Insufficient sleep disrupts glucose metabolism during pregnancy by inhibiting PGC-1α
Highlight box
Key findings
• This study reports increased blood glucose and insulin resistance, downregulation of energy metabolism and antioxidant genes, and metabolic disruption were all signs of sleep disturbance-induced GDM. In sleep-deprived pregnant mice, activating
What is known and what is new?
• Risk of sleep disorder increases gestational diabetes, hypertension,
• The mechanism of how sleep disturbance induces gestational diabetes is not yet clear.
What is the implication, and what should change now?
• This study is aimed to yield evidence that PGC-1α serves as the connection between sleep loss and GDM.
Introduction
Gestational diabetes mellitus (GDM) is typically first diagnosed during weeks 24–28 of pregnancy, which affects approximately 17 million pregnancies worldwide each year (1,2). Due to the maternal need for higher caloric intake and elevated insulin resistance, glycemic control during pregnancy can be challenging. Maternal hyperglycemia accelerates intrauterine growth and increases the risk of macrosomia or the fetus being born large for gestational age (3), which may increase the risk of preeclampsia, neonatal hypoglycemia, shoulder dystocia, late stillbirth, or the need for caesarean section or neonatal intensive care (4-6). Moreover, maternal hyperglycemia can cause abnormal carbohydrate metabolism beyond the second generation (7-9). It has been shown that GDM modifies neurodevelopmental processes resulting in learning disability, attention deficit, and motor impairment in children (10-12) and rodents (13-15).
Sleep disturbance is implicated in numerous health problems including diabetes, cerebrovascular disease, cardiovascular disease, malignant neoplasm, accidents, septicemia, and hypertension (16). Shortened and fragmented sleeping patterns may cause metabolic disturbances resulting in an increased risk of weight gain and skeletal muscle degradation. The metabolic damage may stimulate systemic inflammation, insulin resistance, and suppress muscle protein synthesis, which may disrupt energy balance and skeletal muscle metabolism (17). Poor sleep quality during early pregnancy significantly increases the risk of GDM, especially in women aged 30 years and above (18,19). Abnormally long or short sleep duration increases random blood glucose levels in pregnant women and a duration sleep greater than 10 hours per night significantly increases the risk for GDM (20). Furthermore, gestational sleep deprivation is associated with higher offspring body mass index (BMI), diastolic blood pressure, and waist circumference, and increases the risk for overweight or obesity (21).
Peroxisome proliferator-activated receptor gamma co-activator 1 alpha (PPARGC1A or short form PGC-1α), the master regulator of mitochondrial biogenesis and energy production, is important in controlling many cellular pathways, including metabolism, energy homeostasis, insulin sensitivity, oxidative state, and inflammation (22-26). The messenger RNA (mRNA) levels of PGC-1α mRNA on the fetal side of the placenta from GDM mothers were found to be significantly lower compared to the control group, whereas the methylation level of the PGC-1α gene and blood glucose level were comparatively higher in the GDM group (27). Emerging evidence indicates that sleep loss inhibits PGC-1α expression, reduces mitochondrial function, and subsequently increases insulin resistance (28). However, the mechanism of how sleep disturbance induces gestational diabetes is not yet clear. This study is aimed to yield evidence that PGC-1α serves as the connection between sleep loss and GDM. We present the following article in accordance with the ARRIVE reporting checklist (available at https://atm.amegroups.com/article/view/10.21037/atm-22-5551/rc).
Methods
Gestational sleep disturbance mouse model
Adult B57bl/6 mice (7 weeks of age) were purchased from Cavens Laboratory Animals (Changzhou, China) and acclimated for a week before mating. Every male mouse was housed with 3 female mice in each cage. Mice were housed at 22–24 ℃ with 50% humidity, a 12-hour light/dark cycle, and free access to water and standard chow (000521; Zeyakejiao, Shanghai, China). To initiate pregnancy, every male mouse was housed in a cage with 3 female mice in the evening and the copulatory plug was checked the next morning. Mated female mice were randomly divided into a control group (5 mice) and a sleep disturbance group (15 mice) and the mice in both groups were allowed to eat freely.
