Mechanisms of airway remodeling converge at the critical point of bronchoconstriction in asthma
Airway remodeling is a cardinal feature of asthma progression and is associated with asthma severity. However, its causes are complex, and our understanding of the mechanisms remains limited. Early identification and intervention to prevent progressive airway remodeling and decline in lung function is the ultimate goal to improve outcomes in asthma. Additionally, early identification of treatable traits with novel combined structural and functional phenotyping methods that target airway remodeling would allow a precision medicine approach.
Writing in the Annals of Translational Medicine, Huang and Qiu describe the findings of their extensive review of research advances in airway remodeling in asthma (1). The spectrum of identified mechanisms and observed associations in their review covers the major established topics of airway remodeling in asthma: airway wall thickening; epithelial cell damage; thickening of the subepithelial reticular basement membrane; hyperplasia or hypertrophy of airway smooth muscle cells; mucus gland hypertrophy; and angiogenesis. Additionally, they emphasize the effects of inflammation as a primary driver and repeated exacerbations causing pathological changes in the airway wall. It should be added that there is also evidence for mechanotransduction triggering airway remodeling (2-6).
Airway remodeling in asthma results in increased airway wall thickness, mainly studied using histology and computed tomography (CT). A significant recent finding of a CT imaging study established at the epidemiological level a predictive relationship between airway remodeling and the rate of future exacerbations and lung function decline in severe asthma (7). In another significant study, airway histology has recently established that airway remodeling occurs only in a fraction of airways and that there is considerable overlap in airway measurements between healthy controls and people with asthma (8). This critical insight into the heterogeneity of airway remodeling leads to the question if it is randomly scattered throughout the bronchial tree or regionally clustered. It should be noted that CT imaging has, in comparison to histology, the limitation that image resolution allows reliable measurements of relatively central airways with diameters ≥2 mm only. Additionally, it cannot apply the normalization by the perimeter of the basement membrane, the gold standard in histology allowing for differences in baseline smooth muscle tone and lung inflation.
Huang and Qiu review many different mechanisms of airway remodeling in asthma (1) that all appear to include the implicit assumption that they affect airways equally. That assumption suggests that remodeling should be homogeneous throughout the bronchial tree or, perhaps, have some variability due to random biological variations. However, can biological variation explain why only a fraction of airways appears to be remodeled in airway histology?
A bronchial tree is a system that consists of many airways interacting with each other. So, could a complex systems approach be relevant to our understanding of the link between the mechanisms of airway remodeling and its heterogeneity within the bronchial tree? As a thought experiment of possible behaviors and tipping points in a complex system, suppose there is a pile of sand where grains are added on top. As the height of the pile grows and its slope gets steeper, a falling grain may not only push a few other grains aside, but these grains may push others, triggering a chain reaction that leads to an avalanche going down the slope. At a critical point of stability beyond which the system has positive feedback, a single grain's impulse can trigger the emergence of an avalanche, a cluster of sliding grains, while others remain in their previous state. Such emergent phenomena with clusters of elements exhibiting different behaviors are referred to as phase separation.
During bronchoconstriction, airways in a computational model of a bronchial tree exhibit progressive homogeneous narrowing until a critical point in airway narrowing is reached. Beyond this point, a feedback loop in the airways’ behavior becomes positive amplifying minor differences (Figure 1), which is similar to an avalanche growing in size. For a simplified explanation, suppose there is a single bifurcation of airways and a tiny difference in airflow between the two branches. Less airflow in one branch results in less tidal expansion of the lung parenchyma, lowering the tethering forces at the airway wall and allowing the smooth muscle to constrict further. This positive feedback leads to a progressive narrowing and severe constriction of that airway (Figure 1). In contrast, higher airflow causing higher parenchymal expansion and tethering forces results in a relative dilation as ventilation is redistributed due to the progressively increasing constriction of the other airway.
