Using arterial phase hyperenhancement on CT instead of gadoxetic acid arterial phase enhancement may improve the diagnostic performance for hepatocellular carcinoma
Highlight box
Key findings
• In conclusion, APHE in CTAP images showed a significantly higher detection rate than in MRAP images. The combined use of dynamic-enhanced CT vascular features for HCC diagnosis produced high sensitivity and comparable specificity when compared with the gadoxetic acid-enhanced MRI only.
What is known and what is new?
• The detection performance using gadoxetic acid-enhanced MR imaging for HCC is hampered due to the high incidence of transient severe motion in AP.
• Using CTAP instead of gadoxetic acid-enhanced MRAP can improve the diagnostic sensitivity for HCC and also yields a comparable specificity.
What is the implication, and what should change now?
• Dynamic-contrast-enhanced CT vascular features can be used as an important supplement in the diagnosis of HCC when transient severe dyspnea-related motion happened using gadoxetic acid-enhanced MRI.
Introduction
Despite the great advantages of gadoxetic acid-enhanced magnetic resonance imaging (MRI) in detecting and diagnosing hepatocellular carcinoma (HCC), particularly small HCCs, transient severe dyspnea-related motion (TSDM) artifacts in the arterial phase (AP) still remain a serious problem, as they may reduce the detection of HCC (1,2). Arterial phase hyperenhancement (APHE) is recommended as the major imaging feature in most major guidelines because of its high diagnostic sensitivity (3-5). In addition, when combined with the feature of portal venous or delayed phase hypointensity, it can reach the optimal diagnostic efficiency (5-7). Given the importance of the arterial phase in diagnosing HCC, the challenge of bridging the gap between transient severe motion artifacts and arterial phase image quality is an urgent one.
At present, several strategies are used to overcome this limitation, and these strategies are divided into 2 categories. First is the reduction of the incidence rate of transient severe dyspnea by lowering the injection rate, diluting the contrast agent, or performing respiratory exercises before magnetic resonance (MR) examination (8,9). Second is the honing of the MR technique by shortening the scanning time, using a free breath-hold MR sequence, or achieving multiple arterial phase images (10-12). However, once transient severe dyspnea occurs, arterial phase images cannot be guaranteed for any of these strategies. Dynamic contrast-enhance computed tomography (CT) imaging can provide excellent AP images due to fewer adverse events and greater differences in lesion enhancement density. Thus, using the computed tomography arterial phase (CTAP) images instead of the magnetic resonance arterial phase (MRAP) with gadoxetic acid-enhanced imaging may improve the detection rate and diagnostic performance for hepatic nodules. Previous studies conducted by Basha et al. (13) and Kim et al. (14) investigated the combined use of MR and computed tomography (CT) imaging features for improving the diagnostic performance of the Liver Imaging Reporting and Data System (LI-RADS). Furthermore, Park et al. (15) compared the diagnostic efficiency of an abbreviated MR protocol combined with CT features with that of full gadoxetic acid-enhanced MR sequences, and comparable diagnostic performance was found with the combined imaging protocol set. To our knowledge, very few studies have investigated whether the use of CTAP images can be used as an alternative for the gadoxetic acid-enhanced AP images for the diagnostic performance of hepatic nodules based on various imaging diagnostic criteria.
Therefore, this study aimed to determine whether the combined use of CTAP and gadoxetic acid-enhanced MR imaging can improve the diagnostic performance for HCC based on various imaging diagnostic criteria. We present the following article in accordance with the STARD reporting checklist (available at https://atm.amegroups.com/article/view/10.21037/atm-22-4968/rc).
