Spermine synthase (SMS) serves as a prognostic biomarker in head and neck squamous cell carcinoma: a bioinformatics analysis
Introduction
Head and neck squamous cell carcinoma (HNSC) is the most frequent malignancy in the head and neck region, and most patients are diagnosed at advanced stages (1,2). Although HNSC management, including surgery, chemotherapy, radiotherapy, and immunotherapy, has provided some improvements, patients afflicted with HNSC have a 5-year survival rate of only 50% (3). In the past decade, large proportions of abnormal gene expression have been identified to exhibit suppress or promote role in HNSC by regulating cancer cell proliferation, migration and immune response, and so on. Meanwhile, molecular genetic landscape of HNSC provided new insights of therapeutic intervention. However, current tumor biomarkers have limitation in their sensitivity, specificity and the effectivity of targeted therapy maybe because of complicated signal network in HNSC (4,5). Therefore, exploring novel and effective biomarkers for HNSC is worthy of continuous efforts.
The spermine synthase (SMS) gene, also known as MRSR, SPMSY, SRS, and SpS, is a member of the spermidine/spermin synthase family and is located on chromosomes 1, 5, 6, and X. Current evidence indicates that the mutational activation of this gene is associated with X-linked Snyder-Robinson mental retardation syndrome (6,7). Recently, studies have shown the SMS is involved in tumorigenesis in different tumors including colorectal cancer, breast cancer and so on (8-10). Nevertheless, little is known about the potential role of SMS in the development and progression of HNSC.
In this study, we analyzed the SMS expression pattern and its relationship to the prognosis of patients with HNSC using datasets obtained from The Cancer Genome Atlas (TCGA), which is the world’s largest cancer database. At present, immunotherapy and targeted therapy are used in HNSC treatment (11,12). We investigated the relationship between SMS and tumor-infiltrating immune cells in HNSC using the Tumor Immunity Estimation Resource 2.0 (TIMER 2.0). Furthermore, in recent decades, non-coding RNAs (ncRNAs), including micro RNAs (miRNAs) and long noncoding RNAs (lncRNAs), have been recognized as key molecular regulators in cancer development. Thus, we explored the potential ceRNA networks in HNSC through a series of systematical analyses, including co-expression, miRNA-binding prediction, and survival analyses. Our findings provide new insights into the pathogenesis of HNSC and suggest novel targets for clinical treatment. We present the following article in accordance with the REMARK reporting checklist (available at https://atm.amegroups.com/article/view/10.21037/atm-22-5014/rc).
Methods
Date resources
The RNA-sequence data (Fragments Per Kilobase per Millio, FPKM) for 33 types of human cancer were obtained from TCGA database. RNA-seq data and miRNA-seq data from 504 HNSC tissues and 44 normal tissues, and corresponding clinical information were obtained from the TCGA through using the UCSC XENA tool (13). The level 3 HTSeq-FPKM data were normalized into log2 for the following analysis. The study was conducted in accordance with the Declaration of Helsinki (as revised in 2013).
Survival analysis and SMS gene expression
Gene Expression Profiling Interactive Analysis (GEPIA) is a web server used to analyze the RNA sequencing data of tumors and normal samples from TCGA and Genotype Tissue Expression data (14). In this study, we used GEPIA 2 web server to explore the prognostic value of SMS expression levels by generating the survival curves, including overall survival (OS) and disease-free survival (DFS), between the different SMS expression levels in HNSC patients. The parameters were set as follows: group cutoff = median and hazards ratio (HR) and 95% confidence interval (axis units) = months.
Kaplan-Meier Plotter
The Kaplan-Meier Plotter (15) was used to analyze the correlation between the messenger RNA (mRNA) expression of SMS and the OS of patients with HNSC. We performed a pan-cancer analysis by selecting the “Pan-cancer RNA-seq” module. The patients (n=499) were divided into two groups: high and low expression groups. The result was assessed by Kaplan-Meier OS charts and expressed as risk ratios (RRs), 95% confidence intervals (CIs), and a log-rank P value.
