Corin is regulated by circ-0012397/miR-200a-3p and inhibits the oxygen-glucose deprivation-induced apoptosis of SHSY5Y neuroblastoma cells
Highlight box
Key findings
• Circ-0012397/miR-200a-3p/corin axis regulates SHSY5Y cell proliferation and inhibits OGD-induced apoptosis.
What is known and what is new?
• CircRNAs and miRNAs have proved to regulate the process of ischemic stroke.
• Circ-0012397/miR-200a-3p/corin axis also plays a key role in regulate OGD-induced SHSY5Y cell apoptosis.
What is the implication, and what should change now?
• Circ-0012397/miR-200a-3p/corin axis could be a potential target for ischemic stroke patients.
Introduction
As a major neurological disease, ischemic stroke is mainly caused by atherosclerosis, cardiac embolism, and cerebral small-vessel occlusion (1). Cerebral artery occlusion can cause cerebral ischemia and hypoxia, leading to neuronal apoptosis and brain parenchyma necrosis (2). These pathological changes may lead to neurological dysfunction, disability, or even death. Ischemic strokes are more prevalent in young people as a result of obesity, inactivity, and excessive drinking (3), making it a major disease in humans.
Neuronal apoptosis after ischemic stroke has been widely studied to salvage dysfunctional neurons (2). Generally, apoptosis caused by stroke is mediated through numerous pathways, such as the mitochondrial pathway and the protein 53 (p53)-mediated apoptosis pathway (4). In the former pathway, B-cell lymphoma 2 (BCL-2)-associated X (Bax) and BCL-2-associated death promoter (Bad) of BCL-2 family proteins are mediated in a receptor-independent manner, which leads to nuclear deoxyribonucleic acid (DNA) degradation and apoptosis (4). In the latter pathway, cell death cascades are triggered by genes involved in the mitogen-activated protein kinase (MAPK) family (4,5). These signals serve as important potential therapeutic targets to improve neuronal apoptosis after stroke.
Corin is mainly expressed in heart tissue, mediates many important physiological, and pathological processes, and is associated with an increased risk of cardiovascular disease (6). Notably, corin overexpression reduces the size of myocardial infarction and regulates the apoptosis of cardiomyocytes and the expression of Bcl-2 family proteins (7). Upon detachment from myocardial cells, corin can enter the blood stream. Thus, corin may be detected in the sera of patients with heart failure, hypertension, and obesity (8). Stroke, as a co-induced cardiovascular disease, can significantly reduce serum corin expression (9). Additionally, a deficiency in serum-soluble corin predicts severe disability in the 3 months following acute stroke (8), which suggests that corin could affect the progression of stroke. In this way, we speculate corin could influence the progress of stroke.
Circular ribonucleic acids (circRNAs) are important non-coding RNAs that are highly conserved and expressed in human cells. Recent studies have shown that transient cerebral ischemia and oxygen-glucose deprivation (OGD) significantly alter the expression profiles of circRNAs in neurons (10-12), but the specific regulatory mechanism by which this occurs has yet to be identified. A previous study has shown that microRNA could interact with circRNAs and directly affect the stability of messenger RNA and the expression of their encoded downstream proteins (13). There is increasing evidence that miRNAs, such as miR-125a-5p, miR-125b-5p, and miR-143-3p, play important roles in the process of stroke (14). Further, research has shown that miR-200a-3p plays a critical role in ischemic stroke modulated by long non-coding RNAs (15); however, it is unclear whether miR-200a-3 interacts with circsRNAs to affect the process of stroke.
As a member of the natriuretic peptide family, corin has been presumed to be epigenetically regulated (16); thus, a better understanding of the epigenetic control mechanism for corin will help clarify its role in the development of common cardiovascular and cerebrovascular diseases and lead to the identification of new therapeutic targets. We sought to investigate the effects of corin on OGD-induced SHSY5Y cells and to determine the underlying mechanisms. We present the following article in accordance with the MDAR reporting checklist (available at https://atm.amegroups.com/article/view/10.21037/atm-22-4943/rc).
Methods
Cell cultures and OGD
We purchased the SHSY5Y cell line from the Cell Bank of the Chinese Academy of Sciences (Shanghai, China). After resuscitation, the cells were cultured in Eagle’s minimum essential medium/F12+ 10% fetal bovine serum medium (Gibco, California, USA) at 37 ℃ under 5% carbon dioxide (CO2) in an incubator. When 90% cell confluence was reached, the cells were passaged at a 1:3 ratio The cells were then added to 96-well plates (density =2×105) for OGD treatment and cultured in sugar-free medium under hypoxic conditions (94% nitrogen, 5% CO2, and 1% oxygen at 37 ℃ for 2 h, 4 h, and 6 h), after which they were cultured in normal medium under normoxia for 24 h.