Methods
Study population
The study was conducted in accordance with the Declaration of Helsinki (as revised in 2013). This study was approved by the Institutional Review Board of West China Hospital [No. 2016(297)]. Due to our study’s retrospective nature, the requirement for written informed consent was waived. In addition, the sample size was estimated by using the clinical experience. This retrospective diagnostic study included 316 patients with a persistent history of chronic hepatic disease (CHD) or cirrhosis who underwent gadoxetic acid-enhanced MRI between January 2014 and December 2020. Of these patients, 174 were excluded for the following reasons: (I) history of liver resection (n=11), transarterial chemotherapy (n=22), and radiofrequency ablation (n=5); (II) patients lacked dynamic-enhanced CT examination (n=52); (III) hepatic nodules were typically hemangiomas (n=17) or cysts (n=24); and (IV) the final diagnosis was not confirmed by histopathological results or at the 12-month clinical follow-up (n=43). Finally, 142 patients with 169 hepatic nodules were enrolled.
Imaging technique
MRI was performed using 3.0 T MR systems (Discovery 750w, GE Healthcare, Milwaukee, USA; Skyra 3.0 T, Siemens Healthcare, Erlangen, Germany). All measurements were made using the 16-channel phase array torsor coils. A standard injection protocol of the contrast agent (Primovist, Bayer Pharma AG, Berlin, Germany) was used for all the patients by administering a bolus injection of 0.025 mmol/kg at an injection rate of 1 mL/s, followed by a 20-mL saline flush. The routine MR protocol for gadoxetic acid-enhanced MR imaging included the following: (I) in- and out-of-phase T1-weighted imaging; (II) T2-weighted imaging with fat saturation; (III) diffusion-weighted imaging; (IV) axial non-enhanced T1-weighted imaging with fat saturation; and (V) enhanced T1-weighted imaging at the arterial phase (25–30 s), portal venous phase (60 s), delayed phase (180 s), and hepatobiliary phase (20 min). In addition, CT imaging was performed using multidetector CT scanners (Revolution, GE Healthcare, Milwaukee, USA; SOMATOM Definition, Siemens Healthcare, Erlangen, Germany). The pre-contrast images were initially acquired before the contrast agent injection (iodine concentration, 300–370 mg/mL; volume, 1.5–2.0 mL/kg of body weight; contrast type, Iopromide injection, Bayer Pharma AG) and the images in the arterial phase and portal venous phase were acquired with the following parameters: tube voltage, 100–120 kVp; tube current, 450 mA; slice thickness, 0.625 mm; pitch, 0.992:1; rotation speed: 0.5 s/rot; and volume-based adaptive statistical iterative reconstruction (ASIR-V): 30%.
Image analysis
On a per-lesion basis, a reference standard based on pathology was established and the pathological results were defined as the gold standard. All the MR and CT images were firstly anonymized. According to the different protocol sets, the MR sequences and CT images were combined into different categories. In this study, 2 different combined protocol sets were compiled, including: (I) Protocol-I: full gadoxetic acid-enhanced MR imaging sequences; and (II) Protocol-II: dynamic CT combined with the full gadoxetic acid-enhanced MR imaging but excluding the vascular features-related phase (arterial phase images). After reviewing these images, the reviewers characterized the nodules according to the LI-RADS v2018 and the European Association for the Study of the Liver (EASL) and 2018 Korean guidelines. Three independent reviewers were blinded to the histopathological findings and clinical data, and when the reviewers could not fully agree, a consensus was achieved by using the assessment results of the majority. The MR images were presented in a randomized fashion. In order to minimize recall bias, the reviews of the different combinations of protocols in the same patient were spaced 3–4 weeks. Additionally, all 3 reviewers were advised to read the MR images in a clinical manner, and were allowed to use the coronal and sagittal reconstructions.
Statistical analysis
Lesion detection and diagnosis status were summarized using frequencies and percentages for each protocol. To assess the clinical impact of arterial phase CT images on the detection of the APHE, paired comparisons of arterial phase images between CT and MRI were performed using the McNemar test. Furthermore, with regard to the diagnostic performance for HCC using the LI-RADS v2018 and the EASL and Korean guidelines, the sensitivity was calculated by using the formula:
and specificity was calculated by using the formula:
and the sensitivity and specificity were subsequently compared by using the McNemar test. Interobserver agreement for APHE on the CT and MR arterial phase images, washout during the portal venous phase, and the enhancing capsule were all evaluated using an e-weighted k statistic. A two-sided P value of less than 0.05 was considered to be statistically significant. All statistical analysis were performed using a statistical software package (SPSS 23.0, SPSS Inc., Chicago, IL, USA).