Sleep disturbance was performed in rodent sleep deprivation machine (KW-BD; KEW Basis Biotechnologies, Nanjing, China) from 8:00 am to 6:00 pm daily with the disturbing rod set at 1 rpm. Body weight and water consumption were recorded daily. Fasting blood glucose level and intraperitoneal glucose tolerance were measured after 5 days of sleep disturbance. The mice with higher blood glucose level and glucose intolerance were randomly divided into 2 groups to receive either 50 µL of 0.5% dimethyl sulfoxide (DMSO) or ZLN005 (15 mg/kg in 50 µL of 0.5% DMSO) (S7447, Selleck Chemicals, Houston, TX, USA) daily via gavage for 7 days. The speed of sleep deprivation machine was reduced to 0.1 rpm during this period of time. At the end of the treatment regime, blood was collected via retro-orbital bleeding before mice were euthanized and the gastrocnemius and soleus muscles were harvested.
Experiments were performed under a project license (No. 2020KY235) granted by Institutional Animal Care and Use Committee (IACUC) of Nanjing Medical University, in compliance with national guidelines for the care and use of animals.
Intraperitoneal glucose tolerance test
The intraperitoneal glucose tolerance test (IGTT) was performed essentially following a previously published procedure (29). Briefly, mice were fasted for 12 hours and given 2 g/kg (of body weight) glucose (400 mg/mL) solution via intraperitoneal injection. Blood glucose levels at 0, 15, 30, 60, and 120 minutes after glucose injection were measured with a glucometer (One Touch Ultra2, LifeScan, Shanghai, China).
Enzyme-linked immunosorbent assay
Serum PGC-1α (JL49160, Jianglai Bio, Shanghai, China), interleukin (IL)-1β (JYM0531Mo, ColorfulGene, Wuhan, China), TNF-α (JYM0218Mo, ColorfulGene), IL-6 (JYM0012Mo; ColorfulGene), and insulin (SEKM0141, SolarBio, Beijing, China) levels were assessed with an enzyme-linked immunosorbent assay (ELISA) commercial kit according to the manufacturer’s protocols.
Real-time polymerase chain reaction (RT-PCR)
Total RNA was extracted with Trizol (Invitrogen, Shanghai, China) and then 0.5 µg was reverse transcribed using RevertAid First Strand cDNA Synthesis Kit (K1622, Thermo Fisher Scientific, Shanghai, China). The relative mRNA levels of the studied gene were analyzed by RT-PCR using DyNAmo Flash SYBR Green qPCR Kit (F415XL, Thermo Fisher Scientific) on an ABI 7500 (Thermo Fisher Scientific). The sequences of primers used in this study are listed in Table 1. The relative gene expression level was calculated by 2-∆∆Ct method with glyceraldehyde 3-phosphate dehydrogenase (GAPDH) as the internal control.
Table 1
Primer | Sequence |
---|---|
Pgc1α(Mouse)-F | CAGGAACAGCAGCAGAGACAAAT |
Pgc1α(Mouse)-R | TGGGGTCAGAGGAAGAGATAAAG |
Errα(Mouse)-F | CGGCGGACGGCAGAAGTACAAAC |
Errα(Mouse)-R | CCACCAGCAGATGCGACACCAGA |
Sirt1(Mouse)-F | TCAGATAAGGAAGGAAAACTAC |
Sirt1(Mouse)-R | GATTAAAAATGTCTCCACGAAC |
Glut4(Mouse)-F | CTTCCTTCTATTTGCCGTCCTCC |
Glut4(Mouse)-R | ACTGGGTTTCACCTCCTGCTCTA |
Sod2(Mouse)-F | AGCGTGACTTTGGGTCTTTTGAG |
Sod2(Mouse)-R | GGAATAAGGCCTGTTGTTCCTTG |
Ucp2(Mouse)-F | CATTGTCAACTGTGCTGAGCTG |
Ucp2(Mouse)-R | AGAAGTGAAGTGGCAAGGGAGG |
Gpx1(Mouse)-F | GAGAAGTGCGAAGTGAATGGTGAGA |
Gpx1(Mouse)-R | CAAAGTTCCAGGCAATGTCGTTGCG |
Tfam(Mouse)-F | CAAAGTTCCAGGCAATGTCGTTGCG |
Tfam(Mouse) -R | TTTTTCTGCTTCTGGTAGCTCCCTC |
mt-Nd1(Mouse)-F | ACATTGTTGGTCCATACGGCATTTT |
mt-Nd1(Mouse)-R | TATTGGTAGGGGAACTCATAGACTT |
mt-Cox1(Mouse)-F | GAGTGTCATCTATTTTAGGTGC |
mt-Cox1(Mouse)-R | AAAGTTGTGTTTAGGTTGCGGT |
mt-Atp6(Mouse)-F | CACACACCAAAAGGACGAACAT |
mt-Atp6(Mouse)-R | AATTACGGCTCCAGCTCATAGT |
Gapdh(Mouse)-F | CCTTCCGTGTTCCTACCCCCAATGTG |
Gapdh(Mouse)-R | TCGCAGGAGACAACCTGGTCCTCAGT |
COX IV(Mouse)-F | CTATGTGTATGGCCCCATCCCTC |
COX IV(Mouse)-R | CACTTCTTCCACTCATTCTTGTC |
Measurement of adenosine triphosphate (ATP) level
The ATP levels of muscle tissues were measured with an ATP content assay kit (BC0300, SolarBio) according to manufacturer’s instructions and normalized to protein content.
