TGR5 overexpression mediated by the inhibition of transcription factor SOX9 protects against hypoxia-/reoxygenation-induced injury in hippocampal neurons by activating Nrf2/HO-1 signaling
Introduction
Stroke is a major cause of disability and death worldwide (1). It has been referred to as the “incoming epidemic of the 21st century” by the World Health Organization (2). Generally, stroke is divided into the following 2 main types: (I) ischemic stroke due to lack of blood flow; (II) hemorrhagic stroke due to bleeding (3). Ischemic stroke is the most common type of stroke, and accounts for 80% of all strokes (4). During ischemic stroke, a series of harmful cascades occur, including oxidative stress and an inflammatory response, which may eventually result in apoptosis (5). To date, the restoration of blood reperfusion and the resupply of nutrients remain the most efficient treatments for stroke (6). However, cerebral ischemia/reperfusion (CI/R) injury presents a serious complication, which complicates therapy for ischemic stroke patients (7). Thus, novel therapeutic modalities need to be developed to reduce CI/R injury as a pre-requisite for protecting brain functions in ischemic stroke patients.
TGR5 is a G protein-coupled bile acid receptor belonging to the G-protein-coupled receptor (GPCR) superfamily (8). Numerous studies have shown that TGR5 is expressed in various organs and widely participates in energy homeostasis and metabolism (9-11). More importantly, recent studies on TGR5 have suggested that TGR5 ameliorates inflammation in liver ischemia/reperfusion (I/R) injury (12,13). TGR5 could alleviated liver steatosis and inflammation in nonalcoholic steatohepatitis (14) and TGR5 antagonized kidney inflammation in lipopolysaccharide (LPS)-induced injury (15). It can be seen that TGR5 has a alleviating effect on inflammation. Li et al. conjectured that TGR5 mediates the protein kinase B/glycogen synthase kinase-3β pathway to prevent myocardial I/R injury (16). TGR5 activated by Farnesiferol B was shown to alleviate I/R-triggered kidney damage (17). However, little is known about the effect of TGR5 on CI/R injury.
JASPAR predicts binding of the transcription factor SRY-box transcription factor 9 (SOX9) to the TGR5 promoter. As a member of the SOX family, SOX9 plays pivotal roles in multiple tumors and diseases by regulating the transcription of diverse genes (18). To date, research has primarily focused on the oncogenic roles of SOX9 in malignant tumors (19,20). Notably, recent research on SOX9 shows that SOX9 expression is increased in CI/R rats and the inhibition of SOX9 mitigates CI/R injury (21,22). Additionally, the elevation of SOX9 is considered a predominant driver of ischemic brain injury (23). Elevated SOX9 promotes hepatic ischemia/reperfusion injury through activation of TGF-β1 (24). However, very little research has been conducted on the interaction between SOX9 and TGR5 in CI/R injury.
Nrf2/HO-1 signaling is known as primary antioxidant signaling. There is increasing evidence that Nrf2/HO-1 signaling regulates oxidative stress and is involved in multiple human diseases, such as preeclampsia (25), acute lung injury (26), and myocardial I/R injury (27). Further, there is emerging evidence that the activation of Nrf2/HO-1 signaling plays a protective role in CI/R injury (28-30). However, the specific association between TGR5 and the Nrf2/HO-1 signaling pathway in CI/R injury has not been examined.
In this study, a hypoxia/reoxygenation (H/R) model was constructed in HT22 cells to examine the effect of TGR5 on CI/R injury and the relationship among TGR5, SOX9, and Nrf2/HO-1 signaling in CI/R injury. We present the following article in accordance with the MDAR reporting checklist (available at https://atm.amegroups.com/article/view/10.21037/atm-22-5225/rc).
Methods
Bioinformatics tools
The JASPAR database (https://jaspar.genereg.net/) was used to predict the binding relationship between SOX9 and the TGR5 promoter.
Cell culture and H/R
The mouse hippocampal HT22 cell line (cat. No. BNCC358041) acquired from BeNa Culture Collection (BNCC, Kunshan, China) was grown in Dulbecco’s modified Eagle’s medium (Saimike, Chongqing, China) with 10% fetal bovine serum (Lonza Group, Ltd.), 100 U/mL of penicillin, and 100 µg/mL of streptomycin as supplements at 37 ℃ in an atmosphere of 5% carbon dioxide (CO2). For the H/R treatment, the HT22 cells were cultured under hypoxic conditions (5% CO2 and 95% nitrogen) at 37 ℃ for 24 h, after which, the cells were transferred to a normal humidified incubator at 37 ℃ for 24 h under normal oxygen conditions (95% air and 5% CO2) (31). The cells that were incubated at 37 ℃ with 95% air and 5% CO2 were used as the control group.