Results
Patient characteristics
There were a total of 142 patients (mean age, 50.89±11.25 years; range, 26–83 years) with 169 nodules, including 113 men (50.70±11.16 years; range, 26–83 years) and 29 women (51.62±11.76 years; range, 30–67 years). Of these, 47 patients had hepatic cirrhosis (33.10%, 47/142). In addition, 116 patients (81.69%, 116/142) had 1 nodule and 26 patients (18.31%, 26/142) had 2 or 3 nodules, respectively. The 169 nodules comprised 137 HCC and 32 non-HCC nodules [23 intrahepatic cholangiocarcinomas (IHCCs), 2 focal nodular hyperplasias (FNHs), 3 dysplastic nodules (DNs), and 4 angiomyolipomas (AMLs)]. Table 1 summarizes the clinical and MRI features of the 142 patients.
Table 1
Characteristics | Total (n=142) |
---|---|
Sex | |
Female | 29 (20.42) |
Male | 113 (79.58) |
Age, years | 50.89±11.25 |
AFP (ng/mL) | 502.87±1,864.91 |
CEA (ng/mL) | 3.93±13.01 |
CA19-9 (U/mL) | 29.77±51.84 |
HBSAG (COI) | 1,724.31±1,412.44 |
ALT (IU/L) | 41.76±34.91 |
AST (IU/L) | 40.79±25.17 |
TBIL (μmol/L) | 15.72±7.28 |
DBIL (μmol/L) | 5.86±3.90 |
IBIL (μmol/L) | 9.86±4.69 |
ALB (g/L) | 42.49±4.53 |
Pt (s) | 12.17±1.02 |
PLt (109/L) | 148.27±67.09 |
Number of nodules per patient | |
Patients with 1 nodule | 116 |
Patients with 2 nodules | 25 |
Patients with 3 nodules | 1 |
Data are shown as n (%) or mean ± standard deviation. AFP, alpha fetoprotein; CEA, carcinoembryonic antigen; CA19-9, carbohydrate antigen 19-9; HBSAG, hepatitis B surface antigen; ALT, alanine aminotransferase; AST, aspartate aminotransferase; TBIL, total bilirubin; DBIL, direct bilirubin; IBIL, indirect bilirubin; ALB, albumin; Pt, prothrombin time; PLt, platelet.
Comparison of the APHE
For the whole hepatic nodules, the detection rate of APHE on CT arterial phase (CTAP) images was significantly higher than that on the magnetic resonance arterial phase (MRAP) images [87.57% (148/169) vs. 75.15% (127/169); P<0.001; Table 2]. In the subgroup analysis, for the APHE of HCC, the CTAP images showed a significantly higher APHE detection rate than the MRAP images [97.08% (133/137) vs. 82.48% (113/137); P<0.001]. However, the detection rate for APHE was not significantly different between the CTAP and MRAP images for non-HCC lesions [46.88% (15/32) vs. 43.75% (14/32); P=1.000]. Additionally, the CTAP images showed a higher detection rate for APHE images less than or equal to 2.0 cm in diameter [92.31% (24/26) vs. 69.23% (18/26); P=0.031] and in HCCs greater than 2.0 cm in diameter [98.20% (109/111) vs. 85.59% (95/111); P<0.001] (Table 2). Interobserver agreement for the APHE of CTAP (Kappa value =0.87) was better than that of MRAP (Kappa value =0.74). Good interobserver agreement was also obtained for the portal venous/delayed phase washout appearance (Kappa value =0.83) and capsule appearance (Kappa value =0.81).
Table 2
Category | Computed tomographic arterial phase images | Magnetic resonance arterial phase images | P value |
---|---|---|---|
Total nodules (n=169) | 148 (87.57%) | 127 (75.15%) | <0.001 |
Diagnosis | |||
HCC (n=137) | 133 (97.08%) | 113 (82.48%) | <0.001 |
Non-HCC (n=32) | 15 (46.88%) | 14 (43.75%) | 1.000 |
HCC size | |||
≤20 mm (n=26) | 24 (92.31%) | 18 (69.23%) | 0.031 |
>20 mm (n=111) | 109 (98.20%) | 95 (85.59%) | <0.001 |
HCC, hepatocellular carcinoma.