Cell transfection
The small-interfering RNAs (siRNAs) specific for different sequences of corin mRNA, si-negative control (NC), overexpressed (OV)-circRNA, OV-NC, and a miRNA mimic were designed by Shanghai GenePharma Co., Ltd (see Table 1). SHSY5Y cells were added to a 96-well plate (density =2×105/well), transfected with constructs in the presence of riboFECTCP transfection reagent (RiBoBio, Guangzhou, China), and cultured at 37 ℃ with 5% CO2. Before OGD treatment, the transfected cells were incubated for 24 h. Subsequently, transfection efficiency was measured using Real time-quantitative polymerase chain reaction (RT-qPCR).
Table 1
Name | Sequence |
---|---|
siRNA | 5'-GCAGUGUAAUGGCUACAAUTT-3' |
si-NC | 5'-UAGCACCAUUUGAAAUCAGUGUU-3' |
OV-CircRNA | 5'-GAACTCGAGATTGGCGTCCAACATGGAC-3' |
OV-NC | 5'-GTCCATGTTGGACGCCAATCTCGAGTTC-3' |
miRNA mimic | 5'-UAACACUGUCUGGUAACGAUGU-3' |
NC, negative control; OV, over expression.
Cell viability assays
SHSY5Y cell proliferation was detected using Cell Counting Kit-8 (CCK-8; Beyotime, Shanghai, China). In brief, the SHSY5Y cells were seeded in a 96-well plate (density =2×104/well) and transfected for 24 h. Next, 10 µL of the CCK-8 with solution was added to each well, and the cells were then incubated for 2 h incubation at 37 ℃. Finally, absorbance was calculated at 450 nm.
Flow cytometry analysis
The cytoflex flow cytometer (Beckman Coulter, Inc., California, USA) and Annexin V-FITC Apoptosis Detection Kit (Sigma-Aldrich, Missouri, USA) were used for apoptosis measurement. In brief, the cells (approximately 2×105) were centrifuged at 1,000 ×g for 5 min. After which, the supernatant was removed, and the cells were re-suspended in 500 mL of the conjugation solution. Next, annexin V-FITC and propidium iodide were add by sequential.
Hochest 33258 staining
After the medium was removed, the cells were fixed in paraformaldehyde (4%) for 20 mins. Next, after the fixative was removed, the cells were washed with phosphate buffered solution (PBS) 3 times, and incubated with 0.5 mL of Hochest33258 staining solution for 5 min. After re-washing with PBS, a fluorescence-quenching sealing solution was added. The sample was then covered with a cover slip and observed under a fluorescence microscope.
RT-qPCR analysis
The cytoplasmic and nuclear RNAs were extracted using an RNA purification kit (Norgen BioTek, Thorold, Canada). Reverse transcription was performed using the EasyScript® One-Step gDNA Removal and cDNA Synthesis SuperMix (TransGen Biotech, Beijing, China), followed by amplification using the TransStart® Top Green qPCR SuperMix (TransGen Biotech) and the CFX96 Touch RT-qPCR Detection System (Bio-Rad, California, USA). The assays were repeated 3 times using the cognate specific primer sequences for hsa_circ_0012397, hsa_circ_0002995, hsa-miR-3163, hsa-miR-200a-3p, hsa-miR-141-3p, corin, and U6 (see Table 2). The relative expression levels of the above-mentioned miRNAs were normalized and calculated using the 2−ΔΔ Ct method.