Analysis of SMS’s biological functions by Gene Set Enrichment Analyses (GSEA)
GSEA (16) were employed to identify the underlying biological functions and pathways of high and low SMS expression levels. The reference gene sets (c2.cp.KEGG.v7.5.symbols.gmt and c5.all.v7.5.symbols.gmt) were selected for GSEA. The cut-off criteria were P<0.05 and a false discovery rate <0.05.
Tumor Immunity Estimation Resource (TIMER) website analysis
TIMER 2.0 (17) is a web server for the analysis of the gene expression differences between tumors and normal tissues as well as the association between gene expression and immune infiltration based on TCGA. We used the “Gene”, “sSNA”, and “Gene_Corr” modules in TIMER 2.0 to determine the correlation between SMS and immune cell infiltration levels in HNSC, including cluster of differentiation 4 (CD4)+ T cells, CD8+ T cells, B cells, neutrophils, monocytes, macrophages, and dendritic cells (DCs).
Construct prognosis-associated ceRNA network by starBase and Cytoscape
starBase (or The Encyclopedia of RNA Interactomes) is an open-license and state-of-the-art platform that decodes the cellular interaction networks among thousands of RNA-binding proteins and various RNAs (18). In the present study, starBase was applied to identify the interactions between SMS and miRNA/lncRNA the results were visualized in Cytoscape (version 3.9.1, National Resource for Network Biology, US) (19). Moreover, we used OS as the survival outcome measure to evaluated the prognostic value of prognosis-associated miRNA/lncRNA.
Statistical analysis
All data were analyzed using the R Project for Statistical Computing software (R software version 3.6.3, Vienna University of Economics and Business, Austria) and expressed as the mean ± standard deviation (SD). The expression levels of SMS, hsa-miR-23b-3p, KTN1-AS1, MSC-AS1, NEAT1, and VPS9D1-AS1 between HNSC patients and normal controls were compared using Wilcoxon signed-rank test. Spearman’s correlation analysis and the Cor test were used in the correlation analysis. The Student’s t-test was used to measure the associations between SMS and clinicopathological parameters. Statistical analysis of the survival data was performed using the survivor R package, Kaplan-Meier method, and log-rank test. Univariate and multivariate Cox regression analyses were used to verify the independent prognostic roles of SMS and the clinical parameters. The Wilcoxon and Kruskal-Wallis tests were also utilized to identify the potential correlation between SMS and clinical parameters. P<0.05 (two-sided) was considered statistically significant.
Results
SMS mRNA expression levels in different types of human cancer
We first analyzed the mRNA expression levels of SMS in human cancer using RNA-sequencing (RNA-seq) data from 33 malignancies in TCGA datasets. As shown in Figure 1A, SMS expression was remarkably upregulated in bladder urothelial carcinoma (BLCA), invasive breast carcinoma (BRCA), cholangiocarcinoma (CHOL), colon adenocarcinoma (COAD), esophageal carcinoma (ESCA), glioblastoma multiforme (GBM), HNSC, liver hepatocellular carcinoma (LIHC), lung adenocarcinoma (LUAD), lung squamous cell carcinoma (LUSC), prostate adenocarcinoma (PRAD), rectum adenocarcinoma (READ), stomach adenocarcinoma (STAD), and uterine corpus endometrial carcinoma (UCEC). SMS expression was downregulated in kidney chromophobe (KICH), kidney renal clear cell carcinoma (KIRC), kidney renal papillary cell carcinoma (KIRP), and thyroid carcinoma (THCA). Moreover, SMS expression between the paired HNSC tissues and adjacent normal tissues (n=44) was compared to validate the reliability of the result, and the result was the same (P<0.05, Figure 1B).
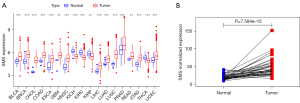
Prognostic potential of SMS in HNSC
The associations between SMS expression and the clinicopathological features in HNSC patients were investigated using the TCGA dataset. The results revealed that SMS expression was correlated with tumor grade (P=0.006) and N stage (P=0.001) but had no statistically significant associations with the patient’s age (P=0.6060, gender (P=0.315), clinical stage (P=0.085), T stage (P=0.64), or M stage (Figure 2A-2F). To further evaluate the prognostic value of SMS expression in HNSC, we used the GEPIA 2 web server to analyze the relationship between the SMS expression level and OS and DFS. The results revealed that a high level of SMS expression resulted in significantly poor OS (HR =1.4, P=0.01, Figure 2G) and DFS (HR =1.5, P=0.014, Figure 2H).