Diagnostic performance of various criteria for HCC
For the LI-RADS criteria (Figure 1), when the LI-RADS-5 (LR-5) was regarded to be HCC, the CTAP combined images significantly increased the sensitivity to 75.91% (104/137) from the 70.80% (97/137) of the MR-only images (P=0.016), with a slight decrease in the diagnostic specificity from 75.00% (24/32) to 71.88% (23/32) (P=1.000). Additionally, when the LR-4/5 were regarded to be HCC, MR-only images showed a sensitivity of 85.40% (117/137) and a specificity of 65.63% (21/32) for diagnosing HCC. However, when we determined APHE on the CTAP, the sensitivity of CTAP combined image sets showed a statistically significant increase to 96.35% (132/137; P<0.001), whereas the specificity decreased to 62.50% (20/32), but without statistical significance (P=1.000).
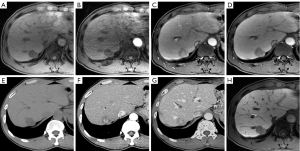
For the EASL criteria (Figure 2), MR-only images showed a sensitivity of 78.10% (107/137) and a specificity of 71.88% (23/32) for the diagnosis of HCC. However, when the APHE was determined on the CTAP, the sensitivity value increased to 81.02% (111/137) (P=0.152), whereas the specificity also increased to 75.00% (24/32), but without statistical significance (P=1.000).
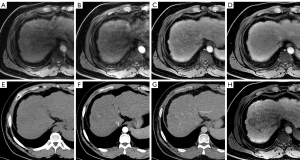
For the Korean guidelines (Figure 3), the CTAP-combined images significantly increased the sensitivity to 94.89% (130/137) from the 83.21% (114/137) of the MR-only images (P<0.001). The specificity also increased to 65.63% (21/32) from 62.50% (20/32), which was not statistically significant (P=1.000) (Table 3).
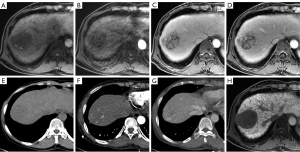
Table 3
Category | TP | FP | FN | TN | Sensitivity, % | P | Specificity, % | P | Accuracy, % |
---|---|---|---|---|---|---|---|---|---|
LR-5 | |||||||||
MRAP | 97 | 8 | 40 | 24 | 70.80 (63.09–78.15) | 0.016 | 75.00 (59.14–90.86) | 1.000 | 71.60 (64.73–78.47) |
CTAP | 104 | 9 | 33 | 23 | 75.91 (68.66–83.16) | 71.88 (55.41–88.34) | 75.15 (68.57–81.73) | ||
LR-4/5 | |||||||||
MRAP | 117 | 11 | 20 | 21 | 85.40 (79.41–91.39) | <0.001 | 65.63 (48.23–83.02) | 1.000 | 81.66 (75.76–87.55) |
CTAP | 132 | 12 | 5 | 20 | 96.35 (93.17–99.53) | 62.50 (44.77–80.23) | 89.94(85.36–94.52) | ||
EASL | |||||||||
MRAP | 107 | 9 | 30 | 23 | 78.10 (71.09–85.12) | 0.152 | 71.88 (55.41–88.34) | 1.000 | 76.92 (70.51–83.34) |
CTAP | 111 | 8 | 26 | 24 | 81.02 (74.37–87.67) | 75.00 (59.14–90.86) | 79.88 (73.78–85.99) | ||
Korean | |||||||||
MRAP | 114 | 12 | 23 | 20 | 83.21 (76.87–89.55) | <0.001 | 62.50 (44.77–80.23) | 1.000 | 79.29 (73.12–85.46) |
CTAP | 130 | 11 | 7 | 21 | 94.89 (91.16–98.62) | 65.63 (48.23–83.02) | 89.35 (84.65–94.05) |
HCC, hepatocellular carcinoma; LI-RADS (LR), Liver Imaging Reporting and Data System; EASL, European Association for the Study of the Liver; MRAP, magnetic resonance arterial phase images; CTAP, computed tomographic arterial phase images; TP, true positive; FN, false negative; FP, false positive; TN, true negative.
