopy of ipsilateral hemisphere from being affected by perfusion through the anterior and posterior communicating artery, patients with unilateral Ml portion of MCA severe stenosis or occlusion were enrolled in the present study. Thus, hemodynamic status in the contralateral hemisphere can be assumed as normal, given the shunting of blood in the bilateral MCA territory through the anterior and posterior communicating artery is excluded.
In the present study, NAA was decreased at the ipsilateral corona radiata and lenticular nucleus (putamen), while NAA/Cr was decreased at the ipsilateral corona radiata. The level of amino acid NAA can be used as an index of neuronal viability in cerebral tissue. It can be depleted during the long duration of mitochondrial dysfunction in infarcted areas but will return to normal levels if nerve cell degeneration necrosis is prevented after timely treatment during the short duration of mitochondrial dysfunction (12,13). The reduction of NAA in ischemic stroke may be due to inhibition of NAA synthesis, accelerated catabolism of NAA, and further metabolism of NAA-related derivatives into the blood (14,15). Our findings suggested that neuronal damage (death and/or dysfunction) occurred at the ipsilateral corona radiata and lenticular nucleus (putamen). Notably, the NAA result was inconsistent with the NAA/Cr at the ipsilateral corona radiata and lenticular nucleus (putamen) in our studies, which might be caused by changes of Cr in the territory supplied by steno-occlusive MCA or caused by measurement error. This issue needs to be verified in the future.
In vivo, the Cho signal predominantly consisted of glycerophosphocholine and phosphocholine, which is a precursor of acetylcholine (16). Elevated Cho suggests increased cell membrane turnover or destruction in invasive brain tumors and demyelinating diseases (17). In a patient with acute MCA stroke, the increase in Cho signal intensity preceded the change in signal intensity shown on T2-weighted MRI. In addition, Cho/Cr values were increased in the subacute and chronic stages after stroke compared with healthy controls (18,19). Garnett et al. found that Cho/Cr was proportional to the severity of traumatic brain injury in normal-looking white matter (20). The above-mentioned studies indicated that Cho and Cho/Cr were sensitive metabolic indexes in evaluating chronic cerebral ischemia/injury. In our study, the increase of Cho and Cho/Cr indicated that nerve cell membrane damage and/or glial cells loss may occur at the ipsilateral corona radiata and lenticular nucleus (putamen) as chronic ischemia that had not yet developed into apparent morphologic changes.
The single peak at 3.0 ppm of Cr in 1H spectrum represents Cr and its phosphorylated form of phosphocreatine (PCr). They were found in high concentrations of metabolically active tissues that require sudden bursts of energy, including the brain, muscles, and heart. Polymerase chain reaction (PCR) can quickly donate its phosphate group to ADP, which can be converted into Cr to regenerate adenosine triphosphate (ATP) rapidly. Under healthy conditions, Cr is thought to vary less throughout the brain than other 1H-MRS metabolites. Therefore, it is the most common denominator for expressing the metabolite ratio of 1H (21). The results of Cr studies in brain ischemic diseases are contradictory (22). In addition, Rumpel et al. suggested that neuronal cells and glial cells loss or death after cerebral infarction would result in a decrease of Cr (23). In the present study, Cr reduced at the ipsilateral corona radiata, but there were no significant changes at the ipsilateral lenticular nucleus. Thus, our findings suggested that neuronal cells or glial cells might be lost at the ipsilateral corona radiata but not at the ipsilateral lenticular nucleus. These results, however, need to be verified in future studies with increased sample size.
As the end product of anaerobic glycolysis consumption, Lac is generally considered a marker of hypoxia and/or ischemia (24). The Lac levels elevated persistently in infarcted brain parenchyma for weeks, which is associated with inflammatory macrophages (25-27). No patient showed a Lac peak in this study.
In the present study, metabolic changes of NAA, Cr, Cho, and Lac were not found at the ipsilateral thalamus. The thalamus blood is supplied by branches of the ICA, posterior communicating artery, and the posterior cerebral artery (6,7), so there are no metabolic changes in the ipsilateral thalamus with sufficient hemodynamics in patients with unilateral chronic steno-occlusive of M1 port of MCA disease.