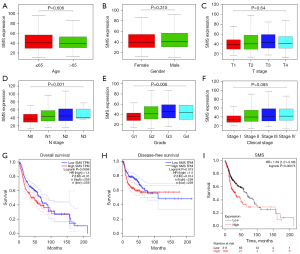
We also investigated the survival value of SMS expression using the Kaplan-Meier Plotter database. Similarly, we found that higher SMS expression levels could result in a worse HNSC prognosis (HR =1.59, P<0.001, Figure 2I). Furthermore, univariate and multivariate Cox regression analyses suggested that SMS expression and N stage were independent prognostic factors for OS based on TCGA dataset (P=0.0056, Table 1). Collectively, these results suggested that high SMS expression contributes to a poor prognosis in HNSC and may play an oncogenic role in HNSC.
Table 1
Parameter | Univariate analysis | Multivariate analysis | |||||
---|---|---|---|---|---|---|---|
HR | 95% CI | P | HR | 95% CI | P | ||
Age | 1.021135 | 0.99–1.04 | 0.10112 | 1.026693 | 0.99–1.05 | 0.056489 | |
N | 1.65793 | 1.25–2.2 | 0.000465 | 1.930864 | 1.26–2.95 | 0.002354 | |
T | 1.226154 | 0.92–1.63 | 0.157912 | 1.239913 | 0.81–1.89 | 0.317726 | |
Stage | 1.418897 | 1.01–1.99 | 0.043132 | 0.811608 | 0.43–1.52 | 0.516651 | |
Grade | 1.209063 | 0.79–1.84 | 0.377631 | 1.019248 | 0.66–1.58 | 0.932486 | |
Gender | 0.680628 | 0.38–1.20 | 0.186118 | 0.731207 | 0.40–1.33 | 0.307363 | |
SMS | 1.006548 | 1.00–1.01 | 0.005654 | 1.006636 | 1.00–1.01 | 0.005649 |
SMS, spermine synthase; OS, overall survival; HNSC, head and neck squamous cell carcinoma; HR, hazard ratio; CI, confidence interval.
Kyoto Encyclopedia of Genes and Genomes (KEGG) and Gene Ontology (GO) enrichment analyses of SMS in HNSC
GO and KEGG were used to analyze the expression phenotypes between high and low SMS expression through GSEA to further explore the SMS-related signaling pathways. We listed the top five statistically significant up- and down-regulated signal pathways in the high and low expression groups of SMS in the KEGG pathway analysis, including alpha-linolenic acid metabolism, B cell receptor signaling pathway, cell adhesion molecules, and the cell cycle (Figure 3A). In addition, GO analysis showed that the following biological functions were enriched: calcium-dependent phospholipase A2 activity, protein folding, and the regulation of T helper 17 type immune response (Figure 3B).
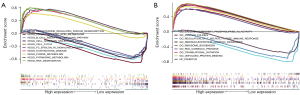
SMS expression is correlated with immune cell infiltration in HNSC
The TIMER 2.0 database was used to investigate the immune infiltration distribution of SMS genes with different somatic copy number alterations (sCNAs) to analyze whether SMS expression is associated with tumor-infiltrating lymphocytes in HNSC. As shown in Figure 4A,4B, the results showed statistically significant differences in B cells and CD8+ T cells. Next, we also analyzed the correlation between SMS expression and immune cell levels in HNSC. As shown in Figure 4C-4H, SMS expression levels were remarkably decreased in CD8+ T cells, B cells, neutrophils, macrophages, and DCs and increased in CD4+ T cells.