Table 2
Primer name | Primer sequence |
---|---|
hsa_circ_0012397 F | 5'-TCATGGCTGCAATGGAGAT-3' |
hsa_circ_0012397 R | 5'-GGGTCTGTGCAGGTGAAGAT-3' |
hsa_circ_0002995 F | 5'-CCATGAACAACCTGGAAA-3' |
hsa_circ_0002995 R | 5'-GTCCTGGAGTAATGATGG-3' |
hsa-miR-3163 F | 5'-GCGGCCGCCATTCTCACACGTGCCTAAG-3' |
hsa-miR-3163 R | 5'-TCTAGATGAT TACCCAACCTACTGCT-3' |
hsa-miR-200a-3p R | 5'-AACACTGTCTGGTAACGATGTCGT-3' |
hsa-miR-200a-3p F | 5'-ACGACATCGTTACCAGACAGTGTT-3' |
hsa-miR-141-3p R | 5'-GGTCCTAACACTGTCTGGTAAAGTGG-3' |
hsa-miR-141-3p F | 5'-CCAGTGCAGGGTCCGAGGT-3' |
Corin F | 5'-TGCCCAAGCGGAAGTGAG-3' |
Corin R | 5'-GACGGATGGTCCAGGTTGTTT-3' |
U6 F | 5'-CTCGCTTCGGCAGCACA-3' |
U6 R | 5'-AACGCTTCACGAATTTGCGT-3' |
RNase R assays
After the RNAs of the SHSY5Y cells were isolated using the RNAeasy system (RiboMinus Kit, Invitrogen, California, USA) (17). A denatured sample was first heated to 70 ℃ and then cooled to 40 ℃, and 1.7 µL of RNase R buffer was then added and whisked for 1 h at 40 ℃. In 3 wells, RT-qPCR was performed to evaluate the human mRNA expression levels of hsa_circ_0012397 and Fas Associated Factor 1 (FAF1).
Dual-luciferase reporter assays
Website tools, such as miRDB (http://mirdb.org/), TargetScan 7.2 (https://www.targetscan.org/vert_80/), and starBase 3.0 (https://www.starbase.info/index.html), were used to predict the potential binding sites of CORIN and hsa_circ_0012397 in miR-200a-5p. The pGL3 vector (Promega, Wisconsin, USA) was applied to clone the target DNA sequences of wild-type and mutant hsa_circ_0012397, and wild-type and mutant CORIN 3’-UTR, miRNA mimics, and miRNA NC, respectively. These constructs were used to validate the interactions between hsa_circ_0012397 and miR-200a-5p, miR-200a-5×p, and CORIN. We used a dual-luciferase reporter assay system (Promega) to measure fluorescence intensity after adding the SHSY5Y cells to 96-well plates and co-transfecting them with the above plasmids for 48 h.
Western blotting analysis
RIPA Lysis Buffer (Applygen Technologies Inc., Beijing, China) was used to extract the total proteins from the SHSY5Y cells. A BCA kit (MultiSciences, Hangzhou, China) was used to measure the protein concentrations. Sodium dodecyl sulfate-polyacrylamide gel electrophoresis) (15% or 8%, MultiSciences) was used for target protein separation, and the polyvinylidene fluoride membranes (Millipore, Darmstadt, Germany) were then transferred and blocked with 5% skimmed milk powder at 25 ℃ for 90 min. The membranes were incubated overnight at 4 ℃ with the following primary antibodies: anti-Bad (ab32445, 1:1,000), anti-Bcl-2 (ab182858, 1:2,000), anti-Cleaved caspase-3 (ab2302, 1:400), anti-extracellular regulated protein kinase (ERK) (ab184699, 1:8,000), anti-phospho-ERK (ab201015, 1:1,000) (Abcam, Cambridge, UK), anti-protein 38 (p38) (#9212, 1:1,000, CST), anti-phospho-p38 (#9216, 1:2,000, CST), and glyceraldehyde-3-phosphate dehydrogenase (ab8245, 1:8,000, Abcam). The membranes were then incubated with horse radish peroxidase-conjugated secondary antibodies (1:1,000, MultiSciences) at 25 ℃ for 90 min. Next, the bands were visualized.
Statistical analysis
All the experiments were repeated 3 times. The data were presented as the mean ± standard deviation (SD). Differences in relative expression levels of cytoplasmic and nuclear hsa_circ_0012397 (or FAF1) (or RNase R– and RNase R+) were evaluated using the un-paired 2-sided t-test or a 1-way analysis of variance (ANOVA) with Tukey multiple comparisons. A P value <0.05 indicated a statistically significant difference.
Results
The protein expression of corin was significantly downregulated in the OGD-induced stroke model
Recently, corin has been reported to be associated with stroke (6,9); however, the role of corin expression in stroke, particularly ischemic stroke, is unclear. In the present study, SHSY5Y cells deprived of glucose and oxygen were used to establish a stroke model to investigate the role of corin. As Figure 1A shows, the longer the duration of OGD, the fewer SHSY5Y cells survived as indicated by the lower optical density (OD) value detected by the Cell Counting Kit-8 (CCK-8) assays. Conversely, the number of apoptotic cells was significantly increased (see Figure 1B). Further, western blotting also revealed that the expression of corin protein was significantly downregulated with the prolongation of OGD (see Figure 1C,1D). These findings are consistent with the decrease in serum-soluble corin described above.