In conclusion, MRS may be a sensitive tool depicting metabolism changes in chronic steno-occlusive cerebral artery disease without evidence of brain abnormalities by conventional MRI. The NAA, NAA/Cr, Cho, and Cho/Cr may be used to evaluate neuronal damage, treatment strategies, and treatment effectiveness in the chronic steno-occlusive cerebral artery disease.
Acknowledgments
Funding: This work was supported by the Shandong Provincial Natural Science Foundation of China (No. ZR2015HM059).
Footnote
Reporting Checklist: The authors have completed the MDAR reporting checklist. Available at https://atm.amegroups.com/article/view/10.21037/atm-22-3993/rc
Data Sharing Statement: Available at https://atm.amegroups.com/article/view/10.21037/atm-22-3993/dss
Conflicts of Interest: All authors have completed the ICMJE uniform disclosure form (available at https://atm.amegroups.com/article/view/10.21037/atm-22-3993/coif). The authors have no conflicts of interest to declare.
Ethical Statement: The authors are accountable for all aspects of the work in ensuring that questions related to the accuracy or integrity of any part of the work are appropriately investigated and resolved. The study was conducted in accordance with the Declaration of Helsinki (as revised in 2013). The study was approved by ethics board of Qilu Hospital of Shandong University (No. 2021005) and informed consent was taken from all the patients.
Open Access Statement: This is an Open Access article distributed in accordance with the Creative Commons Attribution-NonCommercial-NoDerivs 4.0 International License (CC BY-NC-ND 4.0), which permits the non-commercial replication and distribution of the article with the strict proviso that no changes or edits are made and the original work is properly cited (including links to both the formal publication through the relevant DOI and the license). See: https://creativecommons.org/licenses/by-nc-nd/4.0/.
References
- Xie S, Hui LH, Xiao JX, et al. Detecting misery perfusion in unilateral steno-occlusive disease of the internal carotid artery or middle cerebral artery by MR imaging. AJNR Am J Neuroradiol 2011;32:1504-9. [Crossref] [PubMed]
- Meng X, Jun C, Wang Q, et al. High b-value diffusion tensor imaging of the remote white matter and white matter of obstructive unilateral cerebral arterial regions. Clin Radiol 2013;68:815-22. [Crossref] [PubMed]
- Zivadinov R, Bergsland N, Stosic M, et al. Use of perfusion- and diffusion-weighted imaging in differential diagnosis of acute and chronic ischemic stroke and multiple sclerosis. Neurol Res 2008;30:816-26. [Crossref] [PubMed]
- Oz G, Alger JR, Barker PB, et al. Clinical proton MR spectroscopy in central nervous system disorders. Radiology 2014;270:658-79. [Crossref] [PubMed]
- Jiménez-Xarrié E, Davila M, Candiota AP, et al. Brain metabolic pattern analysis using a magnetic resonance spectra classification software in experimental stroke. BMC Neurosci 2017;18:13. [Crossref] [PubMed]
- Gibo H, Carver CC, Rhoton AL Jr, et al. Microsurgical anatomy of the middle cerebral artery. J Neurosurg 1981;54:151-69. [Crossref] [PubMed]
- Hartkamp NS, Bokkers RP, van der Worp HB, et al. Distribution of cerebral blood flow in the caudate nucleus, lentiform nucleus and thalamus in patients with carotid artery stenosis. Eur Radiol 2011;21:875-81. [Crossref] [PubMed]
- Tsuchida C, Kimura H, Sadato N, et al. Evaluation of brain metabolism in steno-occlusive carotid artery disease by proton MR spectroscopy: a correlative study with oxygen metabolism by PET. J Nucl Med 2000;41:1357-62. [PubMed]
- Kobayashi M, Igarashi S, Takahashi T, et al. Optimal timing for measuring cerebral blood flow after acetazolamide administration to detect preexisting cerebral hemodynamics and metabolism in patients with bilateral major cerebral artery steno-occlusive diseases: 15O positron emission tomography studies. Am J Nucl Med Mol Imaging 2021;11:507-18. [PubMed]