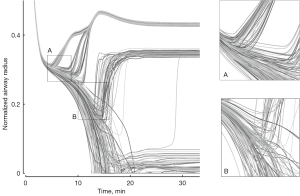
Imaging during experimentally induced bronchoconstriction mimicking an asthma attack has shown the emergence of ventilation defects (VDefs), areas of very low ventilation or gas trapping, consistent with the predictions of the computational model (10). These VDefs are caused by high resistance in small (<2 mm diameter) peripheral airways (11), and heterogeneity in airway narrowing may result in pendelluft within the bronchial tree (12).
The different mechanisms of airway remodeling contribute directly or indirectly to an increase in airway wall thickness. Additionally, the critical point of bronchoconstriction is sensitive to changes in wall thickness. Thus, the overall effect of different remodeling mechanisms is a convergence at the critical point of bronchoconstriction—the shift of the critical point due to increased airway wall thickness is conceptually independent of the dominating mechanism and the different individual contributions. Structural differences between inflammation-dependent and independent airway remodeling (13) may have a similar effect on the critical point. However, a change in total airway thickness shifts the trigger point for the emergence of VDefs and exacerbations in asthma (14).
In contrast to remodeling, airway inflammation may result in reversible increases in wall thickness so that the overall airway wall thickness has reversible and irreversible components. The difference between reversible inflammation and persistent remodeling affects the shifts in the trigger point for an exacerbation. Perhaps, this could explain why the exacerbation rate was in some longitudinal studies not associated with circulating or sputum eosinophil counts (15). Additionally, the identification of non-inflammatory asthma and COPD phenotypes using fluctuation patterns in lung function (16) suggests that variations in wall thickness, including reversible and irreversible components, affect the trigger point for asthma exacerbations (14).
The emergence of VDefs causes significant regional differences in the airways. The evidence for mechanotransduction affecting airway remodeling (3-6,17) suggests that severe bronchoconstriction within VDefs may trigger regional remodeling, and this could result in spatial correlations (18). Additionally, regional differences in airways affect the location of VDefs (19).
For future research, the question has been raised if the altered immunity in the allergic phenotype has been overemphasized at the expense of other structural abnormalities of airways (20). Asthma involves a network of disease mechanisms, including feedback loops where structural and functional changes in immune mechanisms, inflammation, remodeling, mucus in the airway lumen, and microbiota play a role. Critical points and emergent behaviors appear to be key nodes in such complex networks (21).
In summary, the review of research advances in airway remodeling in asthma is very relevant. Further investigation of airway remodeling as a component of complex airway behavior during bronchoconstriction in an airway tree is recommended. Changes in the airways of patients with asthma are not isolated relationships but part of the complex system of interconnected disease mechanisms where isolated mechanisms may not explain the system’s overall behavior and the development of structural and functional differences among airways within the bronchial tree.
Acknowledgments
Funding: None.
Footnote
Provenance and Peer Review: This article was commissioned by the editorial office, Annals of Translational Medicine. The article did not undergo external peer review.
Conflicts of Interest: The author has completed the ICMJE uniform disclosure form (available at https://atm.amegroups.com/article/view/10.21037/atm-22-5095/coif). The author has no conflicts of interest to declare.
Ethical Statement: The author is accountable for all aspects of the work in ensuring that questions related to the accuracy or integrity of any part of the work are appropriately investigated and resolved.
Open Access Statement: This is an Open Access article distributed in accordance with the Creative Commons Attribution-NonCommercial-NoDerivs 4.0 International License (CC BY-NC-ND 4.0), which permits the non-commercial replication and distribution of the article with the strict proviso that no changes or edits are made and the original work is properly cited (including links to both the formal publication through the relevant DOI and the license). See: https://creativecommons.org/licenses/by-nc-nd/4.0/.