Histopathological staining
The histopathological changes of mouse muscle tissue were examined by Oil red O (G1261, SolarBio) and hematoxylin and eosin (HE; ab245880, Abcam, Cambridge, MA) staining following the manufacturer’s procedures.
Immunohistochemical staining
Slides of mouse gastrocnemius and soleus muscle tissues were deparaffinized by immersing in xylene for 30 minutes, followed by another round in xylene for 10 minutes, and then rehydrated in ethanol alcohol gradient (100% to 50%) to phosphate-buffered saline (PBS). After performing antigen retrieval, quenching endogenous peroxidase activity, and blocking with 5% bovine serum albumin (BSA), slides were incubated with primary antibodies at 4 ℃ overnight. Antibodies used were anti-GLUT4 (bs-0384R, Bioss, Woburn, MA, USA), PGC-1α (66369-1-AP, Proteintech, Wuhan, China), TFAM (22586-1-AP, Proteintech), and UCP2 (11081-1-AP, Proteintech). The slides were washed and incubated with appropriate horseradish peroxidase (HRP)-conjugated secondary antibody (PV-9001 or PV-9002, ZSGB Bio, Beijing, China) at room temperature for 30 minutes, washed 3 times, incubated with DAB Chromogenic Solution (ZLI-9017, ZSGB Bio) and counterstained with hematoxylin (170103, Shanghai Zhanyun Chemicals, Shanghai, China).
Reactive oxygen species (ROS) assessment
ROS levels in mouse muscle tissues were assessed using a Green Fluorescence Test Kit for Oxidative Stress ROS in Living Tissues (GMS10016.3, GENMED Scientifics, Shanghai, China) following the protocol provided by the manufacturer.
Western blot
Mouse muscle tissues were ground in liquid nitrogen and lysed in radioimmunprecipitation assay (RIPA) buffer (Beyotime, Shanghai, China) with 1 mM phenylmethylsulfonyl fluoride (PMSF; P7626, Sigma, St. Louis, MO, USA). Then, 25 µg total protein was resolved in 8% polyacrylamide gel and transferred onto polyvinylidene fluoride membranes (HATF00010, Millipore, Shanghai, China). The membranes were blocked with 5% BSA for 2 hours at room temperature (A9647, Biosharp, Hefei, China), incubated with specified primary antibodies at 4 ℃ overnight, washed, and incubated with HRP-conjugated secondary antibodies at room temperature for 1 hour before visualization with enhanced chemiluminescence (ECL) working solution (ECL-0011, Beijing Dingguo Changsheng Biotech, Beijing, China).
Statistical analysis
Statistical analysis was performed with GraphPad 12 (GraphPad Software, San Diego, CA, USA). Data were expressed as mean ± SD. The differences among groups were analyzed using one-way analysis of variance (ANOVA) or t-test. A P value less than 0.05 was considered statistically significant.
Results
Sleep disturbance promoted GDM
To examine the effects of sleep disturbance on gestational health, newly mated female mice were subjected to motion-induced sleep disturbance. Although sleep disturbance did not have any effect on their body weight (Figure 1A), it induced a significant increase of fasting blood glucose level (Figure 1B) and insulin resistance (Figure 1C).