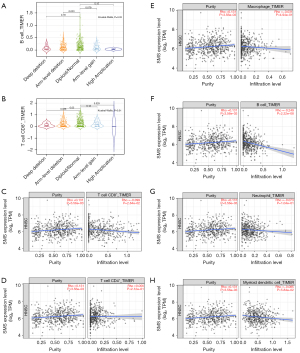
Additionally, using the TCGA datasets, we further analyzed the relationship between SMS expression and a set of marker genes of tumor infiltration-associated immune cells. The results revealed that SMS expression was negatively correlated with most of the immune marker genes in HNSC, including the B cell marker, CD4+ T cell marker, CD8+ T cell marker, M1 macrophage marker, neutrophil marker, and DC marker (Table 2). We also found that immune checkpoint Cytotoxic T-lymphocyte-associated protein 4 (CTLA4) expression exhibited the same negative correlation with SMS expression level through the analysis of GEPIA 2 and TIMER 2.0 (Figure 5). We speculated that SMS might be involved in immune-oncology interactions in HNSC.
Table 2
Immune cell | Marker | Cor | P value |
---|---|---|---|
B cell | CD19 | −0.13274547740586 | 0.0028825445645981 |
CD79A | −0.186898979717136 | 2.5893103505966e-05 | |
CD8+ T cell | CD8A | −0.180542102254427 | 4.86701270327416e-05 |
CD8B | −0.177024410206863 | 6.66477227142263e-05 | |
CD4+ T cell | CD4 | −0.233519369504755 | 1.31509158231199e-07 |
M1 macrophage | NOS2 | 0.00517969672712048 | 0.90783924944789 |
IRF5 | −0.137386383798979 | 0.00205102824600434 | |
PTGS2 | 0.096817809653281 | 0.0301223312041398 | |
M2 macrophage | CD163 | −0.0901240930967859 | 0.0435837371107234 |
VSIG4 | −0.0514783759688689 | 0.249522889406762 | |
MS4A4A | −0.0728675161379932 | 0.102935852615158 | |
Neutrophil | CEACAM8 | 0.0430829269765246 | 0.335382289922588 |
ITGAM | −0.212970287633172 | 1.55848686725463e-06 | |
CCR7 | −0.211288653317588 | 1.88870797400505e-06 | |
Dendritic cell | HLA-DPB1 | −0.213876746202651 | 1.40423632161715e-06 |
HLA-DQB1 | −0.170598187244119 | 0.000125268823059417 | |
HLA-DRA | −0.207969967726148 | 2.74751807115071e-06 | |
HLA-DPA1 | −0.217805934865792 | 8.89228392705508e-07 | |
CD1C | −0.329549869462991 | 3.50963444780664e-14 | |
NRP1 | −0.110233510310611 | 0.0135010539003642 | |
ITGAX | −0.0609071197264726 | 0.172979132438559 |
SMS, spermine synthase; HNSC, head and neck squamous cell carcinoma; TCGA, The Cancer Genome Atlas.
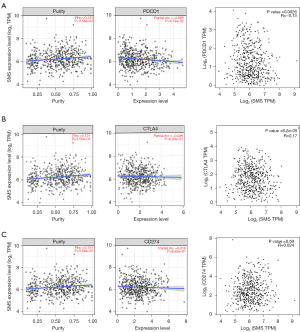
Potential miRNA-mediated SMS gene co-expression network in HNSC
MiRNA-SMS gene interactions were searched to predict the potential miRNAs that might play a regulatory role in the prognosis of HNSC using starBase based on the Crosslinking-immunoprecipitation and high-throughput sequencing (CLIP-seq) data. After the miRNA-SMS gene co-expression analysis and verification of the miRNA expression levels between tumors and normal tissues in TCGA datasets, we obtained the potential binding miRNAs of SMS in HNSC (Figure 6A) and found that only hsa-miR-23b-3p expression was negatively correlated to the SMS gene and was obviously downregulated in HNSC tissues compared to normal tissues (r=−0.21, P<0.001, Figure 6B,6C). Furthermore, survival analysis showed that the low levels of hsa-miR-23b-3p expression resulted in worse OS (P<0.001, Figure 6D).