References
- Huang Y, Qiu C. Research advances in airway remodeling in asthma: a narrative review. Ann Transl Med 2022;10:1023. [Crossref] [PubMed]
- Tschumperlin DJ, Dai G, Maly IV, et al. Mechanotransduction through growth-factor shedding into the extracellular space. Nature 2004;429:83-6. [Crossref] [PubMed]
- Grainge CL, Lau LC, Ward JA, et al. Effect of bronchoconstriction on airway remodeling in asthma. N Engl J Med 2011;364:2006-15. [Crossref] [PubMed]
- Grainge C, Park JA. Inflammatory insights into airway remodelling in asthma. Respirology 2018;23:1084-5. [Crossref] [PubMed]
- Hill MR, Philp CJ, Billington CK, et al. A theoretical model of inflammation- and mechanotransduction-driven asthmatic airway remodelling. Biomech Model Mechanobiol 2018;17:1451-70. [Crossref] [PubMed]
- O'Sullivan MJ, Phung TN, Park JA. Bronchoconstriction: a potential missing link in airway remodelling. Open Biol 2020;10:200254. [Crossref] [PubMed]
- Krings JG, Goss CW, Lew D, et al. Quantitative CT metrics are associated with longitudinal lung function decline and future asthma exacerbations: Results from SARP-3. J Allergy Clin Immunol 2021;148:752-62. [Crossref] [PubMed]
- Pascoe CD, Seow CY, Hackett TL, et al. Heterogeneity of airway wall dimensions in humans: a critical determinant of lung function in asthmatics and nonasthmatics. Am J Physiol Lung Cell Mol Physiol 2017;312:L425-31. [Crossref] [PubMed]
- Winkler T, Venegas JG. Self-organized patterns of airway narrowing. J Appl Physiol (1985) 2011;110:1482-6. [Crossref] [PubMed]
- Venegas JG, Winkler T, Musch G, et al. Self-organized patchiness in asthma as a prelude to catastrophic shifts. Nature 2005;434:777-82. [Crossref] [PubMed]
- Wongviriyawong C, Harris RS, Greenblatt E, et al. Peripheral resistance: a link between global airflow obstruction and regional ventilation distribution. J Appl Physiol (1985) 2013;114:504-14. [Crossref] [PubMed]
- Greenblatt EE, Butler JP, Venegas JG, et al. Pendelluft in the bronchial tree. J Appl Physiol (1985) 2014;117:979-88. [Crossref] [PubMed]
- Elliot JG, Noble PB, Mauad T, et al. Inflammation-dependent and independent airway remodelling in asthma. Respirology 2018;23:1138-45. [Crossref] [PubMed]
- Winkler T, Frey U. Airway remodeling: Shifting the trigger point for exacerbations in asthma. J Allergy Clin Immunol 2021;148:710-2. [Crossref] [PubMed]
- Sterk PJ, Sinha A. Emerging Complexity in the Biomarkers of Exacerbation-Prone Asthma. Am J Respir Crit Care Med 2020;202:915-7. [Crossref] [PubMed]
- Delgado-Eckert E, James A, Meier-Girard D, et al. Lung function fluctuation patterns unveil asthma and COPD phenotypes unrelated to type 2 inflammation. J Allergy Clin Immunol 2021;148:407-19. [Crossref] [PubMed]
- Tschumperlin DJ. Physical forces and airway remodeling in asthma. N Engl J Med 2011;364:2058-9. [Crossref] [PubMed]
- Pascoe CD, Green FHY, Elliot JG, et al. Airway remodelling with spatial correlations: Implications for asthma pathogenesis. Respir Physiol Neurobiol 2020;279:103469. [Crossref] [PubMed]
- Leary D, Winkler T, Braune A, et al. Effects of airway tree asymmetry on the emergence and spatial persistence of ventilation defects. J Appl Physiol (1985) 2014;117:353-62. [Crossref] [PubMed]
- Holgate ST, Wenzel S, Postma DS, et al. Asthma. Nat Rev Dis Primers 2015;1:15025. [Crossref] [PubMed]
- Winkler T. A puzzling question: how can different phenotypes possibly have indistinguishable disease symptoms? In: Sturmberg JP, editor. Embracing Complexity in Health: The Transformation of Science, Practice, and Policy. Cham: Springer International Publishing; 2019. p. 59-67.