Oxygen pulse variation in symptomatic patients with suspected coronary artery disease: a diagnostic analysis
Highlight box
Key findings
• This study found that the difference between the value of the oxygen consumption/heart rate at anaerobic threshold and peak exercise [ΔVO2/HR(Peak–AT)] achieved an area under the curve of 0.804 in the diagnosis of coronary artery disease, with the sensitivity of 95.7% and the specificity of 62.5%.
What is known and what is new?
• Cardiopulmonary exercise testing has gradually been proven to be related to cardiac dysfunction, which provides an assessment approach to myocardial ischemia.
• The impact of cardiopulmonary exercise testing indicators in coronary artery disease and different severities of coronary artery stenosis is unclear.
What is the implication, and what should change now?
• ΔVO2/HR(Peak–AT) may be considered for clinical application as a diagnostic factor of coronary artery disease to help clinicians implement early intervention and treatments.
Introduction
Coronary artery disease (CAD) is one of the leading causes of death in both developed and developing countries (1). The early diagnosis of CAD is essential for the proper management of patients. Coronary angiography is a commonly used diagnostic method in clinical practice. However, it has some disadvantages, such as invasiveness, high examination cost, high requirements for physicians, advanced hospital equipment, and inapplicability to primary hospitals. Cardiopulmonary exercise testing (CPET), which combines an exercise stress test with ventilatory gas exchange measurements, has gradually been proven to be related to cardiac dysfunction, and provides a unique approach to assessing exercise-induced myocardial ischemia. Research has shown that the analysis of CPET indicators has better sensitivity, specificity, and negative and positive predictive values for cardiac ischemia than the analysis of electrocardiography changes only (2,3).
Oxygen pulse, a CPET indicator, is the ratio of oxygen consumption (VO2) to heart rate (HR) (VO2/HR) and reflects increments in stroke volume and oxygen extraction. Currently, oxygen pulse is considered one of the most relevant variables of myocardial ischemia. In theory, the development of myocardial ischemia during exercise may decrease heart stroke volume, and may result in changes in oxygen pulse. Over the past 2 decades, most studies have evaluated the diagnostic performance of oxygen pulse using myocardial ischemia detected by myocardial perfusion scan as the diagnostic standard (2,4,5). However, to date, there has been little discussion of the value of oxygen pulse compared to coronary artery anatomy data, and different studies have reported different results. Chaudhry et al. (6) observed an incremental linear decline in peak oxygen pulse in men with the progression of atherosclerotic-burden, but less progression in women. Conversely, Mazaheri et al. (7) compared the CPET indicators in patients with a mean diameter reduction >70% in at least 1 coronary artery to patients without CAD and found no significant differences in the ∆VO2/∆WR, peak VO2/HR, and the flattening duration of the oxygen pulse curve. This is likely due to the unclear classification of the anatomical severity of coronary stenosis defined by coronary arteriography. To the best of our knowledge, the impact of CPET indicators in CAD and different severities of coronary artery stenosis is unclear.
In the present study, we investigated the diagnostic value of CPET indicators in CAD, and compare the oxygen pulse response to incremental exercise in patients with different severities of coronary artery stenosis. We present the following article in accordance with the STARD reporting checklist (available at https://atm.amegroups.com/article/view/10.21037/atm-22-5279/rc).
Methods
Patients selection
In this cross-sectional study, we assessed 138 patients with symptoms suggestive of CAD, who had been referred to Shanghai East Hospital (Tongji University School of Medicine) from July 2020 to July 2021. All the patients underwent both a coronary angiography and CPET in the diagnostic process. The 24 patients diagnosed with CAD also underwent an intravenous ultrasound (IVUS) test. The inclusion criteria were any symptoms suggestive of CAD, including chest pain, dyspnea, palpitations, and syncope with or without abnormal electrocardiography findings. The exclusion criteria were all contraindications of CPET and a history of CAD, heart failure, the presence of other cardiac diseases (e.g., valvular heart disease or cardiomyopathies), pulmonary disease, arrhythmias, and anemia. The study was conducted in accordance with the Declaration of Helsinki (as revised in 2013). The study was approved by the institutional ethics board of Shanghai East Hospital (No. 2022_286). The requirement for informed consent was waived by the committee due to the retrospective nature of the study.