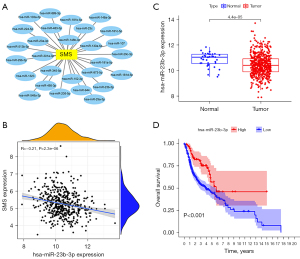
Construction of a SMS-related ceRNA network in HNSC
LncRNA inhibits the negative regulation of miRNA on mRNA by competing with miRNA to bind to the 3'-untranslated region of mRNA, resulting in the rescue of mRNAs from post-transcriptional silence (20). Therefore, starBase was used to obtain the target lncRNAs of hsa-miR-23b-3p. Based on our analysis of differentially expressed profiles, we found four potential lncRNA regulatory molecules. As shown in Figure 7A-7H, the expression levels of KTN1-AS1, MSC-AS1, NEAT1, and VPS9D1-AS1 were negatively associated with hsa-miR-23b-3p and positively associated with SMS (MSC-AS1 and NEAT1 were not statistically different). Similarly, the expression levels of KTN1-AS1, MSC-AS1, NEAT1, and VPS9D1-AS1 were re-upregulated in HNSC compared with normal group (NM) (P<0.05, Figure 7I-7L). This finding was supported by the Kaplan-Meier survival analysis of the OS probability in patients with HNSC who had high KTN1-AS1, MSC-AS1, and VPS9D1-AS1 expression versus those with low KTN1-AS1, MSC-AS1, and VPS9D1-AS1 expression (Figure 7M-7P). Subsequently, a mRNA-miRNA-lncRNA regulatory network related to the OS of HNSC was constructed using Cytoscape (Figure 7Q). In this network, KTN1-AS1 and VPS9D1-AS seemed to express a greater potential as key prognosis-related lncRNAs that participate in HNSC progression.
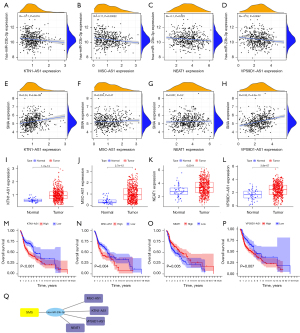
Discussion
Recent evidence has shown that SMS plays an important role in Snyder-Robinson syndrome (21,22), and is also involved in colorectal cancer through the convergent repression of Bim expression (8). However, the role of SMS in other malignant tumors, including HNSC, has not yet been elucidated. Therefore, exploring the effect of SMS in HNSC is urgently needed.
In this study, we demonstrated the oncogenic relevance of SMS in HNSC using a series of logical and comprehensive approaches. First, we performed a pan-cancer analysis of SMS expression in TCGA. The results revealed that, compared with normal tissues, SMS was remarkably upregulated in BLCA, BRCA, CHOL, COAD, ESCA, GBM, HNSC, LIHC, LUAD, LUSC, PRAD, READ, STAD, and UCEC and downregulated in KICH, KIRC, KIRP, and THCA. Using different databases, we found that the SMS expression levels differed among different tumor types, which may be due to the data collection method and the different biological characteristics of SMS. However, in these databases, we identified a consistency in the SMS expression in HNSC. Second, through survival curve analysis using GEPIA 2 and Kaplan-Meier, we further noted that SMS expression was remarkably correlated with a worse tumor grade, N stage, and OS/DFS in patients with HNSC.
GO and KEGG enrichment analyses were performed to explore the function and molecular mechanism of SMS. We identified that SMS was involved in metabolism-related and immunity-related pathways. A previous study confirmed that HNSC is characterized by immunosuppression (23). Therefore, we further focused on gaining insights into SMS to investigate tumor immune infiltration. We found that the sCNA status of SMS changed and could reduce the immune infiltration levels of B cells and CD8+ T cells. Moreover, we analyzed the correlation between SMS expression and changed immune cell expression levels and identified negative correlations in CD8+ T cells, B cells, neutrophils, macrophages, and DCs and a positive correlation in CD4+ T cells. Furthermore, the expressions of genetic markers of B cells, CD4+ T cells, CD8+ T cells, M1 macrophages, neutrophils, and DCs, as well as the immune checkpoint CTLA4, were negatively correlated with SMS expression. Collectively, these results highlight the importance of SMS in regulating immune-oncology interactions in HNSC.