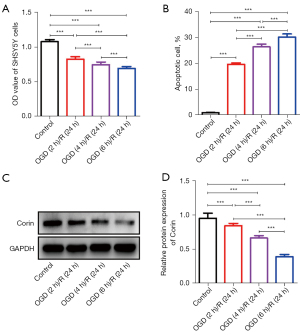
Corin protects cell survival by inhibiting apoptosis-related proteins and ERK/p38 under OGD
To further examine the role of corin in stroke, we explored the effects of the overexpression and silencing of corin on OGD-induced SHSY5Y cells. We found that the number of surviving cells increased and that of apoptotic cells decreased significantly when corin was overexpressed. Conversely, the number of surviving cells decreased and that of apoptotic cells significantly increased when corin was silenced (see Figure 2A,2B). Further, similar results were observed in Hoechst 33258-stained cells, and large numbers of apoptotic cells were observed in the OGD and corin-siRNA + OGD groups, accompanied by pyknosis of the nucleus, deep staining, or fragmented deep staining. However, apoptotic cells were not detected in the control and corin-overexpressed + OGD groups (see Figure 2C).
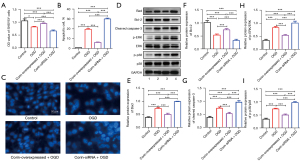
We also analyzed the expression levels of apoptosis-involved proteins in each group (see Figure 2D-2I), and found that the expression levels of Bad, cleaved Caspase-3, p-ERK/ERK, and p-p38/p-38 proteins were significantly higher in the OGD and corin-siRNA + OGD groups than the control group (see Figures 2E,2G-2I), and the expression level of Bcl-2 was significantly downregulated (see Figure 2F). The protein expression levels of Bad, cleaved Caspase-3, p-ERK/ERK, and p-p38/p-38 were significantly lower in the corin-overexpressed + OGD group than the OGD and corin-siRNA + OGD groups, while the expression level of Bcl-2 was higher compared to that of the OGD and corin-siRNA + OGD groups (see Figure 2D-2I). Thus, corin appears to protect cells from apoptosis and ERK/p38 activation under OGD.
Corin regulates hsa_circ_0012397/hsa-miR-200a-3p in OGD-induced SHSY5Y cells
To further clarify the regulatory mechanism of corin, we performed a bioinformatics analysis to predict its upstream-binding miRNAs. In total 10 candidate miRNAs were identified from miRDB, TargetScan 7.2, and starBase 3.0 (see Figure 3A). In miRDB, the miRNAs hsa-miR-3163, hsa-miR-200a-3p, and hsa-miR-141-3p had target scores ≥80. The RT-qPCR analysis showed that the temporal expression of hsa-miR-200a-3p decreased with the prolongation of OGD, while the expression of hsa-miR-3163 and hsa-miR-141-3p did not change significantly (see Figure 3B-3D). Next, we analyzed the potential circRNAs regulating hsa-mir-200a-3p, and identified hsa_circ_0012397 and hsa_circ_0002995 from the Human circRNA database. However, only the former was considered a regulator of hsa-mir-200a-3p in this model, as the expression of the latter did not change significantly, while the former was significantly downregulated with the prolongation of OGD (see Figure 3E,3F).
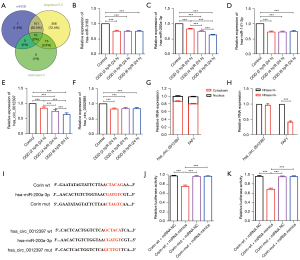
We also found that hsa_circ_0012397 and its target gene FAF1 were mainly expressed in the cytoplasm and nucleus (see Figure 3G). Additionally, RNase R assays confirmed the circularity of hsa_circ_0012397. As Figure 3H shows, the expression of hsa_circ_0012397 was unaffected by RNase R+, while the expression of FAF1 was significantly downregulated by RNase R+, suggesting that hsa_circ_0012397 is indeed a circRNA.