Plasmid transfection
The PcDNA3.1 vector containing full-length open reading frame (ORF) human TGR5 (pcDNA3.1-TGR5) and corresponding negative control (pcDNA3.1), and the SOX9-specific overexpression vector (Oe-SOX9) and corresponding negative control (Oe-NC), were obtained from Shanghai GenePharma Co., Ltd. Plasmid transfection was conducted using Lipofectamine 3000 (Carlsbad Life Technologies) in accordance with the manufacturer’s instructions. The cells were harvested, and the transfection efficiency was tested via reverse transcription-quantitative polymerase chain reaction (RT-qPCR) and western blots 48 h post-transfection. HT22 cells were transfected with recombined vectors before H/R treatment.
RT-qPCR
To obtain the complementary DNA, total RNA, which had been isolated from the HT22 cells using TRIzol Reagent (Sagon Biotech, Shanghai, China), was reverse transcribed using the QuantiTect RT kit (Qiagen GmbH). PCR was performed using the Fast Start Universal SYBR Green Master mix (Roche Applied Science) on a 7900HT system (Applied Biosystems, CA, USA). The PCR procedure was as follows: denaturation at 94 ℃ for 2 min, amplification at 94 ℃ for 30 sec (30 cycles), annealing at 58 ℃ for 30 sec, extension at 72 ℃ for 1 min, and terminal elongation at 72 ℃ for 10 min. Relative gene expression was estimated using the 2–ΔΔCq method (32), which designated glyceraldehyde 3-phosphate dehydrogenase (GAPDH) as the internal reference.
Western blot
For the detection of nuclear factor erythroid 2-related factor 2 (Nrf2), nucleoprotein and cytoplasmic protein extraction kit obtained from Beyotime (Cat. No. P0028) was used to extract the nucleoprotein and cytoplasmic proteins from the transfected HT22 cells. RIPA cell lysis buffer (Selleck Chemicals) was employed to lyse the transfected HT22 cells and protein quantification was conducted using a BCA protein assay kit (Vazyme). After being segregated by 10% sodium dodecyl sulfate polyacrylamide gel electrophoresis (SDS-PAGE), the proteins were loaded onto PVDF membranes, which were then blocked using 5% non-fat milk. The primary antibodies specific for TGR5 (Abcam, 1:1,000, ab72608), BCL-2 associated X (Bax; Abcam, 1:1,000, ab32503), B-cell lymphoma 2 (Bcl-2; Abcam, 1:1,000, ab194583), cleaved caspase-3 (Cell Signaling Technology, 1:1,000, #9664), cleaved-PARP (Cell Signaling Technology, 1:1,000, #94885), SOX9 (Abcam, 1:1,000, ab185966), Nrf2 (Abcam, 1:1,000, ab92946), HO-1 (Abcam, 1:2000, ab52947), GAPDH (Abcam, 1:10,000, ab181602) were added to the membranes to be incubated overnight at 4 ℃ and then the HRP-conjugated secondary antibody (Abcam, 1:1,000, ab133470) was added to the membranes to be incubated at room temperature for 1 h. The blots were visualized using the enhanced chemiluminescence system (Clinx Science Instruments Co., Ltd., Shanghai, China), and the signal intensity was measured using ImageJ software (version 1.48v; National Institutes of Health).
Cell Counting Kit-8 (CCK-8) assays
The transfected HT22 cells (5×103/well) were seeded into 96-well plates and maintained at 37 ℃ overnight. The cells were cultivated for another 2 h at 37 ℃ after the addition of 10 µL of CCK-8 solution (Beijing Transgen Biotech Co., Ltd.). With the aid of a microplate reader (Dynex Technologies), the absorbance was read at 450 nm.
Detection of lactate dehydrogenase (LDH) release
Briefly, a LDH assay kit (C0016, Beyotime Institute of Biotechnology) was used to detect the LDH concentration in the HT22 cells. In accordance with the manufacturer's instructions, after centrifugation at 400 ×g for 10 min, the cell supernatant was collected. Next, 60 µL of the supernatant was mixed with 30 µL of the LDH substrate solution, and the cells were incubated for additional 30 min at 37 ℃. LDH activity was measured with a microplate reader (Dynex Technologies) at 450 nm.
TUNEL
The apoptotic rate of the HT22 cells was quantified by the terminal deoxynucleotidyl transferase (TdT)-mediated dUTP nick-end labeling (TUNEL) apoptosis kit (Nanjing Biobox Biotech Co., Ltd.) in accordance with the manufacturer’s instructions. In brief, the transfected HT22 cells were immobilized with 4% paraformaldehyde and permeabilized with 0.1% Triton X-100 for 8 min. Next, the cells were incubated with 50 µL of TUNEL assay solution for 1 h, and the nuclei were labeled by DAPI (Koritai Biotechnology, Beijing, China) for 10 min. Finally, the images were captured under a fluorescence microscope (magnification, ×200; UltraVIEW VoX; PerkinElmer, Inc.).