CPET
After a familiarization test, a symptom-limited, physician-supervised CPET was performed on an electromagnetically-braked cycle ergometer with a breath-by-breath analysis of ventilation and exhaled gas. All the tests were performed in accordance with the guidelines for cardiopulmonary exercise testing (CPX) of the American Heart Association and the American College of Sports Medicine (8,9). Patients were instructed not to eat for at least 2 h before the test, and not to drink tea, coffee, or cola drinks during that time. Before each test, the equipment was calibrated in a standard fashion according to the manufacturer’s instructions. All the patients had a 3-min baseline period and a 3-min warm-up period (warm-up, 0 W, 60 rpm), followed by the incremental exercise test, and a 5-min cool-down after exercise. The incremental exercise test used a customized linear-ramp protocol designed to elicit fatigue within 8–12 min of exercise. Blood pressure was measured and recorded automatically every 2 min. A 12-channel electrocardiogram (ECG) was used, and oxygen saturation was monitored throughout the duration of the test. The standard criteria for test termination included dyspnea, moderately severe angina, abnormal ST depression (>2 mm) in at least 2 adjacent leads, severe arrythmia, a sustained drop in systolic blood pressure, and marked hypertension (>250/130 mmHg) (9). In the absence of each of the above-mentioned criteria, the patients were encouraged to exert their maximal effort [respiratory exchange ratio (RER) target >1.0].
The VO2, carbon dioxide production (VCO2), and minute ventilation (VE) were continuously measured on a breath-by-breath basis. The anaerobic threshold (AT) was determined using the V-slope method (10). Peak oxygen uptake (peakVO2) was the average oxygen uptake during the last 15 s of the exercise. Predicted peak VO2 was the percentage of VO2 peak predicted by the anthropometric data. ΔVO2/ΔWR was automatically calculated by linear regression using the following formulae:
where T is the time of incremental exercise, and S is the slope of work rate increment in watts per minute (11). VE/VCO2 was the slope of ventilation versus CO2 output. The relationship between oxygen uptake and ventilation was expressed by the oxygen uptake efficiency slope (OUES) and was calculated using the following equation (8):
The oxygen pulse (VO2/HR) was calculated by dividing the VO2 by the heart rate throughout exercise, and peak VO2/HR was the percentage of the predicted VO2/HR. We also introduced new CPET indicators, including the ΔVO2/HR(Peak–AT), which was defined as the difference between the value of the VO2/HR at the AT and peak exercise.
Coronary angiography, IVUS, and taxus and cardiac surgery (SYNTAX) score
Coronary angiography and IVUS was performed through the radial artery using standard techniques within 2 days of CPET. Angiograms were obtained from multiple projections of coronary arteries and analyzed by 2 experienced cardiologists. Each segment was numerically evaluated according to the percentage reduction in the lumen diameter based on the nearest proximal intact coronary artery segment. CAD was diagnosed if stenosis was >30% in the left main artery and/or >50% in any other coronary arteries. The lesion site selected for the IVUS analysis was the image slice with the minimal lumen area (MLA) and area stenosis (AS), which were measured following the guidelines for IVUS measurements of the American College of Cardiology (12). Angiograms were also scored according to the SYNTAX score algorithm (13) by the 2 experienced cardiologists.
Statistical analysis
The statistical analysis was performed using the IBM SPSS Statistics 26.0 software package. The results for the continuous variables are expressed as the mean ± standard deviation (SD), and the categorical data are expressed as percentages. An analysis of the normality of the continuous variables was performed using the Kolmogorov-Smirnov test. The student’s t-test was used to compare continuous variables between groups. The categorical data were analyzed using the chi-square test or Fisher’s exact test. The receiver operating characteristic (ROC) curve was used to assess the optimal ΔVO2/HR(Peak–AT) threshold value, which maximized the sensitivity and specificity averages for predicting moderate and severe stenosis of the coronary artery. The area under the curve (AUC) >0.8 was considered to have a good diagnostic performance. Two-sided P value <0.05 was considered statistically significant.
Results
Patients
In total, 138 patients were included in this study. No myocardial infarctions or deaths resulted from the tests. The patients were grouped according to the results of the coronary angiography. Group 1 comprised 60 patients with <30% stenosis in the left main artery and <50% stenosis in any other coronary arteries. Group 2 comprised 78 patients with significant coronary lesions. Table 1 sets out the patients’ characteristics by group. More patients were taking antiplatelet medication and nitrates in Group 2 than Group 1, as the Group 2 patients had more significant stenosis. There were no significant differences between the 2 groups in terms of age, gender, body mass index (BMI), left ventricular ejection fraction (LVEF) at rest, and conventional coronary risk factors.