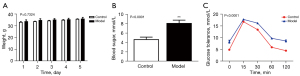
The levels of PGC-1α and its downstream targets were affected by sleep disturbance
As PGC-1α is a master regulator of metabolism and plays a critical role in insulin resistance (29), we next tested the hypothesis that sleep disturbance causes the perturbation of PGC-1α-regulated metabolic and antioxidant pathways which leads to gestational diabetes. PGC-1α expression was significantly down regulated by sleep disturbance in mouse gastrocnemius (Figure 2A,2B) and soleus (Figure 2C,2D) muscles. Sleep disturbance induced the inhibition of a wide range of PGC-1α target genes including Erra, SOD2, UCP2, GPx1, Tfam, and others in mouse muscle tissue (Figure 2A-2E). Treating sleep disturbed mice with PGC-1α transcriptional activator ZLN005 substantially increased both mRNA (Figure 2A,2C) and protein (Figure 2B,2D,2E) levels of PGC-1α and its target genes in mouse muscle tissues.
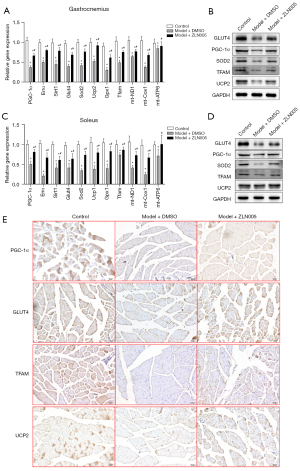
PGC-1α activation attenuated sleep disturbance-induced oxidative stress and inflammation
Sleep disturbance caused elevation of ROS level in the gastrocnemius and soleus muscles of pregnant mice (Figure 3A). Meanwhile, serum levels of inflammatory cytokines IL-1β, IL-6, and TNF-α were significantly increased in sleep disturbed mice (Figure 3B). Treating sleep disturbed pregnant mice with ZLN005 markedly reduced ROS level (Figure 3A) and inflammation (Figure 3B).
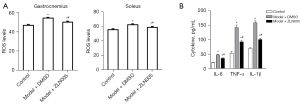
PGC-1α mediated the effects of sleep disturbance on tissue ATP levels
We next assessed whether sleep disturbance impacted muscle tissue ATP contents and if and PGC-1α played a role in this process. Sleep disturbance caused a significant decrease of the ATP level of the gastrocnemius and soleus muscles of pregnant mice (Figure 4). ZLN005 treatment substantially relieved sleep disturbance-induced reduction of ATP contents in the muscle tissues of pregnant mice (Figure 4).
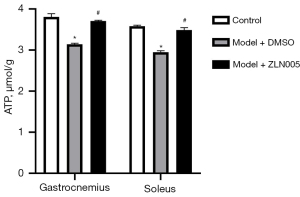
Sleep disturbance-induced metabolic abnormality was reversed by PGC-1α activation
Sleep disturbance significantly increased the blood glucose (Figure 5A), serum insulin (Figure 5B), and glucose resistance (Figure 5C) levels of pregnant mice. ZLN005 treatment lowered blood glucose levels by about 30% (Figure 5A) and serum insulin level by around 20% (Figure 5B) compared to those of sleep disturbed pregnant mice receiving DMSO. Moreover, the blood glucose level receded much faster from the peak in IGTT in ZLN005 treated mice than sleep disturbed pregnant mice without ZLN005 (Figure 5C).
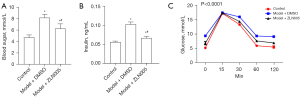
ZLN005 reduced lipid accumulation in muscle tissue of sleep disturbed pregnant mice
Sleep disturbance did not obviously change the histology of the gastrocnemius and soleus muscles of pregnant mice (Figure 6A). However, Oil red O staining showed that sleep disturbance significantly increased lipid droplets in the muscles of pregnant mice and this increase was partially inhibited by ZLN005 treatment (Figure 6B).
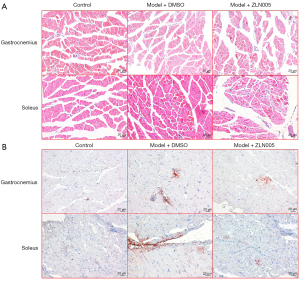
Discussion
Sleep disturbance of gestational mice induced a significant increase of blood glucose level and insulin resistance, accompanied by an increase of inflammatory cytokines, elevation of oxidative stress, and reduction of ATP production in muscle tissues, downregulation of PGC-1α, and a range of PGC-1α-regulated genes. Treating sleep disturbed pregnant mice with the PGC-1α transcriptional activator ZLN005 relieved the inhibition of expression of PGC-1α and its target genes in mouse muscle affected by sleep disturbance, which inhibited ROS production and inflammation and increased ATP level. These changes eventually led to improvement of gestational glucose metabolism.