IF staining
In brief, 24 h post H/R treatment, the HT22 cells were immobilized with 4% paraformaldehyde (PFA) solution, and permeabilized with 0.2% Triton X-100 for 10 min. A primary antibody against cytochrome c (cyto-c; Abcam, 1:100, ab133504) was added to label the cells overnight at 4 ℃, and a goat secondary antibody to rabbit immunoglobulin G (IgG) (Alexa Fluor 488; Abcam, 1:1,000, ab150081) was used. DAPI (Koritai Biotechnology, Beijing, China) was used for the nuclear staining. The images were photographed with a fluorescence microscope (UltraVIEW VoX; PerkinElmer, Inc.).
Measurement of malondialdehyde (MDA), glutathione peroxidase (GSH-Px), and superoxide dismutase (SOD)
The cells were decomposed in 300 µL of lysis buffer, and the total protein was quantified using a BCA protein assay kit (Vazyme). The corresponding kits from Nanjing Jiancheng Bioengineering Co. Ltd. were used to determine the levels of MDA (A003-1-2), GSH-Px (A005-1-2), and SOD (A001-1-2) in accordance with the manufacturer’s instructions. The absorbance was determined at 450 nm using a microplate reader (Dynex Technologies).
ChIP
The EZ-Magna ChIP A/G kit (MilliporeSigma) was used for the chromatin immunoprecipitation (ChIP) assays. The HT22 cells were sonicated into 200–1,000 bp DNA fragments and centrifuged at 300 ×g for 3 min at 25 ℃. Next, the SOX9 antibody (Abcam, 1:60, ab185966) or the IgG antibody (Beyotime Institute of Biotechnology, 1 µg/µL, A7016) was used for immunoprecipitation.
Luciferase reporter assays
The sequences of TGR5 promoter region were inserted into the pGL3 basic vector (Laboratory of Anhui Medical University) to construct TGR5-WT (CCATTGGTC) and TGR5-MUT (AACGGAAGA), after which, they were co-transfected with Oe-SOX9 and Oe-NC into the HT22 cells via Lipofectamine 3000 (Carlsbad Life Technologies). After 48 h, the luciferase assay system (Ambion, Austin, TX, USA) was employed to estimate the luciferase activity.
Statistical analysis
All the statistical analyses were conducted using SPSS 22.0 (Chicago, Illinois, USA). The experimental data were presented as the mean ± standard deviation (SD) and were biologically repeated in triplicate. Differences between the groups were evaluated by the Student’s t-test or a 1-way analysis of variance followed by a Tukey’s post-hoc test. A P value <0.05 was considered statistically significant.
Results
Elevation of TGR5 contributes to HT22 cell viability after H/R
To assess the effect of TGR5 on CI/R injury, TGR5 expression was examined following H/R treatment in the HT22 cells. As Figure 1A,1B show, the RT-qPCR and western blots indicated that TGR5 expression was sharply reduced in the H/R-induced HT22 cells compared to the control group. After the transfection of pcDNA3.1-TGR5, the overexpression efficiency was tested, and TGR5 expression was found to be significantly increased (Figure 1C,1D). The experimental results of the CCK-8 assays revealed that H/R treatment significantly suppressed the viability of the HT22 cells, and when TGR5 was upregulated, the viability of the H/R-induced HT22 cells was significantly increased (Figure 1E). Further, LDH release was enhanced in the HT22 cells after H/R treatment, after which, the overexpression of TGR5 led to a decrease in LDH release (Figure 1F). Collectively, TGR5 was downregulated in the H/R-induced HT22 cells and decreased the viability of H/R-induced HT22 cells.
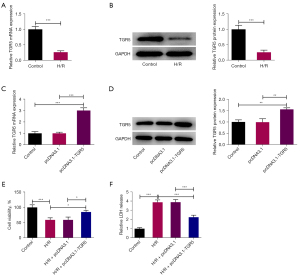
TGR5 overexpression suppresses the apoptosis of HT22 cells under H/R conditions
Conversely, the TUNEL assays revealed that H/R treatment significantly increased the apoptotic rate of the HT22 cells, but this effect was decreased after TGR5 was overexpressed (Figure 2A). The mitochondrial release of cyto-c has been confirmed as a hallmark of neuronal death (33). The immunofluorescence assays revealed that the increased cyto-c level was decreased by the upregulation of TGR5 (Figure 2B). Additionally, the western blots showed that the Bcl-2 protein level declined, while the Bax, cleaved caspase-3 and cleaved-PARP protein levels increased in the HT22 cells under H/R conditions, and TGR5 elevation increased the protein level of Bcl-2 but further decreased the protein levels of Bax, cleaved caspase-3, and cleaved-PARP (Figure 2C,2D). Thus, these results suggest that TGR5 played a suppressive role in the H/R-induced apoptosis in the HT22 cells.