We then used dual-luciferase reporter assays to explore whether there was a direct regulatory relationship between corin expression and hsa-miR-200a-3p, hsa-miR-200a-3p, and hsa_circ_0012397 (see Figure 3I). As Figure 3J shows, the relative luciferase activity of corin-wt + miRNA mimics was significantly lower compared to that of corin-wt + miRNA NC, but there were no significant difference in the relative luciferase activities between corin-mut + miRNA mimics and corin-mut + miRNA NC. Similar results were observed for circRNA-wt + miRNA mimics and CircRNA-wt + miRNA NC, CircRNA-mut + miRNA mimics, and CircRNA-mut + miRNA NC (see Figure 3K). Thus, corin expression in the OGD-induced SHSY5Y cells was regulated by hsa_circ_0012397/hsa-miR-200a-3p.
Corin is regulated by hsa_circ_0012397/hsa-miR-200a-3p
To confirm the function of the hsa_circ_0012397/hsa-miR-200a-3p/corin pathway, we constructed different recombinant plasmids that were used to transfect SHSY5Y cells to find the effect of OGD. As Figure 4A shows, the OD values of the control, OV-CircRNA, miRNA inhibitor, and OV-CircRNA + miRNA mimic groups were significantly higher than those of the model group, suggesting that the numbers of viable cells in these groups were significantly higher than that of the model group. Similarly, the numbers of apoptotic cells in the control, OV-CircRNA, miRNA inhibitor, OV-CircRNA + miRNA mimics, and OV-CircRNA + miRNA mimics + corin-siRNA groups were significantly lower than those of the model group, but those of the OV-CircRNA + miRNA mimics and OV-CircRNA + miRNA mimics + corin-siRNA group were significantly higher than that of the control, OV-CircRNA, or miRNA inhibitor groups (see Figure 4B).
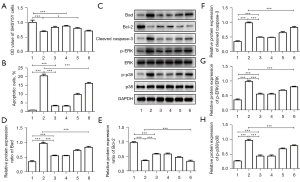
We further analyzed the expression levels of relative proteins. As shown in Figure 4C-4H, the protein expression level of Bad in the model group was significantly higher than those of the other groups, while those of the OV-CircRNA + miRNA mimics or OV-CircRNA + miRNA mimics + corin-siRNA groups were significantly higher than those of the control, OV-CircRNA, or miRNA inhibitor groups (Figure 4C,4D). The protein expression levels of cleaved Caspase-3, p-ERK, and p-p38 in each group were similar to those of Bad (see Figure 4F-4H). However, the protein expression level of Bcl-2 in each group differed to that of Bad, cleaved caspase-3, p-ERK, or p-p38. The level of Bcl-2 protein expression of the model group was lower than those of the control, OV-CircRNA, miRNA inhibitor, or OV-CircRNA + miRNA mimics groups, and those of the OV-CircRNA and miRNA inhibitor groups were significantly higher than those of the OV-CircRNA + miRNA mimics or OV-CircRNA + miRNA mimics + corin-siRNA groups (see Figure 4E). Thus, corin is regulated by hsa_circ_0012397/hsa-miR-200a-3p, such that OGD-induced SHSY5Y cells undergo apoptosis.
Discussion
Ischemic stroke is a major disease associated with the highest rates of disability and mortality worldwide (18). In the minutes after stroke, irreversible tissue damage and subsequent cell death occur (18). Notably, the incidence of ischemic stroke in young people has increased since the 1980s (3). Mechanical thrombectomy within 6 or 24 h after the onset of ischemic stroke is beneficial to the recovery of patients (19); however, it is critical to reduce the necrosis or apoptosis of nerve cells within this time to the greatest extent possible. A previous study has shown that numerous miRNAs play important roles in the regulation of ischemic stroke, including certain non-coding RNAs and circRNAs (20). We predicted and verified the regulatory mechanism of corin, whose serum concentration is significantly decreased in stroke patients. We showed that corin was regulated by has_circ_0012397/hsa-mir-200a-3p during ischemia and hypoxia, which in turn regulated apoptosis-related proteins, such as Bad and cleaved caspase-3, and MAPK signaling proteins (p-ERK and p-38), thereby inhibiting neuronal apoptosis in OGD-induced SHSY5Y cells. Further, we found that corin was regulated by circ-0012397/miR-200a-3p in the SHSY5Y cells subjected to OGD.