Table 1
Variables | Non-CAD (n=60) | CAD (n=78) | P |
---|---|---|---|
Age, years, mean ± SD | 60±8 | 61±9 | 0.375 |
Male, n (%) | 36 (60.0) | 49 (62.8) | 0.736 |
BMI, kg/m2, mean ± SD | 24.90±2.92 | 24.50±2.97 | 0.427 |
LVEF, %, mean ± SD | 65±4 | 65±4 | 0.503 |
Risk factors, n (%) | |||
Smoking | 20 (33.3) | 33 (42.3) | 0.383 |
Hypertension | 38 (63.3) | 50 (64.1) | 0.926 |
Hyperlipidemia | 4 (6.7) | 8 (10.3) | 0.458 |
Diabetes mellitus | 10 (16.7) | 14 (17.9) | 0.844 |
Medications, n (%) | |||
β-blockade | 29 (48.3) | 40 (51.3) | 0.731 |
Diuretics | 2 (3.3) | 4 (5.1) | 0.697 |
Antiplatelet medication | 34 (56.7) | 78 (100.0) | <0.001 |
Lipid-lowering treatment | 57 (95.0) | 78 (100.0) | 0.08 |
ACEI/ARB | 21 (35.0) | 33 (42.3) | 0.383 |
CCB | 22 (36.7) | 32 (41.0) | 0.603 |
Nitrates | 7 (11.7) | 22 (28.2) | 0.018 |
CAD, coronary artery disease; BMI, body mass index; LVEF, left ventricular ejection fraction; ACEI/ARB, angiotensin converting enzyme inhibitor/Angiotensin receptor blocker; CCB, calcium channel blocker.
The CPET indicators of patients with and without CAD are set out in Table 2. The peak RER, peak workload, and predicted peak workload were similar in the 2 groups. Additionally, the peak HR, heart rate reserve (HRR), 1st minute of HR recovery, and peak systolic blood pressure did not differ significantly between the patients with and without CAD. The patients with CAD had a lower VO2 at peak exercise; however, it did not differ significantly to that of the patients without CAD (18.65±3.92 vs. 19.26±3.57 mL/kg/min, P=0.344). Patients’ ventilation performance during CPET was also similar between the 2 groups, which was characterized by variables compatible with peak VE, predicted peak VE, OUES, and VE/VCO2. As for the relevant variables for myocardial ischemia, the 2 groups showed a continuous and similar increase in VO2/HR (10.14±2.38 vs. 10.78±3.08 mL/beat, P=0.171) and ∆VO2/∆WR (10.09±1.14 vs. 10.05±1.29 mL/watt/min, P=0.828) during exercise. The ΔVO2/HR(Peak–AT) mean value in the CAD group was 1.10±0.95 mL per beat, while that in the non-CAD group was 1.26±1.14 mL per beat (P=0.394).
Table 2
Variables | Non-CAD (n=60) | CAD (n=78) | P |
---|---|---|---|
RER | 1.12±0.07 | 1.12±0.08 | 0.872 |
Peak workload, W | 99±34 | 92±31 | 0.259 |
Predicted peak workload, % | 85.17±18.37 | 83.63±22.65 | 0.67 |
Peak HR, beats/min | 124±18 | 125±17 | 0.86 |
HRR, beats/min | 36±16 | 34±17 | 0.487 |
HR recovery at 1 min, beats/min | 17±7 | 17±8 | 0.837 |
Peak SBP, mmHg | 164±21 | 167±26 | 0.474 |
Peak VE, L/min | 47.31±14.98 | 45.43±13.87 | 0.446 |
Predicted peak VE, % | 59.70±12.50 | 60.42±16.52 | 0.779 |
OUES | 1730.58±370.64 | 1679.27±406.54 | 0.447 |
VE/VCO2 slope | 28.69±4.04 | 28.77±4.35 | 0.913 |
Peak VO2, mL/kg/min | 19.26±3.57 | 18.65±3.92 | 0.344 |
Peak VO2, % of predicted | 75.40±13.05 | 74.03±15.13 | 0.576 |
ΔVO2/ΔWR, mL/min/W | 10.05±1.29 | 10.09±1.14 | 0.828 |
Peak VO2/HR, mL/beat | 10.78±3.08 | 10.14±2.38 | 0.171 |
Peak VO2/HR, % of predicted | 98.35±18.69 | 95.38±17.27 | 0.186 |
VO2/HR at the AT, mL/beat | 9.52±2.29 | 9.04±2.19 | 0.206 |
ΔVO2/HR(Peak–AT), mL/beat | 1.26±1.14 | 1.10±0.95 | 0.394 |
Data are presented as mean ± SD. CAD, coronary artery disease; RER, respiratory exchange ratio; HR, heart rate; HRR, heart rate reserve; SBP, systolic blood pressure; OUES, oxygen uptake efficiency slope; SBP, systolic blood pressure; VE, ventilation; VCO2, carbon dioxide production; VO2, oxygen consumption; WR, work rate; AT, anaerobic threshold.