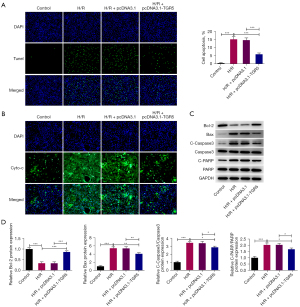
TGR5 upregulation reduces H/R-evoked oxidative stress in HT22 cells
To explore the effect of TGR5 on oxidative stress in the H/R-induced HT22 cells, the levels of oxidative stress markers, including SOD, GSH-Px, and MDA, were evaluated by corresponding kits, and we discovered that TGR5 increased the downregulated SOD and GSH-Px levels and decreased the upregulated MDA level in the H/R-treated HT22 cells (Figure 3). In summary, the elevation of TGR5 mitigated oxidative stress in HT22 cells under H/R conditions.
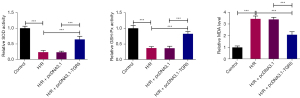
TGR5 is transcriptionally inhibited by SOX9
According to the JASPAR database, we hypothesized that SOX9 would have a binding relationship with the TGR5 promoter and predicted possible binding sites (Figure 4A). The RT-qPCR and western blots also showed that SOX9 was highly expressed in the H/R-induced HT22 cells compared to the control group (Figure 4B,4C). Meanwhile, after SOX9 overexpression following the transfection of the Oe-SOX9 plasmid (Figure 4D,4E), the luciferase reporter assays showed that SOX9 overexpression notably reduced the luciferase activity of TGR5-WT but not TGR5-MUT (Figure 4F). Additionally, the results of the ChIP assays revealed that the TGR5 promoter was highly abundant in the SOX9 antibody, which suggested that the TGR5 promoter had a strong affinity with SOX9 (Figure 4G). Moreover, the increased expression of TGR5 after the transfection of pcDNA3.1-TGR5 plasmid was decreased in the H/R-induced HT22 cells when SOX9 was upregulated (Figure 4H,4I). Overall, SOX9 was an upstream transcription factor of TGR5 and transcriptionally suppressed TGR5 expression.
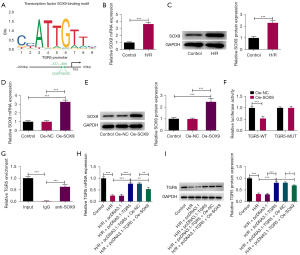
Upregulation of SOX9 reverses the inhibitory role of TGR5 in H/R-triggered HT22 cell injury
To further determine the effects of the SOX9/TGR5 axis in the H/R-induced HT22 cells, pcDNA3.1-TGR5 and Oe-SOX9 plasmids were co-transfected into the H/R-induced HT22 cells and functional experiments were then conducted. The CCK-8 assays showed that the stimulated viability of the H/R-induced HT22 cells generated by TGR5 overexpression was restored when SOX9 was upregulated (Figure 5A). In addition, the increased SOD and GSH-Px levels, the falling MDA level and LDH release resulting from TGR5 were reversed by SOX9 in the H/R-treated HT22 cells (Figure 5B). As Figure 5C shows, the TUNEL assays confirmed that the upregulation of TGR5 impaired the apoptotic capacity of the HT22 cells under H/R conditions, but this effect was reversed by SOX9. Similarly, the decrease in cyto-c release due to TGR5 was also reversed after SOX9 was overexpressed (Figure 5D). Similarly, SOX9 elevation offset the upregulation on Bcl-2 protein level and the downregulation on Bax, cleaved caspase-3 and cleaved-PARP protein levels (Figure 5E). Taken together, our results showed that the effects of TGR5 on H/R-treated HT22 cells were all reversed by SOX9.
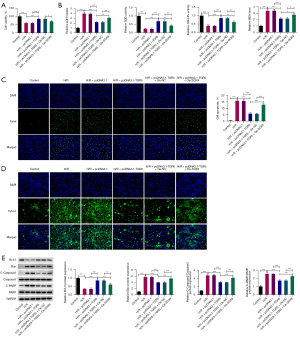
TGR5 elevation mediated by SOX9 interference activates Nrf2/HO-1 signaling
To examine the role of Nrf2/HO-1 signaling in SOX9/TGR5 axis-mediated HT22 cell injury under H/R conditions, the protein levels of related factors in Nrf2/HO-1 signaling were detected by western blots. The H/R treatment increased the Nrf2 protein level in the cytoplasm but decreased the Nrf2 protein level in the nucleus and HO-1 protein level. After TGR5 was overexpressed, Nrf2 expression was decreased in the cytoplasm but both Nrf2 expression in the nucleus and HO-1 expression were increased, and this effect was in turn reversed by SOX9 (Figure 6). Thus, SOX9 silencing-mediated TGR5 elevation led to the activation of Nrf2/HO-1 signaling.