Discussion
In the present study, we combined CTAP and MRAP images to explore whether this new diagnostic method could improve arterial phase enhancements in patients with HCC and the diagnostic performance of the various guideline criteria. The results demonstrated that compared with the MRAP images, the CTAP images showed significantly higher detection rates for APHE in both the total nodules and HCCs. Furthermore, the combination of vascular features on CTAP could significantly improve sensitivity for the diagnosis of HCC on the various criteria (LI-RADS criteria, EASL, and Korean guidelines), particularly for LI-4/5 and the 2018 Korean guideline. The study also showed that the specificity of the diagnosis was almost unaffected using this approach. Therefore, our study may be able to improve the diagnostic performance of gadoxetic acid-enhanced MRI in patients with HCC and further affect the clinical treatment plan.
Hyperenhancement of the arterial phase is one of the most important imaging features for the diagnosis of HCCs (4). According to previous research, the APHE results from neoangiogenesis of the unpaired artery in the nodule during hepatocarcinogenesis (16), and APHE was shown in 68.4–82.7% of early-stage HCCs (17-23). Therefore, the detection of APHE is of great importance for the diagnosis of HCCs. In the comparison made for the APHE, we found that the CTAP images showed a better diagnostic performance for the identification of APHE in patients with HCCs than MRAP. For all the HCC lesions, the detection rate of APHE using CTAP was 14.6% higher than MRAP, 23.08% higher in HCCs less than or equal to 2.0 cm in diameter, and 12.61% higher in HCCs greater than 2.0 cm in diameter. Park et al. (15) reported that the nonrim APHE tended to be more common in abbreviated MRI with optional multiphasic CT than full-sequence gadoxetic acid-enhanced MRI, although there was no significant difference between both groups mentioned above. Our results were similar, and this can be attributed to the following 3 explanations: first, as reported in previous studies, there is a high frequency of transient serve motion (TSM) in gadoxetic acid-enhanced MRI. Therefore, about 30% of patients’ arterial phase images are disturbed by respiratory motion artifacts, which seriously interfere with the diagnosis of APHE by radiologists. However, there was no report of TSM with CT contrast agents. Second, according to this principle, the degree of MRI enhancement following contrast agent administration is closely related to the amount and relaxation rate of the contrast agent. The gadoxetic acid used in this study had a high relaxation rate but with a small injection dose of 0.025 mol/kg, which is equal to 0.2 mL/kg of body weight. In contrast, the injection dose used in CT dynamic enhancement examination can be as high as 1.5–2.0 mL/kg of body weight, which leads to a more obvious transient liver perfusion effect in CTAP than in MRAP. Third, and more importantly, gadoxetic acid is a dual channel excretory contrast agent, about 50% of which is ingested by hepatocytes and excreted through the biliary system, and the uptake by hepatocytes can occur as early as 20–35 s after injection (24,25). This means that the extracellular enhancement effect of gadoxetic acid will be affected, resulting in less enhancement of MRAP.
In the evaluation of diagnostic efficacy for HCCs, we found that regardless of the 2018 Korean criteria or the LI-RADS criteria, particularly for LI-RADS 4/5, the sensitivity for the diagnosis of HCCs was higher using CTAP images than MRAP images, and the difference was statistically significant. This may be because sensitivity primarily reflects the ability to detect lesions, whereas the APHE sign is a very important indicator for judging HCC lesions in the LI-RADS criteria and the 2018 Korean criteria. The CTAP images can reflect the APHE of the lesions better than MRAP; therefore, it further improves the sensitivity of the LI-RADS criteria and the 2018 Korean criteria for HCC diagnosis. In the EASL criteria, the use of CTAP images tended to improve the sensitivity for HCC diagnosis compared with the MRAP images (81.02% vs. 78.10%), but without a significant difference (P=0.152). We speculate that this may be because, in the EASL criteria, the diagnosis of HCC emphasizes both the APHE and portal vein phase (PVP) washout. However, for some atypical HCC lesions, the PVP washout may not be obvious. Although the CTAP images improved their APHE display compared to MRAP, they still could not be used to diagnose HCCs using the EASL criteria, which required both APHE and portal PVP washout. Therefore, improvement of the sensitivity in EASL criteria was not clear.