Emerging evidence has indicated that large proportions of miRNAs and lncRNAs exhibit tumor-suppressing or tumor-promoting impacts in diverse cancers via oncogenesis, apoptosis, and cell growth (24-27). Herein, we constructed co-expression networks in HNSC using starBase and performed correlation and survival analyses of SMS, miRNAs, and lncRNAs. The results showed that hsa-miR-23b-3p was negatively correlated with SMS and was lowly expressed in HNSC; a lower expression of hsa-miR-23b-3p indicated a worse OS in patients with HNSC. Previous studies demonstrated that hsa-miR-23b-3p exerts carcinogenic functions in different types of cancers, including renal, gastric, and prostate cancers (28-31).
LncRNAs can competitively interact with miRNAs. In the present study, we also identified that hsa-miR-23b-3p might target four lncRNAs in HNSC, namely, KTN1-AS1, MSC-AS1, NEAT1, and VPS9D1-AS1. Accordingly, KTN1-AS1 and VPS9D1-AS1 expression was positively correlated with SMS, and the overexpression of KTN1-AS1 and VPS9D1-AS1 leads to a worse OS in HNSC patients. A previous study pointed out that KTN1-AS1 results in a poor prognosis in non-small cell lung cancer via the hsa-miR-23b/DEPDC1 axis (32). KTN1-AS1 and VPS9D1-AS1 have high expressions and promote tumorigenesis in several cancers (33-36). Collectively, we supposed that the lncRNAs, KTN1-AS1 and VPS9D1-AS1, may promote HNSC progression by sponging hsa-miR-23b-3p to upregulate SMS expression in HNSC.
The present study has some limitations that should be noted. Firstly, this study was only based on TCGA datasets; therefore, the value of SMS as an oncogene in HNSC needs to be verified by in vitro and in vivo experiments. Second, the mechanism of SMS involvement in immune infiltration and the potential ceRNA network need to be further explored. Despite these limitations, our study showed for the first time that SMS might serve as a potential prognosis biomarker for HNSC. Furthermore, SMS may also play an important role in immune cell infiltration. We also revealed that the SMS/hsa-miR-23b-3p/KTN1-AS1 and VPS9D1-AS axis could provide new insights into HNSC treatment.
Acknowledgments
We acknowledge and appreciate our colleagues for their valuable efforts and comments on this paper.
Funding: None.
Footnote
Reporting Checklist: The authors have completed the REMARK reporting checklist. Available at https://atm.amegroups.com/article/view/10.21037/atm-22-5014/rc
Conflicts of Interest: All authors have completed the ICMJE uniform disclosure form (available at https://atm.amegroups.com/article/view/10.21037/atm-22-5014/coif). The authors have no conflicts of interest to declare.
Ethical Statement: The authors are accountable for all aspects of the work in ensuring that questions related to the accuracy or integrity of any part of the work are appropriately investigated and resolved. The study was conducted in accordance with the Declaration of Helsinki (as revised in 2013).
Open Access Statement: This is an Open Access article distributed in accordance with the Creative Commons Attribution-NonCommercial-NoDerivs 4.0 International License (CC BY-NC-ND 4.0), which permits the non-commercial replication and distribution of the article with the strict proviso that no changes or edits are made and the original work is properly cited (including links to both the formal publication through the relevant DOI and the license). See: https://creativecommons.org/licenses/by-nc-nd/4.0/.