Table 3 shows the CPET indicators stratified by the SYNTAX scores based on the coronary angiography results of the patients with CAD. The high SYNTAX score group comprised 8 patients with a SYNTAX score >22, and no characteristic differences were found between the 2 groups. Similarly, no differences were observed in terms of the peak RER, peak workload, predicted peak workload, peak HR, HRR, 1st min of HR recovery results, peak systolic blood pressure, and ventilation variables. Additionally, the peak VO2/HR (10.89±2.88 vs. 10.06±2.33 mL/beat, P=0.352) and ∆VO2/∆WR (9.75±0.92 vs. 10.13±1.16 mL/watt/min, P=0.369) of the CAD patients with high SYNTAX scores did not differ significantly to those of the CAD patients with low SYNTAX scores. However, the ΔVO2/HR(Peak–AT) was significantly lower in the high SYNTAX score group than that in the low SYNTAX score group (0.20±0.84 vs. 1.21±0.91 mL/beat, P=0.004). A ROC curve was generated to determine the best cut-off value for the ΔVO2/HR(Peak–AT). The AUC was 0.804 [95% confidence interval (CI): 0.617–0.99, P=0.005] (see Figure 1). The value of –0.0074 mL per beat corresponded to the maximum joint sensitivity and specificity of the ROC curve (95.7% sensitivity and 62.5% specificity), which was defined as the cut-off value.
Table 3
Variables | Low SYNTAX score (<22) (n=70) | High SYNTAX score (≥22) (n=8) | P |
---|---|---|---|
RER | 1.12±0.08 | 1.10±0.05 | 0.481 |
Peak workload, W | 91±30 | 99±41 | 0.525 |
Predicted peak workload, % | 0.83±0.23 | 0.92±0.20 | 0.255 |
Peak HR (beats/min) | 125±17 | 123±17 | 0.82 |
HRR (beats/min) | 34±17 | 33±15 | 0.919 |
HR recovery at 1 min (beats/min) | 17±7 | 16±12 | 0.792 |
Peak SBP, mmHg | 166±25 | 177±30 | 0.236 |
Peak VE, L/min | 45.00±13.86 | 49.17±14.30 | 0.423 |
Predicted peak VE, % | 0.60±0.17 | 0.66±0.12 | 0.329 |
OUES | 1,677.99±380.39 | 1,690.49±625.74 | 0.957 |
VE/VCO2 slope | 28.57±4.35 | 30.48±4.30 | 0.242 |
Peak VO2, mL/kg/min | 18.51±3.81 | 19.86±4.90 | 0.36 |
Peak VO2, % of predicted | 0.73±0.15 | 0.82±0.15 | 0.136 |
ΔVO2/ΔWR, mL/min/W | 10.13±1.16 | 9.75±0.92 | 0.369 |
Peak VO2/HR, mL/beat | 10.06±2.33 | 10.89±2.88 | 0.352 |
Peak VO2/HR, % of predicted | 0.95±0.17 | 1.03±0.16 | 0.212 |
VO2/HR at the AT, mL/beat | 8.85±1.96 | 10.69±3.37 | 0.17 |
ΔVO2/HR(Peak–AT), mL/beat | 1.21±0.91 | 0.20±0.84 | 0.004 |
Data are presented as mean ± SD. CAD, coronary artery disease; SYNTAX, synergy between percutaneous coronary intervention with taxus and cardiac surgery; RER, respiratory exchange ratio; HR, heart rate; HRR, heart rate reserve; SBP, systolic blood pressure; OUES, oxygen uptake efficiency slope; VE, ventilation; VCO2, carbon dioxide production; VO2, oxygen consumption; WR, work rate; AT, anaerobic threshold.