Both the diagnostic sensitivity and specificity are important for hepatic nodule surveillance and treatment decisions. Our research demonstrated that when using CTAP instead of the MRAP image as the vascular feature of the lesion, whether for LR-5, LR-4/5, the EASL criteria, or the Korean guideline, the specificity of diagnosis for HCCs was not affected, but the sensitivity improved. Particularly for the EASL criteria and the Korean guideline, the CTAP images showed higher specificity than the MRAP image. We speculate that the reason may be because some of the lesions we included, such as IHCC, were caused by motion artifacts on the MRAP image, which manifested as arterial mass enhancement with HCC signs and were mistaken for HCC. However, because the CTAP image is not disturbed by respiratory artifacts, it presents true ring enhancement, which eliminates the possibility of the HCC lesions. Choi et al. (26) reported the inconsistency of arterial phase enhancement between MRI and CT, and speculated that the reasons for this may include the following: the difference in the underlying contrast-enhancement mechanisms between MR imaging and CT, better soft-tissue contrast in MR imaging compared to CT, narrower AP time window in MRI leading to difficulty in acquiring optimal AP MR images, and higher susceptibility to respiratory motion artifacts in MR imaging compared to CT. However, Choi et al. did not further explore the impact of replacing MRAP images with CTAP images on the diagnosis of HCC. Our results showed that CTAP was more effective in judging the arterial phase enhancement mode of HCCs than gadoxetic acid-enhanced MRAP, which may be helpful for discerning HCCs.
This study had several limitations. First, most of the lesions recruited in this study were HCCs, and the use of CTAP images combined with MRAP images for the diagnostic efficacy of non-HCCs needs to be further confirmed. However, the proportion of lesion types we included exactly fit the current clinical situation in China. Second, the different parameters of the various MRI/CT machines in our study may have affected data consistency. However, our parameter optimization conducted for each machine to archive the best image quality in the early stages of this prospective study can avoid development of the bias seen in the results. Third, the diagnostic criteria used in our research were the LI-RADS, EASL, and Korean criteria, which led to uncertainty regarding whether gadoxetic acid-enhanced MRI combined with CTAP images can improve the efficacy of other diagnostic criteria for HCCs. However, the 3 diagnostic criteria we used were the international mainstream diagnostic criteria, which reflected evaluation of the diagnostic efficacy of HCCs, thereby affecting clinical diagnosis and treatment.
Conclusions
In conclusion, APHE in CTAP images showed a significantly higher detection rate than in MRAP images. The combined use of dynamic-enhanced CT vascular features for HCC diagnosis produced high sensitivity and comparable specificity when compared with the gadoxetic acid-enhanced MRI only.
Acknowledgments
Funding: This work was supported by National Natural Science Foundation of China (grant No. 82202117), Science and Technology Support Program of Sichuan Province (No. 2020YFS0121 and No. 2021YFS0144), China Postdoctoral Science Foundation (No. 2021M692289), and Post-Doctor Research Project of West China Hospital of Sichuan University (No. 2020HXBH130).
Footnote
Reporting Checklist: The authors have completed the STARD reporting checklist. Available at https://atm.amegroups.com/article/view/10.21037/atm-22-4968/rc
Data Sharing Statement: Available at https://atm.amegroups.com/article/view/10.21037/atm-22-4968/dss
Conflicts of Interest: All authors have completed the ICMJE uniform disclosure form (available at https://atm.amegroups.com/article/view/10.21037/atm-22-4968/coif). XW and LN are from GE Healthcare China. The other authors have no conflicts of interest to declare.