Corin is a serine protease selectively anchored to the heart membrane. Its essential activity is to cleave and activate pro-atrial natriuretic peptide and pro-B-type natriuretic peptide. Thus, corin plays key roles in maintaining saline balance and regulating heart function and blood pressure (21,22). Similar to other transmembrane proteases, cardiac corin enters the circulation when it is released by apoptotic cells. A significant decrease in the corin levels in the heart and blood has been reported in patients with heart failure in clinical and experimental settings, and the extent of the decrease is related to the severity of the cardiac dysfunction (23). However, numerous studies have shown that the serum levels of corin significantly change in the presence of diseases, such as obesity (24), hypertension (25), hyperlipidemia (26), preeclampsia (27), diabetic nephropathy (28), and stroke (6,9). Because of the special location of corin, it is used as a biomarker for the diagnosis of cardiovascular disease (29). The corin protein is mainly expressed in heart tissue and enters the circulatory system after shedding; however, a previous study has detected the expression of its gene in dopaminergic neural progenitor cells (30). Moreover, striatal dopaminergic neurons are potential targets for the treatment of ischemic stroke associated with Glial cell line-derived neurotrophic factor (GDNF) (31). Thus, the control of protein expression of corin in dopamine nerves and the mechanism require further research.
Corin has been reported to have anti-inflammatory role in natriuretic peptides treating Congestive heart failure (32). IL-1β and TNF-α could increase the level of urinary corin in patients with primary proteinuric kidney diseases (33). Recent studies have reported that corin participates in regulating several physiological signal transduction pathways, such as the MAPK, PI3K/Akt, and NF-κB pathways (4,5,28). These signaling pathways are closely associated with apoptosis; for example, the activation of the ERK and the p38 pathways phosphorylates p53 to promote the cell death cascade. These findings suggest that corin plays a regulatory role in apoptosis, and corin activates the PI3K/Akt and NF-κB signaling pathways to inhibit hydrogen peroxide-induced apoptosis (34). PI3K/Akt and NF-κB have also been reported to regulate neuroinflammation and oxidative stress (35). Thus, the therapeutic effect of corin may be due to its regulation of neuroinflammation and oxidative stress through PI3K/Akt and NF-κB pathway.
Apoptosis is a pathological or physiological process initiated after the occurrence of ischemic stroke; thus, corin may regulate apoptosis after ischemic stroke. We showed that the expression of corin in OGD-induced SHSY5Y cells was significantly decreased, while the number of apoptotic cells was significantly increased. We also found that corin regulated the expression of apoptosis-related proteins, such as Bad, cleaved caspase-3, and Bcl-2, which suggests that the regulation of corin expression may contribute to the prognosis of ischemic stroke patients. Corin exerts protective role in OGD treated cells. Thus, the low corin level might indicate poor prognosis. Promoting the expression of corin in the brain could be a potential method in treating stroke.
Numerous miRNAs, non-coding RNAs, and circRNAs are involved in the process of ischemic stroke (20). Thus, we conducted a bioinformatics analysis of potential miRNAs regulating the expression of corin and identified 3 candidate miRNAs (i.e., hsa-miR-3163, hsa-miR-200a-3p, and hsa-miR-141-3p). However, further verification showed that only hsa-miR-200a-3p was associated with corin expression. In this model, the expression levels of hsa-miR-3163 and hsa-miR-141-3p were not absolutely associated with corin expression, which may be related to different experimental cells or the fact that they require stimulation in vivo. This issue requires further research.
Evidence indicates that circRNAs can be used as competitive endogenous RNAs to adsorb miRNAs and regulate gene expression by binding to proteins (17,36). Thus, we also analyzed potential circRNAs that adsorb hsa-mir-200a-3p to regulate the protein expression of corin, such as hsa_circ_0012397 and hsa_circ_0002995. Our subsequent experiments revealed that hsa_circ_0012397/hsa-miR-200a-3p regulated the differential expression of corin when overexpressed or silenced. Further, the corin-mediated regulation of the expression of apoptosis-related proteins, including Bad, cleaved caspase-3, and Bcl-2, resulted in changes in OGD-induced SHSY5Y cells.
There were some limitations of this study. Although we confirmed corin was regulated by hsa_circ_0012397/hsa-mir-200a-3p in SHSY5Y cells, there was no animal models were used in this study. To establish the role of corin in ischemic stroke, further studies of primary neurons in animal models and clinical studies need to be conducted. The expression profiles of hsa_circ_0012397 and hsa-mir-200a-3p should also been detected in the in vivo experiments.
Conclusions
We showed that, in SHSY5Y cells, corin was regulated by hsa_circ_0012397/hsa-miR-200a-3p, which in turn regulated the expression of apoptosis-related proteins, such as Bax, cleaved caspase-3, and Bcl-2. Additionally, corin reduced apoptosis in OGD-induced SHSY5Y cells. Our findings provide further insights into the role of corin in ischemic stroke and its potential as a potential therapeutic target.