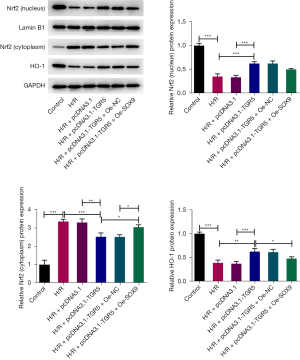
Discussion
Cerebral ischemic stroke is generally acknowledged to be one of the most under-treated serious diseases and has high mortality and disability rates globally (1,34). Despite great advancements in therapy for ischemic stroke, the therapeutic options remain limited (3,35). During the course of cerebral ischemic stroke, the existence of I/R injury may contribute to blood-brain-barrier disruption, neuron death, and the deterioration of cerebral infarction (36,37). Thus, effective therapeutic measures that protect the brain from CI/R injury need to be established. Oxidative stress, which frequently occurs following CI/R, may cause further damage to the brain tissue in ischemic stroke by stimulating cell death (38). In this study, a H/R model was first established in the HT22 cells. We then explored the proliferation, apoptosis, and oxidative stress of H/R-induced HT22 cells to gain further insights into the pathogenesis of CI/R injury.
In recent years, the role of TGR5, which was the first identified G-coupled protein receptor specific for bile acids, has received extensive attention in relation to the regulation of metabolic syndrome and related disorders (8,39,40). For example, Huang et al. showed that the TGR5 agonist enhances glucose homeostasis in diabetes (41). While Guo et al. suggested that TGR5 ameliorates gastric inflammation by suppressing the nuclear factor kappa B signaling pathway (42). Interestingly, there is extensive evidence that TGR5 alleviates I/R injury in the liver (12,43), myocardium (16), and kidney (17). Consistent with these findings, TGR5 was found to be significantly downregulated in the HT22 cells under H/R conditions in the present study.
Functionally, the overexpression of TGR5 facilitated the viability of the H/R-induced HT22 cells. LDH is a stable cytoplasmic enzyme, the activity of which can be determined to appraise cytotoxicity (44). The experimental results in this study showed that the stimulated LDH release decreased after TGR5 was overexpressed. Additionally, TGR5 elevation significantly reduced the apoptotic rate of the H/R-treated HT22 cells. Cyto-c is a multifunctional enzyme associated with cell apoptosis. As anticipated, TGR5 hampered the H/R-triggered cyto-c release in the HT22 cells. Further, TGR5 upregulation increased the protein level of Bcl-2 and decreased the protein levels of Bax, cleaved caspase-3, and cleaved-PARP in the H/R-induced HT22 cells. Our study also showed that TGR5 upregulated the levels of antioxidant stress-related enzymes, including SOD and GSH-Px, but downregulated the level of the oxidative damage marker MDA.
It has been reported that SOX9 modulates the expression of some genes by functioning as a transcription factor (18). For example, SOX9 is a transcriptional regulator of TSPAN8 in pancreatic cancer (45). FOXA1 is also dependent on the SOX9 transcription factor in lung carcinoma (46). In this study, SOX9 expression was found to be increased in the H/R-induced HT22 cells. The binding between SOX9 and TGR5 promoter was predicted using the JASPAR database and proven by mechanism assays. Additionally, TGR5 expression in the HT22 cells transfected with pcDNA3.1-TGR5 plasmid under H/R conditions was reduced when SOX9 was upregulated, implying that TGR5 was transcriptionally inhibited by SOX9. It should be noted that numerous studies have emphasized the significance of SOX9 in CI/R injury, and all these studies have suggested that SOX9 primarily serves as a promoter in CI/R injury (21-23). Our findings also suggested that the increased viability and the decreased LDH release, apoptosis, cyto-c release, and oxidative stress in the H/R-treated HT22 cells due to TGR5 overexpression were all reversed by SOX9.
Transcription factor Nrf2 provides adaptive protection against oxidative stress by activating the transcription of the antioxidant response element-driven gene HO-1 in the cell nucleus (47). More importantly, the dysregulation of Nrf2/HO-1 signaling is associated with CI/R injury (28,29,48). TGR5 has also been shown to act as an activator of Nrf2/HO-1 signaling in cholestatic liver disease (49) and high glucose-stimulated cardiomyocyte injury (50). Similarly, our results also indicated that Nrf2 expression was increased in the cytoplasm but decreased in the nucleus and HO-1 expression was also decreased in the HT22 cells following H/R treatment. Additionally, the induced translocation of Nrf2 from the cytoplasm to the nucleus and HO-1 expression due to TGR5 were restored by SOX9 overexpression.