Ethical Statement: The authors are accountable for all aspects of the work in ensuring that questions related to the accuracy or integrity of any part of the work are appropriately investigated and resolved. The study was conducted in accordance with the Declaration of Helsinki (as revised in 2013). This study was approved by the Institutional Review Board of West China Hospital [No. 2016(297)]. Due to the study’s retrospective nature, the requirement for written informed consent was waived.
Open Access Statement: This is an Open Access article distributed in accordance with the Creative Commons Attribution-NonCommercial-NoDerivs 4.0 International License (CC BY-NC-ND 4.0), which permits the non-commercial replication and distribution of the article with the strict proviso that no changes or edits are made and the original work is properly cited (including links to both the formal publication through the relevant DOI and the license). See: https://creativecommons.org/licenses/by-nc-nd/4.0/.
References
- Bashir MR, Castelli P, Davenport MS, et al. Respiratory motion artifact affecting hepatic arterial phase MR imaging with gadoxetate disodium is more common in patients with a prior episode of arterial phase motion associated with gadoxetate disodium. Radiology 2015;274:141-8. [Crossref] [PubMed]
- Davenport MS, Viglianti BL, Al-Hawary MM, et al. Comparison of acute transient dyspnea after intravenous administration of gadoxetate disodium and gadobenate dimeglumine: effect on arterial phase image quality. Radiology 2013;266:452-61. [Crossref] [PubMed]
- Bruix J, Sherman MAmerican Association for the Study of Liver Diseases. Management of hepatocellular carcinoma: an update. Hepatology 2011;53:1020-2. [Crossref] [PubMed]
- European Association for Study of Liver. EASL-EORTC clinical practice guidelines: management of hepatocellular carcinoma. Eur J Cancer 2012;48:599-641. [Crossref] [PubMed]
- Radiology. ACo. CT/MRI LI-RADS v2018 core. Available online: https://www.acr.org/-/media/ACR/Files/RADS/LI-RADS/LI-RADS-2018-Core.pdf. Accessed December 4, 2018.
- Chernyak V, Flusberg M, Berman J, et al. Liver Imaging Reporting and Data System Version 2018: Impact on Categorization and Hepatocellular Carcinoma Staging. Liver Transpl 2019;25:1488-502. [Crossref] [PubMed]
- Moura Cunha G, Chernyak V, Fowler KJ, et al. Up-to-Date Role of CT/MRI LI-RADS in Hepatocellular Carcinoma. J Hepatocell Carcinoma 2021;8:513-27. [Crossref] [PubMed]
- Haradome H, Grazioli L, Tsunoo M, et al. Can MR fluoroscopic triggering technique and slow rate injection provide appropriate arterial phase images with reducing artifacts on gadoxetic acid-DTPA (Gd-EOB-DTPA)-enhanced hepatic MR imaging? J Magn Reson Imaging 2010;32:334-40. [Crossref] [PubMed]
- Ippolito D, Maino C, Pecorelli A, et al. Influence of injection rate in determining the development of artifacts during the acquisition of dynamic arterial phase in Gd-EOB-DTPA MRI studies. MAGMA 2021;34:133-40. [Crossref] [PubMed]
- Gruber L, Rainer V, Plaikner M, et al. CAIPIRINHA-Dixon-TWIST (CDT)-VIBE MR imaging of the liver at 3.0T with gadoxetate disodium: a solution for transient arterial-phase respiratory motion-related artifacts? Eur Radiol 2018;28:2013-21. [Crossref] [PubMed]
- Wei Y, Deng L, Yuan Y, et al. Gadoxetate acid disodium-enhanced MRI: Multiple arterial phases using differential sub-sampling with cartesian ordering (DISCO) may achieve more optimal late arterial phases than the single arterial phase imaging. Magnetic Resonance Imaging 2019;61:116-23. [Crossref] [PubMed]