Acknowledgments
Funding: This work was supported by the Huzhou Public Welfare Applied Research Project (No. 2017GYB02).
Footnote
Reporting Checklist: The authors have completed the MDAR reporting checklist. Available at https://atm.amegroups.com/article/view/10.21037/atm-22-4943/rc
Data Sharing Statement: Available at https://atm.amegroups.com/article/view/10.21037/atm-22-4943/dss
Conflicts of Interest: All authors have completed the ICMJE uniform disclosure form (available at https://atm.amegroups.com/article/view/10.21037/atm-22-4943/coif). The authors have no conflicts of interest to declare.
Ethical Statement: The authors are accountable for all aspects of the work in ensuring that questions related to the accuracy or integrity of any part of the work are appropriately investigated and resolved.
Open Access Statement: This is an Open Access article distributed in accordance with the Creative Commons Attribution-NonCommercial-NoDerivs 4.0 International License (CC BY-NC-ND 4.0), which permits the non-commercial replication and distribution of the article with the strict proviso that no changes or edits are made and the original work is properly cited (including links to both the formal publication through the relevant DOI and the license). See: https://creativecommons.org/licenses/by-nc-nd/4.0/.
References
- Maida CD, Norrito RL, Daidone M, et al. Neuroinflammatory Mechanisms in Ischemic Stroke: Focus on Cardioembolic Stroke, Background, and Therapeutic Approaches. Int J Mol Sci 2020;21:6454. [Crossref] [PubMed]
- Thirugnanachandran T, Beare R, Mitchell M, et al. Anterior Cerebral Artery Stroke: Role of Collateral Systems on Infarct Topography. Stroke 2021;52:2930-8. [Crossref] [PubMed]
- Putaala J. Ischemic Stroke in Young Adults. Continuum (Minneap Minn) 2020;26:386-414. [Crossref] [PubMed]
- Datta A, Sarmah D, Mounica L, et al. Cell Death Pathways in Ischemic Stroke and Targeted Pharmacotherapy. Transl Stroke Res 2020;11:1185-202. [Crossref] [PubMed]
- Yue J, López JM. Understanding MAPK Signaling Pathways in Apoptosis. Int J Mol Sci 2020;21:2346. [Crossref] [PubMed]
- Dong N, Niu Y, Chen Y, et al. Function and regulation of corin in physiology and disease. Biochem Soc Trans 2020;48:1905-16. [Crossref] [PubMed]
- Sullivan RD, Houng AK, Gladysheva IP, et al. Corin Overexpression Reduces Myocardial Infarct Size and Modulates Cardiomyocyte Apoptotic Cell Death. Int J Mol Sci 2020;21:3456. [Crossref] [PubMed]
- Hu W, Chen S, Song Y, et al. Serum Soluble Corin Deficiency Predicts Major Disability within 3 Months after Acute Stroke. PLoS One 2016;11:e0163731. [Crossref] [PubMed]
- Shen X, Dong N, Xu Y, et al. Analyzing Corin-BNP-NEP Protein Pathway Revealing Differential Mechanisms in AF-Related Ischemic Stroke and No AF-Related Ischemic Stroke. Front Aging Neurosci 2022;14:863489. [Crossref] [PubMed]
- Zuo L, Li C, Zu J, et al. Circular RNA FUNDC1 improves prediction of stroke associated infection in acute ischemic stroke patients with high risk. Biosci Rep 2020;40:BSR20200902. [Crossref] [PubMed]
- Chen W, Wang H, Feng J, et al. Overexpression of circRNA circUCK2 Attenuates Cell Apoptosis in Cerebral Ischemia-Reperfusion Injury via miR-125b-5p/GDF11 Signaling. Mol Ther Nucleic Acids 2020;22:673-83. [Crossref] [PubMed]
- Yang L, Han B, Zhang Z, et al. Extracellular Vesicle-Mediated Delivery of Circular RNA SCMH1 Promotes Functional Recovery in Rodent and Nonhuman Primate Ischemic Stroke Models. Circulation 2020;142:556-74. [Crossref] [PubMed]
- Sinha T, Panigrahi C, Das D, et al. Circular RNA translation, a path to hidden proteome. Wiley Interdiscip Rev RNA 2022;13:e1685. [Crossref] [PubMed]