References
- Miller KD, Nogueira L, Devasia T, et al. Cancer treatment and survivorship statistics, 2022. CA Cancer J Clin 2022;72:409-36. [Crossref] [PubMed]
- Cohen EE, LaMonte SJ, Erb NL, et al. American Cancer Society Head and Neck Cancer Survivorship Care Guideline. CA Cancer J Clin 2016;66:203-39. [Crossref] [PubMed]
- Bhat GR, Hyole RG, Li J. Head and neck cancer: Current challenges and future perspectives. Adv Cancer Res 2021;152:67-102. [Crossref] [PubMed]
- Li LX, Wu JX, Huang W. The Prognostic Function of Biomarkers in Head and Neck Squamous Cell Carcinomas (HNSCC). Available online: http://www.cancerbio.net/cb/cb0101/07_1380cb0101_51_60.pdf
- Young RJ. Investigating prognostic and predictive tissue biomarkers in head and neck squamous cell carcinoma. Available online: https://minerva-access.unimelb.edu.au/items/699df749-1e4a-51d0-9312-65a0adc8d051
- de Alencastro G, McCloskey DE, Kliemann SE, et al. New SMS mutation leads to a striking reduction in spermine synthase protein function and a severe form of Snyder-Robinson X-linked recessive mental retardation syndrome. J Med Genet 2008;45:539-43. [Crossref] [PubMed]
- Qazi TJ, Wu Q, Aierken A, et al. Whole-exome sequencing identifies a novel mutation in spermine synthase gene (SMS) associated with Snyder-Robinson Syndrome. BMC Med Genet 2020;21:168. [Crossref] [PubMed]
- Guo Y, Ye Q, Deng P, Cao Y, et al. Spermine synthase and MYC cooperate to maintain colorectal cancer cell survival by repressing Bim expression. Nat Commun 2020;11:3243. [Crossref] [PubMed]
- Fahrmann JF, Vykoukal J, Fleury A, et al. Association Between Plasma Diacetylspermine and Tumor Spermine Synthase With Outcome in Triple-Negative Breast Cancer. J Natl Cancer Inst 2020;112:607-16. [Crossref] [PubMed]
- Thomas T, Thomas TJ. Polyamine metabolism and cancer. J Cell Mol Med 2003;7:113-26. [Crossref] [PubMed]
- Zhang X, Shi M, Chen T, et al. Characterization of the Immune Cell Infiltration Landscape in Head and Neck Squamous Cell Carcinoma to Aid Immunotherapy. Mol Ther Nucleic Acids 2020;22:298-309. [Crossref] [PubMed]
- Zhang F, Liu Y, Yang Y, et al. Development and validation of a fourteen- innate immunity-related gene pairs signature for predicting prognosis head and neck squamous cell carcinoma. BMC Cancer 2020;20:1015. [Crossref] [PubMed]
- Goldman MJ, Craft B, Hastie M, et al. Visualizing and interpreting cancer genomics data via the Xena platform. Nat Biotechnol 2020;38:675-8. [Crossref] [PubMed]
- Tang Z, Li C, Kang B, et al. GEPIA: a web server for cancer and normal gene expression profiling and interactive analyses. Nucleic Acids Res 2017;45:W98-W102. [Crossref] [PubMed]
- Nagy Á, Munkácsy G, Győrffy B. Pancancer survival analysis of cancer hallmark genes. Sci Rep 2021;11:6047. [Crossref] [PubMed]
- Subramanian A, Tamayo P, Mootha VK, et al. Gene set enrichment analysis: a knowledge-based approach for interpreting genome-wide expression profiles. Proc Natl Acad Sci U S A 2005;102:15545-50. [Crossref] [PubMed]
- Li T, Fu J, Zeng Z, et al. TIMER2.0 for analysis of tumor-infiltrating immune cells. Nucleic Acids Res 2020;48:W509-14. [Crossref] [PubMed]
- Li JH, Liu S, Zhou H, et al. starBase v2.0: decoding miRNA-ceRNA, miRNA-ncRNA and protein-RNA interaction networks from large-scale CLIP-Seq data. Nucleic Acids Res 2014;42:D92-7. [Crossref] [PubMed]