Conclusions
In conclusion, TGR5 overexpression mediated by the inhibition of SOX9 increased the viability but decreased the apoptosis and oxidative stress of the H/R-treated HT22 cells by activating Nrf2/HO-1 signaling. Our findings might lead to the identification of a novel molecular mechanism of SOX9/TGR5/Nrf2/HO-1 signaling in CI/R injury. However, there was also existed limitation in this study. The effects of TGR5 on CI/R injury in animal models needed to be explored in future study.
Acknowledgments
Funding: None.
Footnote
Reporting Checklist: The authors have completed the MDAR reporting checklist. Available at https://atm.amegroups.com/article/view/10.21037/atm-22-5225/rc
Data Sharing Statement: Available at https://atm.amegroups.com/article/view/10.21037/atm-22-5225/dss
Conflicts of Interest: All authors have completed the ICMJE uniform disclosure form (available at https://atm.amegroups.com/article/view/10.21037/atm-22-5225/coif). The authors have no conflicts of interest to declare.
Ethical Statement: The authors are accountable for all aspects of the work in ensuring that questions related to the accuracy or integrity of any part of the work are appropriately investigated and resolved.
Open Access Statement: This is an Open Access article distributed in accordance with the Creative Commons Attribution-NonCommercial-NoDerivs 4.0 International License (CC BY-NC-ND 4.0), which permits the non-commercial replication and distribution of the article with the strict proviso that no changes or edits are made and the original work is properly cited (including links to both the formal publication through the relevant DOI and the license). See: https://creativecommons.org/licenses/by-nc-nd/4.0/.
References
- Kuriakose D, Xiao Z. Pathophysiology and Treatment of Stroke: Present Status and Future Perspectives. Int J Mol Sci 2020;21:7609. [Crossref] [PubMed]
- Sarikaya H, Ferro J, Arnold M. Stroke prevention--medical and lifestyle measures. Eur Neurol 2015;73:150-7. [Crossref] [PubMed]
- Paul S, Candelario-Jalil E. Emerging neuroprotective strategies for the treatment of ischemic stroke: An overview of clinical and preclinical studies. Exp Neurol 2021;335:113518. [Crossref] [PubMed]
- Boursin P, Paternotte S, Dercy B, et al. Semantics, epidemiology and semiology of stroke. Soins 2018;63:24-7. [Crossref] [PubMed]
- Juan WS, Lin HW, Chen YH, et al. Optimal Percoll concentration facilitates flow cytometric analysis for annexin V/propidium iodine-stained ischemic brain tissues. Cytometry A 2012;81:400-8. [Crossref] [PubMed]
- Matveev DV, Kuznetsov MR, Matveev AD, et al. Reperfusion syndrome: state of the art. Angiol Sosud Khir 2020;26:176-83. [Crossref] [PubMed]
- Yu S, Zhai J, Yu J, et al. miR-98-5p protects against cerebral ischemia/reperfusion injury through anti-apoptosis and anti-oxidative stress in mice. J Biochem 2021;169:195-206. [Crossref] [PubMed]
- Duboc H, Taché Y, Hofmann AF. The bile acid TGR5 membrane receptor: from basic research to clinical application. Dig Liver Dis 2014;46:302-12. [Crossref] [PubMed]
- Deutschmann K, Reich M, Klindt C, et al. Bile acid receptors in the biliary tree: TGR5 in physiology and disease. Biochim Biophys Acta Mol Basis Dis 2018;1864:1319-25. [Crossref] [PubMed]
- Zhang MY, Zhu L, Zheng X, et al. TGR5 Activation Ameliorates Mitochondrial Homeostasis via Regulating the PKCδ/Drp1-HK2 Signaling in Diabetic Retinopathy. Front Cell Dev Biol 2021;9:759421. [Crossref] [PubMed]
- van Nierop FS, Scheltema MJ, Eggink HM, et al. Clinical relevance of the bile acid receptor TGR5 in metabolism. Lancet Diabetes Endocrinol 2017;5:224-33. [Crossref] [PubMed]