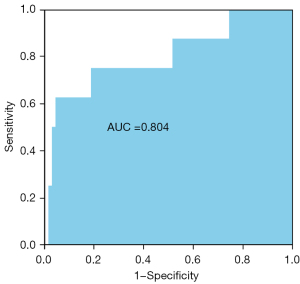
Among the 24 CAD patients undergoing IVUS, 13 had a percentage AS <65%, with a mean minimal lumen area-IVUS (MLA-IVUS) of 4.52±1.00 mm2, while the other 11 had a percentage AS >65% and had a significantly lower MAL-IVUS of 3.42±0.84 mm2 (P=0.009). Additionally, the group with a percentage AS >65% had a slower increase of O2 pulse and a significantly lower ΔVO2/HR(Peak–AT) (0.71±0.87 vs. 1.53±0.89 mL per beat, P=0.034). The other CPET indicators investigated, including the peak VO2/HR and ∆VO2/∆WR, were similar between the 2 groups (Table 4).
Table 4
Variables | IVUS-AS <65% (n=13) | IVUS-AS ≥65% (n=11) | P |
---|---|---|---|
IVUS-MLA, mm2 | 4.52±1.01 | 3.42±0.84 | 0.009 |
RER | 1.11±0.09 | 1.14±0.09 | 0.437 |
Peak workload, W | 102±22 | 105±35 | 0.824 |
Peak workload, % of predicted | 81.94±24.40 | 85.97±20.17 | 0.668 |
Peak HR, beats/min | 128±18 | 124±12 | 0.566 |
HRR, beats/min | 33±15 | 36±15 | 0.554 |
HR recovery at 1 min, beats/min | 19±8 | 18±8 | 0.891 |
Peak SBP, mmHg | 162±20 | 177±24 | 0.108 |
Peak VE, L/min | 47.62±10.24 | 49.48±12.38 | 0.691 |
Predicted peak VE, % | 56.65±14.65 | 59.75±9.82 | 0.558 |
OUES | 1,823.22±325.75 | 1,826.52±486.31 | 0.984 |
VE/VCO2 slope | 29.09±3.90 | 28.87±2.54 | 0.875 |
Peak VO2, mL/kg/min | 18.34±3.15 | 19.35±3.69 | 0.481 |
Peak VO2, % of predicted | 71.46±17.10 | 75.64±14.38 | 0.529 |
ΔVO2/ΔWR, mL/min/W | 10.02±0.92 | 10.06±1.09 | 0.92 |
Peak VO2/HR, mL/beat | 10.68±2.18 | 11.19±2.53 | 0.602 |
Peak VO2/HR, % of predicted | 88.77±18.51 | 96.35±13.29 | 0.269 |
VO2/HR at the AT, mL/beat | 9.15±1.60 | 10.48±2.85 | 0.166 |
ΔVO2/HR(Peak–AT), mL/beat | 1.53±0.89 | 0.71±0.87 | 0.034 |
Data are presented as mean ± SD. CAD, coronary artery disease; IVUS, intravascular ultrasound; AS, area stenosis; MLA, minimal lumen area; RER, respiratory exchange ratio; HR, heart rate; HRR, heart rate reserve; SBP, systolic blood pressure; OUES, oxygen uptake efficiency slope; SBP, systolic blood pressure; VE, ventilation; VCO2, carbon dioxide production; VO2, oxygen consumption; WR, work rate; AT, anaerobic threshold.
Discussion
CPET, which is an important non-invasive tool, provides objective and reproducible variables that may be used for the diagnostic and prognostic evaluation of cardiovascular disease. However, in recent years, relatively few studies have researched CPET. Thus, CPET is not usually employed to its full clinical potential. Our study evaluated the diagnostic application of CPET in CAD assessment, using the oxygen pulse related variable as an indicator of exercise-induced ischemia, and compared it to objective evidence of CAD based on coronary angiography.
Oxygen pulse is a measure of oxygen consumed per heartbeat obtained at CPET, which provides an estimate of LV stroke volume changes during exercise, assuming that the C(a–v)O2 is maximal, and no anemia is present (14). During the exercise-induced myocardial ischemic cascade, metabolic abnormalities, diastolic dysfunction, systolic dysfunction, and ECG changes occur in a sequential manner (15). This indicates that the change of oxygen pulse occurs early in the process of the myocardial ischemia and is manifested by a decrease in the value of oxygen pulse and the oxygen pulse trajectory prematurely flattening or declining (16). However, in this study we found no significant difference in peak VO2/HR between patients with or without CAD. Our results supported Mazaheri’s findings (7). Mazaheri also used the coronary angiography as the gold standard for diagnosing CAD and reported that while patients with CAD had a lower VO2/HR at peak exercise, it did not differ significantly to that of patients without CAD (P=0.61). Conversely, Chaudhr (6) demonstrated an incremental linear decline in the peak VO2/HR value with progression of atherosclerotic-burden.