- Saito R, Smoot ME, Ono K, et al. A travel guide to Cytoscape plugins. Nat Methods 2012;9:1069-76. [Crossref] [PubMed]
- Lu W, Zhang H, Niu Y, et al. Long non-coding RNA linc00673 regulated non-small cell lung cancer proliferation, migration, invasion and epithelial mesenchymal transition by sponging miR-150-5p. Mol Cancer 2017;16:118. [Crossref] [PubMed]
- Pegg AE, Michael AJ. Spermine synthase. Cell Mol Life Sci 2010;67:113-21. [Crossref] [PubMed]
- Murray-Stewart T, Dunworth M, Foley JR, et al. Polyamine Homeostasis in Snyder-Robinson Syndrome. Med Sci (Basel) 2018;6:112. [Crossref] [PubMed]
- Romano E, Romero P. The therapeutic promise of disrupting the PD-1/PD-L1 immune checkpoint in cancer: unleashing the CD8 T cell mediated anti-tumor activity results in significant, unprecedented clinical efficacy in various solid tumors. J Immunother Cancer 2015;3:15. [Crossref] [PubMed]
- Balch C, Nephew KP. The role of chromatin, microRNAs, and tumor stem cells in ovarian cancer. Cancer Biomark 2010-2011;8:203-21. [Crossref] [PubMed]
- Bai Y, Zhou X, Huang L, et al. Long noncoding RNA EZR-AS1 promotes tumor growth and metastasis by modulating Wnt/β-catenin pathway in breast cancer. Exp Ther Med 2018;16:2235-42. [Crossref] [PubMed]
- Zhang W, Cai X, Yu J, et al. Exosome-mediated transfer of lncRNA RP11-838N2.4 promotes erlotinib resistance in non-small cell lung cancer. Int J Oncol 2018;53:527-38. [Crossref] [PubMed]
- Geisler S, Coller J. RNA in unexpected places: long non-coding RNA functions in diverse cellular contexts. Nat Rev Mol Cell Biol 2013;14:699-712. [Crossref] [PubMed]
- Grossi I, Salvi A, Baiocchi G, et al. Functional Role of microRNA-23b-3p in Cancer Biology. Microrna 2018;7:156-66. [Crossref] [PubMed]
- Li G, Zhang Z, Chen Z, et al. LncRNA DLEU2 is activated by STAT1 and induces gastric cancer development via targeting miR-23b-3p/NOTCH2 axis and Notch signaling pathway. Life Sci 2021;277:119419. [Crossref] [PubMed]
- Zaman MS, Thamminana S, Shahryari V, et al. Inhibition of PTEN gene expression by oncogenic miR-23b-3p in renal cancer. PLoS One 2012;7:e50203. [Crossref] [PubMed]
- Zhou C, Chen Y, He X, et al. Functional Implication of Exosomal miR-217 and miR-23b-3p in the Progression of Prostate Cancer. Onco Targets Ther 2020;13:11595-606. [Crossref] [PubMed]
- Liu C, Li X, Hao Y, et al. STAT1-induced upregulation of lncRNA KTN1-AS1 predicts poor prognosis and facilitates non-small cell lung cancer progression via miR-23b/DEPDC1 axis. Aging (Albany NY) 2020;12:8680-701. [Crossref] [PubMed]
- Liu H, Zhang X, Jin X, et al. Long Noncoding RNA VPS9D1-AS1 Sequesters microRNA-525-5p to Promote the Oncogenicity of Colorectal Cancer Cells by Upregulating HMGA1. Cancer Manag Res 2020;12:9915-28. [Crossref] [PubMed]
- Wang X, Chen Q, Wang X, et al. ZEB1 activated-VPS9D1-AS1 promotes the tumorigenesis and progression of prostate cancer by sponging miR-4739 to upregulate MEF2D. Biomed Pharmacother 2020;122:109557. [Crossref] [PubMed]
- Hu X, Xiang L, He D, et al. The long noncoding RNA KTN1-AS1 promotes bladder cancer tumorigenesis via KTN1 cis-activation and the consequent initiation of Rho GTPase-mediated signaling. Clin Sci (Lond) 2021;135:555-74. [Crossref] [PubMed]
- Li C, Zhao W, Pan X, et al. LncRNA KTN1-AS1 promotes the progression of non-small cell lung cancer via sponging of miR-130a-5p and activation of PDPK1. Oncogene 2020;39:6157-71. [Crossref] [PubMed]
(English Language Editor: A. Kassem)