- Zhuang L, Ding W, Zhang Q, et al. TGR5 Attenuated Liver Ischemia-Reperfusion Injury by Activating the Keap1-Nrf2 Signaling Pathway in Mice. Inflammation 2021;44:859-72. [Crossref] [PubMed]
- Zhou H, Zhou S, Shi Y, et al. TGR5/Cathepsin E signaling regulates macrophage innate immune activation in liver ischemia and reperfusion injury. Am J Transplant 2021;21:1453-64. [Crossref] [PubMed]
- Shi Y, Su W, Zhang L, et al. TGR5 Regulates Macrophage Inflammation in Nonalcoholic Steatohepatitis by Modulating NLRP3 Inflammasome Activation. Front Immunol 2020;11:609060. [Crossref] [PubMed]
- Su J, Zhang Q, Qi H, et al. The G-protein-coupled bile acid receptor Gpbar1 (TGR5) protects against renal inflammation and renal cancer cell proliferation and migration through antagonizing NF-κB and STAT3 signaling pathways. Oncotarget 2017;8:54378-87. [Crossref] [PubMed]
- Li J, Cheng R, Wan H. Overexpression of TGR5 alleviates myocardial ischemia/reperfusion injury via AKT/GSK-3β mediated inflammation and mitochondrial pathway. Biosci Rep 2020;40:BSR20193482. [Crossref] [PubMed]
- Zhang L, Fu X, Gui T, et al. Effects of Farnesiferol B on Ischemia-Reperfusion-Induced Renal Damage, Inflammation, and NF-κB Signaling. Int J Mol Sci 2019;20:6280. [Crossref] [PubMed]
- Girardot M, Bayet E, Maurin J, et al. SOX9 has distinct regulatory roles in alternative splicing and transcription. Nucleic Acids Res 2018;46:9106-18. [Crossref] [PubMed]
- Panda M, Tripathi SK, Biswal BK. SOX9: An emerging driving factor from cancer progression to drug resistance. Biochim Biophys Acta Rev Cancer 2021;1875:188517. [Crossref] [PubMed]
- Grimm D, Bauer J, Wise P, et al. The role of SOX family members in solid tumours and metastasis. Semin Cancer Biol 2020;67:122-53. [Crossref] [PubMed]
- Zhang M, Zhu Y, Wei M, et al. Neuroprotective effects of miR-30c on rats with cerebral ischemia/reperfusion injury by targeting SOX9. Pathol Res Pract 2020;216:153271. [Crossref] [PubMed]
- Yang B, Nie Y, Wang L, et al. Flurbiprofen axetil protects against cerebral ischemia/reperfusion injury via regulating miR-30c-5p and SOX9. Chem Biol Drug Des 2022;99:197-205. [Crossref] [PubMed]
- Chang L, An Z, Zhang J, et al. H3K27 demethylase KDM6B aggravates ischemic brain injury through demethylation of IRF4 and Notch2-dependent SOX9 activation. Mol Ther Nucleic Acids 2021;24:622-33. [Crossref] [PubMed]
- Fan XD, Zheng HB, Fan XS, et al. Increase of SOX9 promotes hepatic ischemia/reperfusion (IR) injury by activating TGF-β1. Biochem Biophys Res Commun 2018;503:215-21. [Crossref] [PubMed]
- Guo H, Wang Y, Jia W, et al. MiR-133a-3p relieves the oxidative stress induced trophoblast cell apoptosis through the BACH1/Nrf2/HO-1 signaling pathway. Physiol Res 2021;70:67-78. [Crossref] [PubMed]
- Bi XG, Li ML, Xu W, et al. Helix B surface peptide protects against acute lung injury through reducing oxidative stress and endoplasmic reticulum stress via activation of Nrf2/HO-1 signaling pathway. Eur Rev Med Pharmacol Sci 2020;24:6919-30. [PubMed]
- Ding M, Li M, Zhang EM, et al. FULLEROL alleviates myocardial ischemia-reperfusion injury by reducing inflammation and oxidative stress in cardiomyocytes via activating the Nrf2/HO-1 signaling pathway. Eur Rev Med Pharmacol Sci 2020;24:9665-74. [PubMed]
- Xue Z, Zhao K, Sun Z, et al. Isorhapontigenin ameliorates cerebral ischemia/reperfusion injury via modulating Kinase Cε/Nrf2/HO-1 signaling pathway. Brain Behav 2021;11:e02143. [Crossref] [PubMed]
- Fu K, Chen M, Zheng H, et al. Pelargonidin ameliorates MCAO-induced cerebral ischemia/reperfusion injury in rats by the action on the Nrf2/HO-1 pathway. Transl Neurosci 2021;12:20-31. [Crossref] [PubMed]
- Hu L, Chen W, Tian F, et al. Neuroprotective role of fucoxanthin against cerebral ischemic/reperfusion injury through activation of Nrf2/HO-1 signaling. Biomed Pharmacother 2018;106:1484-9. [Crossref] [PubMed]