Several studies have evaluated the diagnostic performance of the oxygen pulse using myocardial ischemia detected by myocardial perfusion scan as the diagnostic standard. Belardinelli (2) showed that ischemia patients diagnosed by Gated- single photon emission computed tomography (SPECT) had a significantly lower peak VO2/HR, while Pinkstaff (4) and Chen (5) found that the peak VO2/HR could not be used to identify patients with myocardial ischemia. This discrepancy could be attributed to the different severities of ischemia in these studies, as oxygen pulse response during incremental exercise might only present with extensive myocardial ischemia (17,18). In the current study, we found no significant differences in the peak VO2/HR between the low and high SYNTAX score groups, as similar as between the IVUS-AS <65% and ≥65% groups. This may be because the patients who were referred to our center for CPET with symptoms of suspected ischemic CAD were usually in a good physical condition.
In Belardinelli’s study, 86% of CAD patients had at least 2 coronary arteries with >50% stenosis and 91% had positive myocardial scintigraphy (2). Conversely, in our study only 22 (28%) CAD patients had more than 1 coronary artery with >50% stenosis. The severity of coronary artery stenosis in our patients may be insufficient to induce extensive myocardial ischemia to achieve a positive result of an oxygen pulse response during exercise, indicating that a decrease in the peak oxygen pulse has low sensitivity and specificity for the diagnosis of patients with mild to moderate coronary artery stenosis, and has no positive predictive effect in assessing the severity of coronary artery stenosis.
In 2003, duration of oxygen pulse flattening was reported to be an independent predictor of myocardial ischemia detected by a positive myocardial scintigraphy (2). Since then, the focus has begun to shift to the trajectory of the oxygen pulse curve. However, the results remain controversial. Chaudhry (6) demonstrated that the oxygen pulse trajectory provides valuable information that can be used to evaluate patients with CAD. Conversely, De Lorenzo (19,20) classified the oxygen pulse curves into 4 patterns and found that the oxygen pulse curve pattern had low diagnostic performance in the diagnosis of CAD, and the abnormal curve pattern was not associated with myocardial ischemia defined by scintigraphy. Unfortunately, the process of identifying the oxygen pulse curve pattern may be highly subjective. Thus, we introduced the variable of ΔVO2/HR(Peak–AT), which was defined as the difference between the value of VO2/HR at the AT and peak exercise, to try to quantify the variation of the oxygen pulse curve pattern. The smaller the value, the less the oxygen pulse curve increase or the more the oxygen pulse curve decreases after the AT. We found no significant difference in the ΔVO2/HR(Peak–AT) between the CAD group and the non-CAD group. However, the patients with high SYNTAX scores showed a significant reduction in the ΔVO2/HR(Peak–AT) compared to the patients with low SYNTAX scores.
There was also a significant difference in the ΔVO2/HR(Peak–AT) between groups divided according to the IVUS-AS. The patients with a high SYNTAX score or IVUS-AS >65% generally had the more severe stenosis of the coronary artery, which means that they are likely to show more extensive myocardial ischemia during exercise. Thus, our results further support the idea that the variation in the oxygen pulse response to incremental exercise can detect extensive but not mild myocardial ischemia, and the value of ΔVO2/HR(Peak–AT), as a quantified variable of oxygen pulse curve development, has higher sensitivity in detecting myocardial ischemia than other CPET indicators.
It is somewhat surprising that no differences were found in the remaining variables of CPET, including peak VO2 and ∆VO2/∆WR, which are also considered to be related to myocardial ischemia (2,21,22). This finding might also be attributed to the differences in the patient populations between studies. In our study, 72% of the CAD patients had >50% stenosis in only 1 coronary artery, and 10.3% of the CAD patients with a high SYNTAX score (≥22) had a moderate to severe obstructive coronary artery. Thus, our results are likely to be related to the mild myocardial ischemia of these patients. This suggests that, as with the VO2/HR, the variation in the ∆VO2/∆WR response to exercise and peak VO2 are not sensitive to mild and moderate myocardial ischemia.