Sleep disturbance resulted in higher blood glucose levels and higher insulin resistance in pregnant mice, which was consistent with the trends in pregnant women (30). Poor sleep quality and extreme sleep duration increased the risk of GDM (31). Objective assessment found that short sleep duration and a later sleep midpoint significantly increased the risk of GDM (32). Similarly, short or long sleep duration incrementally increased the risk of type 2 diabetes (33,34). Sleep duration, slow wave sleep suppression, and rapid eye movement sleep were shown to be associated with insulin resistance in children and adolescents (35). Taken together, sleep duration and sleep quality were associated with the risk of GDM and type 2 diabetes in general population.
Dysregulation of mitochondrial function and downregulation of glucose transporter (GLUT4) are main factors of insulin resistance (36) and PGC-1α has been identified as a master regulator of metabolism (22). Studies have shown that the expression level of PGC-1α in energy-consuming tissues of diabetic cases is significantly reduced (22,36). Similarly, PGC-1α expression in the skeletal muscle tissues of pregnant mice was inhibited by sleep disturbance, which led to downregulation of genes involved in energy metabolism and antioxidization, and increase of blood glucose level and insulin resistance. These adverse effects of sleep disturbance in pregnant mice were suppressed by PGC-1α activator ZLN005 (37), confirming the roles of PGC-1α in mediating metabolism disruption caused by sleep disturbance.
Conclusions
In summary, sleep disturbance-induced GDM was evidenced by increased blood glucose and insulin resistance, downregulation of energy metabolism and antioxidant genes, and perturbation of metabolism. Activating PGC-1α with ZLN005 in sleep disturbed pregnant mice promoted expression of PGC-1α and its target genes, increased muscle ATP level, decreased muscle ROS, and reduced blood glucose level and insulin resistance. These data indicated that PGC-1α played a critical role in sleep insufficiency caused GDM and might be a target for intervention.
Acknowledgments
We deeply appreciate the support of all participants.
Funding: This work was supported by the Major Clinical Research Project for the Second Round of Shanghai Shenkang Three-year Action Plan to Promote Clinical Skills and Innovation in Municipal Hospitals (No. SHDC2020CR2060B).
Footnote
Reporting Checklist: The authors have completed the ARRIVE reporting checklist. Available at https://atm.amegroups.com/article/view/10.21037/atm-22-5551/rc
Data Sharing Statement: Available at https://atm.amegroups.com/article/view/10.21037/atm-22-5551/dss
Conflicts of Interest: All authors have completed the ICMJE uniform disclosure form (available at https://atm.amegroups.com/article/view/10.21037/atm-22-5551/coif). The authors have no conflicts of interest to declare.
Ethical Statement: The authors are accountable for all aspects of the work in ensuring that questions related to the accuracy or integrity of any part of the work are appropriately investigated and resolved. Experiments were performed under a project license (No. 2020KY235) granted by Institutional Animal Care and Use Committee (IACUC) of Nanjing Medical University, in compliance with national guidelines for the care and use of animals.
Open Access Statement: This is an Open Access article distributed in accordance with the Creative Commons Attribution-NonCommercial-NoDerivs 4.0 International License (CC BY-NC-ND 4.0), which permits the non-commercial replication and distribution of the article with the strict proviso that no changes or edits are made and the original work is properly cited (including links to both the formal publication through the relevant DOI and the license). See: https://creativecommons.org/licenses/by-nc-nd/4.0/.