- Min JH, Kim YK, Kang TW, et al. Artifacts during the arterial phase of gadoxetate disodium-enhanced MRI: Multiple arterial phases using view-sharing from two different vendors versus single arterial phase imaging. Eur Radiol 2018;28:3335-46. [Crossref] [PubMed]
- Basha MAA, AlAzzazy MZ, Ahmed AF, et al. Does a combined CT and MRI protocol enhance the diagnostic efficacy of LI-RADS in the categorization of hepatic observations? A prospective comparative study. Eur Radiol 2018;28:2592-603. [Crossref] [PubMed]
- Kim M, Kang TW, Cha DI, et al. Identification of Arterial Hyperenhancement in CT and MRI in Patients with Hepatocellular Carcinoma: Value of Unenhanced Images. Korean J Radiol 2019;20:236-45. [Crossref] [PubMed]
- Park SH, Kim B, Kim SY, et al. Abbreviated MRI with optional multiphasic CT as an alternative to full-sequence MRI: LI-RADS validation in a HCC-screening cohort. Eur Radiol 2020;30:2302-11. [Crossref] [PubMed]
- Hayashi M, Matsui O, Ueda K, et al. Correlation between the blood supply and grade of malignancy of hepatocellular nodules associated with liver cirrhosis: evaluation by CT during intraarterial injection of contrast medium. AJR Am J Roentgenol 1999;172:969-76. [Crossref] [PubMed]
- Kwon HJ, Byun JH, Kim JY, et al. Differentiation of small (≤2 cm) hepatocellular carcinomas from small benign nodules in cirrhotic liver on gadoxetic acid-enhanced and diffusion-weighted magnetic resonance images. Abdom Imaging 2015;40:64-75. [Crossref] [PubMed]
- Choi SH, Kim SY, Lee SS, et al. Subtraction Images of Gadoxetic Acid-Enhanced MRI: Effect on the Diagnostic Performance for Focal Hepatic Lesions in Patients at Risk for Hepatocellular Carcinoma. AJR Am J Roentgenol 2017;209:584-91. [Crossref] [PubMed]
- Khalili K, Kim TK, Jang HJ, et al. Optimization of imaging diagnosis of 1-2 cm hepatocellular carcinoma: an analysis of diagnostic performance and resource utilization. J Hepatol 2011;54:723-8. [Crossref] [PubMed]
- Kim TK, Lee KH, Jang HJ, et al. Analysis of gadobenate dimeglumine-enhanced MR findings for characterizing small (1-2-cm) hepatic nodules in patients at high risk for hepatocellular carcinoma. Radiology 2011;259:730-8. [Crossref] [PubMed]
- An C, Park MS, Kim D, et al. Added value of subtraction imaging in detecting arterial enhancement in small (<3 cm) hepatic nodules on dynamic contrast-enhanced MRI in patients at high risk of hepatocellular carcinoma. Eur Radiol 2013;23:924-30. [Crossref] [PubMed]
- Park MJ, Kim YK, Lee MH, et al. Validation of diagnostic criteria using gadoxetic acid-enhanced and diffusion-weighted MR imaging for small hepatocellular carcinoma (<= 2.0 cm) in patients with hepatitis-induced liver cirrhosis. Acta Radiol 2013;54:127-36. [Crossref] [PubMed]
- Yu MH, Kim JH, Yoon JH, et al. Small (≤1-cm) hepatocellular carcinoma: diagnostic performance and imaging features at gadoxetic acid-enhanced MR imaging. Radiology 2014;271:748-60. [Crossref] [PubMed]
- Seale MK, Catalano OA, Saini S, et al. Hepatobiliary-specific MR contrast agents: role in imaging the liver and biliary tree. Radiographics 2009;29:1725-48. [Crossref] [PubMed]
- Fidler J, Hough D. Hepatocyte-specific magnetic resonance imaging contrast agents. Hepatology 2011;53:678-82. [Crossref] [PubMed]
- Choi SH, Lee SS, Kim SY, et al. Intrahepatic Cholangiocarcinoma in Patients with Cirrhosis: Differentiation from Hepatocellular Carcinoma by Using Gadoxetic Acid-enhanced MR Imaging and Dynamic CT. Radiology 2017;282:771-81. [Crossref] [PubMed]
(English Language Editor: C. Betlazar-Maseh)