One limitation of the present study was the lack of myocardial perfusion scintigraphy data and LV function during exercise, which could help to identify of the extent of the myocardial ischemia. Additionally, the patients in the non-CAD group also had symptoms, and we could not exclude the possibility of micro-vascular ischemia, as the coronary arteriography and IVUS could only assess the proximal vessels of the coronary artery. It has previously been observed that micro-vascular ischemia is becoming increasingly recognized in symptomatic patients, especially in women with angiographically normal coronary arteries (23). Thus, having age- and sex-matched asymptomatic, relatively sedentary individuals without cardiovascular risk factors would have made for a more ideal healthy cohort. However, it was not feasible in this clinical observational study. Another limitation of this study was the small sample size, especially that of patients with severe stenosis of coronary artery. This difference in the patient populations between the groups may have had an effect on our findings. Thus, continued investigations with a larger sample size are needed.
Conclusions
This study showed that the decrease in the peak oxygen pulse response to incremental exercise was not generally present in patients with mild to moderate myocardial ischemia. The variable of ΔVO2/HR(Peak–AT), which has been identified as a quantitative indicator for the variation of oxygen pulse response after the AT during incremental exercise, has been shown to have good predictive ability for identifying the stenosis of the coronary artery. Future research in this area should seek to quantifiably determine the value of CPET in diagnosing CAD and assessing the function of the coronary artery compared to other variables [e.g., fractional flow reserve (FFR) and quantitative flow ratio (QFR)] to guide interventional treatments.
Acknowledgments
Funding: This study was supported by the Top-Level Clinical Discipline Project of Shanghai Pudong (No. PWYgf2021-01) and the Jiangxi Province Municipal Health Commission Science Technology Grant (No. 202212848).
Footnote
Reporting Checklist: The authors have completed the STARD reporting checklist. Available at https://atm.amegroups.com/article/view/10.21037/atm-22-5279/rc
Data Sharing Statement: Available at https://atm.amegroups.com/article/view/10.21037/atm-22-5279/dss
Conflicts of Interest: All authors have completed the ICMJE uniform disclosure form (available at https://atm.amegroups.com/article/view/10.21037/atm-22-5279/coif). The authors have no conflicts of interest to declare.
Ethical Statement: The authors are accountable for all aspects of the work in ensuring that questions related to the accuracy or integrity of any part of the work are appropriately investigated and resolved. The study was conducted in accordance with the Declaration of Helsinki (as revised in 2013). The study was approved by the institutional ethics board of Shanghai East Hospital (No. 2022_286). The requirement for informed consent was waived by the committee due to the retrospective nature of the study.
Open Access Statement: This is an Open Access article distributed in accordance with the Creative Commons Attribution-NonCommercial-NoDerivs 4.0 International License (CC BY-NC-ND 4.0), which permits the non-commercial replication and distribution of the article with the strict proviso that no changes or edits are made and the original work is properly cited (including links to both the formal publication through the relevant DOI and the license). See: https://creativecommons.org/licenses/by-nc-nd/4.0/.
References
- GBD 2015 Mortality and Causes of Death Collaborators. Global, regional, and national life expectancy, all-cause mortality, and cause-specific mortality for 249 causes of death, 1980-2015: a systematic analysis for the Global Burden of Disease Study 2015. Lancet 2016;388:1459-544. [Crossref] [PubMed]
- Belardinelli R, Lacalaprice F, Carle F, et al. Exercise-induced myocardial ischaemia detected by cardiopulmonary exercise testing. Eur Heart J 2003;24:1304-13. [Crossref] [PubMed]
- Li N, Liu J, Ren Y, et al. Diagnostic value of the cardiopulmonary exercise test in coronary artery disease. J Thorac Dis 2022;14:607-13. [Crossref] [PubMed]