References
- Champion ML, Battarbee AN, Biggio JR, et al. Postpartum glucose intolerance following early gestational diabetes mellitus. Am J Obstet Gynecol MFM 2022;4:100609. [Crossref] [PubMed]
- Messika A, Toledano Y, Hadar E, et al. Relationship among chrononutrition, sleep, and glycemic control in women with gestational diabetes mellitus: a randomized controlled trial. Am J Obstet Gynecol MFM 2022;4:100660. [Crossref] [PubMed]
- Owen MD, Baker BC, Scott EM, et al. Interaction between Metformin, Folate and Vitamin B12 and the Potential Impact on Fetal Growth and Long-Term Metabolic Health in Diabetic Pregnancies. Int J Mol Sci 2021;22:5759. [Crossref] [PubMed]
- Kul Ş, Güvenç TS, Baycan ÖF, et al. Combined past preeclampsia and gestational diabetes is associated with a very high frequency of coronary microvascular dysfunction. Microvasc Res 2021;134:104104. [Crossref] [PubMed]
- Pathirana MM, Ali A, Lassi ZS, et al. Protective Influence of Breastfeeding on Cardiovascular Risk Factors in Women With Previous Gestational Diabetes Mellitus and Their Children: A Systematic Review and Meta-Analysis. J Hum Lact 2022;38:501-12. [Crossref] [PubMed]
- Vetterlein J, Doehmen CAE, Voss H, et al. Antenatal risk prediction of shoulder dystocia: influence of diabetes and obesity: a multicenter study. Arch Gynecol Obstet 2021;304:1169-77. [Crossref] [PubMed]
- Jiang Y, Zhu H, Chen Z, et al. Hepatic IGF2/H19 Epigenetic Alteration Induced Glucose Intolerance in Gestational Diabetes Mellitus Offspring via FoxO1 Mediation. Front Endocrinol (Lausanne) 2022;13:844707. [Crossref] [PubMed]
- Lee KW, Tan SF, Omar A, et al. Effectiveness of system-based intervention in reducing incidence of type 2 diabetes and to improve the postnatal metabolic profiles in women with gestational diabetes mellitus: a randomized controlled study. Gynecol Endocrinol 2022;38:55-62. [Crossref] [PubMed]
- Liu Y, Kuang A, Bain JR, et al. Maternal Metabolites Associated With Gestational Diabetes Mellitus and a Postpartum Disorder of Glucose Metabolism. J Clin Endocrinol Metab 2021;106:3283-94. [Crossref] [PubMed]
- Nielsen GL, Andersen E, Lundbye-Christensen S. Maternal blood glucose in diabetic pregnancies and cognitive performance in offspring in young adulthood: a Danish cohort study. Diabet Med 2010;27:786-90. [Crossref] [PubMed]
- Nomura Y, Marks DJ, Grossman B, et al. Exposure to gestational diabetes mellitus and low socioeconomic status: effects on neurocognitive development and risk of attention-deficit/hyperactivity disorder in offspring. Arch Pediatr Adolesc Med 2012;166:337-43. [Crossref] [PubMed]
- Titmuss A, Longmore DK, Barzi F, et al. Association between hyperglycaemia in pregnancy and growth of offspring in early childhood: The PANDORA study. Pediatr Obes 2022;17:e12932. [Crossref] [PubMed]
- Chandna AR, Kuhlmann N, Bryce CA, et al. Chronic maternal hyperglycemia induced during mid-pregnancy in rats increases RAGE expression, augments hippocampal excitability, and alters behavior of the offspring. Neuroscience 2015;303:241-60. [Crossref] [PubMed]
- Huerta-Cervantes M, Peña-Montes DJ, López-Vázquez MÁ, et al. Effects of Gestational Diabetes in Cognitive Behavior, Oxidative Stress and Metabolism on the Second-Generation Off-Spring of Rats. Nutrients 2021;13:1575. [Crossref] [PubMed]
- Kim YH, Sung YH, Lee HH, et al. Postnatal treadmill exercise alleviates short-term memory impairment by enhancing cell proliferation and suppressing apoptosis in the hippocampus of rat pups born to diabetic rats. J Exerc Rehabil 2014;10:209-17. [Crossref] [PubMed]
- Mensah A, Toivanen S, Diewald M. Working Hours, Sleep Disturbance and Self-Assessed Health in Men and Women: A Multilevel Analysis of 30 Countries in Europe. Front Public Health 2022;10:818359. [Crossref] [PubMed]
- Prokopidis K, Dionyssiotis Y. Effects of sleep deprivation on sarcopenia and obesity: A narrative review of randomized controlled and crossover trials. J Frailty Sarcopenia Falls 2021;6:50-6. [Crossref] [PubMed]