- Powers WJ, Press GA, Grubb RL Jr, et al. The effect of hemodynamically significant carotid artery disease on the hemodynamic status of the cerebral circulation. Ann Intern Med 1987;106:27-34. [Crossref] [PubMed]
- Koller KJ, Zaczek R, Coyle JT. N-acetyl-aspartyl-glutamate: regional levels in rat brain and the effects of brain lesions as determined by a new HPLC method. J Neurochem 1984;43:1136-42. [Crossref] [PubMed]
- Demougeot C, Marie C, Giroud M, et al. N-acetylaspartate: a literature review of animal research on brain ischaemia. J Neurochem 2004;90:776-83. [Crossref] [PubMed]
- Chauhan A, Sharma U, Jagannathan NR, et al. Rapamycin ameliorates brain metabolites alterations after transient focal ischemia in rats. Eur J Pharmacol 2015;757:28-33. [Crossref] [PubMed]
- Harris JL, Yeh HW, Choi IY, et al. Altered neurochemical profile after traumatic brain injury: (1)H-MRS biomarkers of pathological mechanisms. J Cereb Blood Flow Metab 2012;32:2122-34. [Crossref] [PubMed]
- Berthet C, Xin L, Buscemi L, et al. Non-invasive diagnostic biomarkers for estimating the onset time of permanent cerebral ischemia. J Cereb Blood Flow Metab 2014;34:1848-55. [Crossref] [PubMed]
- Johnson B, Gay M, Zhang K, et al. The use of magnetic resonance spectroscopy in the subacute evaluation of athletes recovering from single and multiple mild traumatic brain injury. J Neurotrauma 2012;29:2297-304. [Crossref] [PubMed]
- Stovell MG, Yan JL, Sleigh A, et al. Assessing Metabolism and Injury in Acute Human Traumatic Brain Injury with Magnetic Resonance Spectroscopy: Current and Future Applications. Front Neurol 2017;8:426. [Crossref] [PubMed]
- Gideon P, Henriksen O, Sperling B, et al. Early time course of N-acetylaspartate, creatine and phosphocreatine, and compounds containing choline in the brain after acute stroke. A proton magnetic resonance spectroscopy study. Stroke 1992;23:1566-72. [Crossref] [PubMed]
- Zöllner JP, Hattingen E, Singer OC, et al. Changes of pH and energy state in subacute human ischemia assessed by multinuclear magnetic resonance spectroscopy. Stroke 2015;46:441-6. [Crossref] [PubMed]
- Garnett MR, Blamire AM, Corkill RG, et al. Early proton magnetic resonance spectroscopy in normal-appearing brain correlates with outcome in patients following traumatic brain injury. Brain 2000;123:2046-54. [Crossref] [PubMed]
- Mountford CE, Stanwell P, Lin A, et al. Neurospectroscopy: the past, present and future. Chem Rev 2010;110:3060-86. [Crossref] [PubMed]
- Panigrahy A, Borzage M, Blüml S. Basic principles and concepts underlying recent advances in magnetic resonance imaging of the developing brain. Semin Perinatol 2010;34:3-19. [Crossref] [PubMed]
- Rumpel H, Khoo JB, Chang HM, et al. Correlation of the apparent diffusion coefficient and the creatine level in early ischemic stroke: a comparison of different patterns by magnetic resonance. J Magn Reson Imaging 2001;13:335-43. [Crossref] [PubMed]
- Woo CW, Lee BS, Kim ST, et al. Correlation between lactate and neuronal cell damage in the rat brain after focal ischemia: An in vivo 1H magnetic resonance spectroscopic (1H-MRS) study. Acta Radiol 2010;51:344-50. [Crossref] [PubMed]
- Kim DH, Kim S, Jung WY, et al. The neuroprotective effects of the seeds of Cassia obtusifolia on transient cerebral global ischemia in mice. Food Chem Toxicol 2009;47:1473-9. [Crossref] [PubMed]
- Wisnowski JL, Wu TW, Reitman AJ, et al. The effects of therapeutic hypothermia on cerebral metabolism in neonates with hypoxic-ischemic encephalopathy: An in vivo 1H-MR spectroscopy study. J Cereb Blood Flow Metab 2016;36:1075-86. [Crossref] [PubMed]
- Silachev DN, Gulyaev MV, Zorova LD, et al. Magnetic resonance spectroscopy of the ischemic brain under lithium treatment. Link to mitochondrial disorders under stroke. Chem Biol Interact 2015;237:175-82. [Crossref] [PubMed]