- Pinkstaff S, Peberdy MA, Kontos MC, et al. Usefulness of decrease in oxygen uptake efficiency slope to identify myocardial perfusion defects in men undergoing myocardial ischemic evaluation. Am J Cardiol 2010;106:1534-9. [Crossref] [PubMed]
- Chen P, Wu T, Peng Q, et al. Application value between dynamic electrocardiogram and MSCT myocardial perfusion imaging in the diagnosis of myocardial ischemia in coronary heart disease. Ann Palliat Med 2021;10:10720-5. [Crossref] [PubMed]
- Chaudhry S, Kumar N, Behbahani H, et al. Abnormal heart-rate response during cardiopulmonary exercise testing identifies cardiac dysfunction in symptomatic patients with non-obstructive coronary artery disease. Int J Cardiol 2017;228:114-21. [Crossref] [PubMed]
- Mazaheri R, Shakerian F, Vasheghani-Farahani A, et al. The usefulness of cardiopulmonary exercise testing in assessment of patients with suspected coronary artery disease. Postgrad Med J 2016;92:328-32. [Crossref] [PubMed]
- Glaab T, Taube C. Practical guide to cardiopulmonary exercise testing in adults. Respir Res 2022;23:9. [Crossref] [PubMed]
- Riebe D, Franklin BA, Thompson PD, et al. Updating ACSM's Recommendations for Exercise Preparticipation Health Screening. Med Sci Sports Exerc 2015;47:2473-9. [Crossref] [PubMed]
- Rovai S, Contini M, Sciomer S, et al. The double anaerobic threshold in heart failure. Int J Cardiol 2022;353:68-70. [Crossref] [PubMed]
- Sacre JW, Wong C, Chan YK, et al. Left Ventricular Dysfunction and Exercise Capacity Trajectory: Implications for Subclinical Heart Failure Staging Criteria. JACC Cardiovasc Imaging 2019;12:798-806. [Crossref] [PubMed]
- Barua RS, Rigotti NA, Benowitz NL, et al. 2018 ACC Expert Consensus Decision Pathway on Tobacco Cessation Treatment: A Report of the American College of Cardiology Task Force on Clinical Expert Consensus Documents. J Am Coll Cardiol 2018;72:3332-65. [Crossref] [PubMed]
- Vizirianakis IS, Chatzopoulou F, Papazoglou AS, et al. The GEnetic Syntax Score: a genetic risk assessment implementation tool grading the complexity of coronary artery disease-rationale and design of the GESS study. BMC Cardiovasc Disord 2021;21:284. [Crossref] [PubMed]
- Accalai E, Vignati C, Salvioni E, et al. Non-invasive estimation of stroke volume during exercise from oxygen in heart failure patients. Eur J Prev Cardiol 2020; Epub ahead of print. [Crossref] [PubMed]
- Lie ØH, Chivulescu M, Rootwelt-Norberg C, et al. Left Ventricular Dysfunction in Arrhythmogenic Cardiomyopathy: Association With Exercise Exposure, Genetic Basis, and Prognosis. J Am Heart Assoc 2021;10:e018680. [Crossref] [PubMed]
- Guazzi M, Arena R, Halle M, et al. 2016 focused update: clinical recommendations for cardiopulmonary exercise testing data assessment in specific patient populations. Eur Heart J 2018;39:1144-61. [Crossref] [PubMed]
- Petek BJ, Churchill TW, Sawalla Guseh J, et al. Utility of the oxygen pulse in the diagnosis of obstructive coronary artery disease in physically fit patients. Physiol Rep 2021;9:e15105. [Crossref] [PubMed]
- van de Wouw J, Sorop O, van Drie RWA, et al. Perturbations in myocardial perfusion and oxygen balance in swine with multiple risk factors: a novel model of ischemia and no obstructive coronary artery disease. Basic Res Cardiol 2020;115:21. [Crossref] [PubMed]
- De Lorenzo A, Da Silva CL, Castro Souza FC, et al. Value of the oxygen pulse curve for the diagnosis of coronary artery disease. Physiol Res 2018;67:679-86. [Crossref] [PubMed]
- De Lorenzo A, da Silva CL, Souza FCC, et al. Clinical, scintigraphic, and angiographic predictors of oxygen pulse abnormality in patients undergoing cardiopulmonary exercise testing. Clin Cardiol 2017;40:914-8. [Crossref] [PubMed]
- Kurpaska M, Krzesiński P, Gielerak G, et al. Cardiopulmonary exercise testing and impedance cardiography in the assessment of exercise capacity of patients with coronary artery disease early after myocardial revascularization. BMC Sports Sci Med Rehabil 2022;14:134. [Crossref] [PubMed]
- Liu W, Liu X, Liu T, et al. The Value of Cardiopulmonary Exercise Testing in Predicting the Severity of Coronary Artery Disease. J Clin Med 2022;11:4170. [Crossref] [PubMed]
- Ouellette ML, Löffler AI, Beller GA, et al. Clinical Characteristics, Sex Differences, and Outcomes in Patients With Normal or Near-Normal Coronary Arteries, Non-Obstructive or Obstructive Coronary Artery Disease. J Am Heart Assoc 2018;7:e007965. [Crossref] [PubMed]
(English Language Editor: L. Huleatt)