- Wang B, Yu P, Lin W, et al. MicroRNA-21-5p Reduces Hypoxia/Reoxygenation-Induced Neuronal Cell Damage through Negative Regulation of CPEB3. Anal Cell Pathol (Amst) 2021;2021:5543212. [Crossref] [PubMed]
- Livak KJ, Schmittgen TD. Analysis of relative gene expression data using real-time quantitative PCR and the 2(-Delta Delta C(T)) Method. Methods 2001;25:402-8. [Crossref] [PubMed]
- Sanderson TH, Raghunayakula S, Kumar R. Neuronal hypoxia disrupts mitochondrial fusion. Neuroscience 2015;301:71-8. [Crossref] [PubMed]
- Guo Z, Wu X, Fan W. Clarifying the effects of diabetes on the cerebral circulation: Implications for stroke recovery and beyond. Brain Res Bull 2021;171:67-74. [Crossref] [PubMed]
- Datta A, Sarmah D, Mounica L, et al. Cell Death Pathways in Ischemic Stroke and Targeted Pharmacotherapy. Transl Stroke Res 2020;11:1185-202. [Crossref] [PubMed]
- Shaik NF, Regan RF, Naik UP. Platelets as drivers of ischemia/reperfusion injury after stroke. Blood Adv 2021;5:1576-84. [Crossref] [PubMed]
- Wang YY, Chang CY, Lin SY, et al. Quercetin protects against cerebral ischemia/reperfusion and oxygen glucose deprivation/reoxygenation neurotoxicity. J Nutr Biochem 2020;83:108436. [Crossref] [PubMed]
- Orellana-Urzúa S, Rojas I, Líbano L, et al. Pathophysiology of Ischemic Stroke: Role of Oxidative Stress. Curr Pharm Des 2020;26:4246-60. [Crossref] [PubMed]
- Ma H, Patti ME. Bile acids, obesity, and the metabolic syndrome. Best Pract Res Clin Gastroenterol 2014;28:573-83. [Crossref] [PubMed]
- Stepanov V, Stankov K, Mikov M. The bile acid membrane receptor TGR5: a novel pharmacological target in metabolic, inflammatory and neoplastic disorders. J Recept Signal Transduct Res 2013;33:213-23. [Crossref] [PubMed]
- Huang S, Ma S, Ning M, et al. TGR5 agonist ameliorates insulin resistance in the skeletal muscles and improves glucose homeostasis in diabetic mice. Metabolism 2019;99:45-56. [Crossref] [PubMed]
- Guo C, Qi H, Yu Y, et al. The G-Protein-Coupled Bile Acid Receptor Gpbar1 (TGR5) Inhibits Gastric Inflammation Through Antagonizing NF-κB Signaling Pathway. Front Pharmacol 2015;6:287. [Crossref] [PubMed]
- Yang H, Zhou H, Zhuang L, et al. Plasma membrane-bound G protein-coupled bile acid receptor attenuates liver ischemia/reperfusion injury via the inhibition of toll-like receptor 4 signaling in mice. Liver Transpl 2017;23:63-74. [Crossref] [PubMed]
- Kumar P, Nagarajan A, Uchil PD. Analysis of Cell Viability by the Lactate Dehydrogenase Assay. Cold Spring Harb Protoc 2018; [Crossref] [PubMed]
- Li J, Chen X, Zhu L, et al. SOX9 is a critical regulator of TSPAN8-mediated metastasis in pancreatic cancer. Oncogene 2021;40:4884-93. [Crossref] [PubMed]
- Wang X, Yin Y, Du R. SOX9 dependent FOXA1 expression promotes tumorigenesis in lung carcinoma. Biochem Biophys Res Commun 2019;516:236-44. [Crossref] [PubMed]
- Tong F, Zhou X. The Nrf2/HO-1 Pathway Mediates the Antagonist Effect of L-Arginine On Renal Ischemia/Reperfusion Injury in Rats. Kidney Blood Press Res 2017;42:519-29. [Crossref] [PubMed]
- Ucar BI, Ucar G, Saha S, et al. Pharmacological Protection against Ischemia-Reperfusion Injury by Regulating the Nrf2-Keap1-ARE Signaling Pathway. Antioxidants (Basel) 2021;10:823. [Crossref] [PubMed]
- Yang H, Luo F, Wei Y, et al. TGR5 protects against cholestatic liver disease via suppressing the NF-κB pathway and activating the Nrf2/HO-1 pathway. Ann Transl Med 2021;9:1158. [Crossref] [PubMed]
- Deng L, Chen X, Zhong Y, et al. Activation of TGR5 Partially Alleviates High Glucose-Induced Cardiomyocyte Injury by Inhibition of Inflammatory Responses and Oxidative Stress. Oxid Med Cell Longev 2019;2019:6372786. [Crossref] [PubMed]