- Song P, Zhao Y, Chen X, et al. Association between Sleep Duration and Mild Cognitive Impairment at Different Levels of Metabolic Disease in Community-Dwelling Older Chinese Adults. J Nutr Health Aging 2022;26:139-46. [Crossref] [PubMed]
- Zhu B, Shi C, Park CG, et al. Sleep quality and gestational diabetes in pregnant women: a systematic review and meta-analysis. Sleep Med 2020;67:47-55. [Crossref] [PubMed]
- Myoga M, Tsuji M, Tanaka R, et al. Impact of sleep duration during pregnancy on the risk of gestational diabetes in the Japan environmental and Children's study (JECS). BMC Pregnancy Childbirth 2019;19:483. [Crossref] [PubMed]
- Harskamp-van Ginkel MW, Ierodiakonou D, Margetaki K, et al. Gestational sleep deprivation is associated with higher offspring body mass index and blood pressure. Sleep 2020;43:zsaa110. [Crossref] [PubMed]
- Chambers JM, Wingert RA. PGC-1α in Disease: Recent Renal Insights into a Versatile Metabolic Regulator. Cells 2020;9:2234. [Crossref] [PubMed]
- Chen H, Fan W, He H, et al. PGC-1: a key regulator in bone homeostasis. J Bone Miner Metab 2022;40:1-8. [Crossref] [PubMed]
- Fernandez-Marcos PJ, Auwerx J. Regulation of PGC-1α, a nodal regulator of mitochondrial biogenesis. Am J Clin Nutr 2011;93:884S-90. [Crossref] [PubMed]
- Sun JP, Shi L, Wang F, et al. Modified Linggui Zhugan Decoction () Ameliorates Glycolipid Metabolism and Inflammation via PI3K-Akt/mTOR-S6K1/AMPK-PGC-1 α Signaling Pathways in Obese Type 2 Diabetic Rats. Chin J Integr Med 2022;28:52-9. [Crossref] [PubMed]
- Zhang Q, Song W, Zhao B, et al. Quercetin Attenuates Diabetic Peripheral Neuropathy by Correcting Mitochondrial Abnormality via Activation of AMPK/PGC-1α Pathway in vivo and in vitro. Front Neurosci 2021;15:636172. [Crossref] [PubMed]
- Wang L, Fan H, Zhou L, et al. Altered expression of PGC-1α and PDX1 and their methylation status are associated with fetal glucose metabolism in gestational diabetes mellitus. Biochem Biophys Res Commun 2018;501:300-6. [Crossref] [PubMed]
- Saner NJ, Bishop DJ, Bartlett JD. Is exercise a viable therapeutic intervention to mitigate mitochondrial dysfunction and insulin resistance induced by sleep loss? Sleep Med Rev 2018;37:60-8. [Crossref] [PubMed]
- Pedro PF, Tsakmaki A, Bewick GA. The Glucose Tolerance Test in Mice. Methods Mol Biol 2020;2128:207-16. [Crossref] [PubMed]
- Du M, Liu J, Han N, et al. Association between sleep duration in early pregnancy and risk of gestational diabetes mellitus: a prospective cohort study. Diabetes Metab 2021;47:101217. [Crossref] [PubMed]
- Zhang X, Zhang R, Cheng L, et al. The effect of sleep impairment on gestational diabetes mellitus: a systematic review and meta-analysis of cohort studies. Sleep Med 2020;74:267-77. [Crossref] [PubMed]
- Facco FL, Grobman WA, Reid KJ, et al. Objectively measured short sleep duration and later sleep midpoint in pregnancy are associated with a higher risk of gestational diabetes. Am J Obstet Gynecol 2017;217:447.e1-447.e13. [Crossref] [PubMed]
- Antza C, Kostopoulos G, Mostafa S, et al. The links between sleep duration, obesity and type 2 diabetes mellitus. J Endocrinol 2021;252:125-41. [Crossref] [PubMed]
- Yadav D, Cho KH. Total Sleep Duration and Risk of Type 2 Diabetes: Evidence-Based On Clinical and Epidemiological Studies. Curr Drug Metab 2018;19:979-85. [Crossref] [PubMed]
- Dutil C, Chaput JP. Inadequate sleep as a contributor to type 2 diabetes in children and adolescents. Nutr Diabetes 2017;7:e266. [Crossref] [PubMed]
- Dos Santos JM, Moreli ML, Tewari S, et al. The effect of exercise on skeletal muscle glucose uptake in type 2 diabetes: An epigenetic perspective. Metabolism 2015;64:1619-28. [Crossref] [PubMed]
- Liu Y, Bai H, Guo F, et al. PGC-1α activator ZLN005 promotes maturation of cardiomyocytes derived from human embryonic stem cells. Aging (Albany NY) 2020;12:7411-30. [Crossref] [PubMed]
(English Language Editor: J. Jones)