- Tiedt S, Prestel M, Malik R, et al. RNA-Seq Identifies Circulating miR-125a-5p, miR-125b-5p, and miR-143-3p as Potential Biomarkers for Acute Ischemic Stroke. Circ Res 2017;121:970-80. [Crossref] [PubMed]
- Yang H, Wang H, Zhang X, et al. Upregulated LINC00319 aggravates neuronal injury induced by oxygen-glucose deprivation via modulating miR-200a-3p. Exp Ther Med 2021;22:844. [Crossref] [PubMed]
- Rubattu S, Stanzione R, Cotugno M, et al. Epigenetic control of natriuretic peptides: implications for health and disease. Cell Mol Life Sci 2020;77:5121-30. [Crossref] [PubMed]
- Jeck WR, Sorrentino JA, Wang K, et al. Circular RNAs are abundant, conserved, and associated with ALU repeats. RNA 2013;19:141-57. [Crossref] [PubMed]
- Herpich F, Rincon F. Management of Acute Ischemic Stroke. Crit Care Med 2020;48:1654-63. [Crossref] [PubMed]
- Silva GS, Nogueira RG. Endovascular Treatment of Acute Ischemic Stroke. Continuum (Minneap Minn) 2020;26:310-31. [Crossref] [PubMed]
- Qian Y, Chopp M, Chen J. Emerging role of microRNAs in ischemic stroke with comorbidities. Exp Neurol 2020;331:113382. [Crossref] [PubMed]
- Parcha V, Irvin MR, Lange LA, et al. Corin Missense Variants, Blood Pressure, and Hypertension in 11 322 Black Individuals: Insights From REGARDS and the Jackson Heart Study. J Am Heart Assoc 2022;11:e025582. [Crossref] [PubMed]
- Zhang X, Gu X, Zhang Y, et al. Corin: A Key Mediator in Sodium Homeostasis, Vascular Remodeling, and Heart Failure. Biology (Basel) 2022;11:717. [Crossref] [PubMed]
- Wang D, Gladysheva IP, Sullivan RD, et al. Increases in plasma corin levels following experimental myocardial infarction reflect the severity of ischemic injury. PLoS One 2018;13:e0202571. [Crossref] [PubMed]
- Zhang H, Mo X, Zhou Z, et al. Smoking modifies the effect of two independent SNPs rs5063 and rs198358 of NPPA on central obesity in the Chinese Han population. J Genet 2018;97:987-94. [Crossref] [PubMed]
- Zou T, Yao S, Du MF, et al. Associations of corin genetic polymorphisms with salt sensitivity, blood pressure changes, and hypertension incidence in Chinese adults. J Clin Hypertens (Greenwich) 2021;23:2115-23. [Crossref] [PubMed]
- Liu Y, Peng H, Zhang Q, et al. Association between serum soluble corin and hyperglycaemia: a cross-sectional study among Chinese adults. BMJ Open 2015;5:e009085. [Crossref] [PubMed]
- Degrelle SA, Chissey A, Stepanian A, et al. Placental Overexpression of Soluble CORIN in Preeclampsia. Am J Pathol 2020;190:970-6. [Crossref] [PubMed]
- Xue M, Shi Y, Pang A, et al. Corin plays a protective role via upregulating MAPK and downregulating eNOS in diabetic nephropathy endothelial dysfunction. FASEB J 2020;34:95-106. [Crossref] [PubMed]
- Yu R, Han X, Zhang X, et al. Circulating soluble corin as a potential biomarker for cardiovascular diseases: A translational review. Clin Chim Acta 2018;485:106-12. [Crossref] [PubMed]
- He M, Zhang H, Li Y, et al. Direct and selective lineage conversion of human fibroblasts to dopaminergic precursors. Neurosci Lett 2019;699:16-23. [Crossref] [PubMed]
- Beker MÇ, Beker M, Çağlayan AB, et al. Striatal dopaminergic neurons as a potential target for GDNF based ischemic stroke therapy. Turk J Med Sci 2021; Epub ahead of print. [Crossref] [PubMed]
- Khoury EE, Kinaneh S, Aronson D, et al. Natriuretic peptides system in the pulmonary tissue of rats with heart failure: potential involvement in lung edema and inflammation. Oncotarget 2018;9:21715-30. [Crossref] [PubMed]
- Sun C, Shen L, Sha W, et al. IL-1β increases urinary corin in patients with primary proteinuric kidney diseases and in 293 cells. Exp Ther Med 2018;15:487-93. [PubMed]
- Li Y, Xia J, Jiang N, et al. Corin protects H2O2-induced apoptosis through PI3K/AKT and NF-κB pathway in cardiomyocytes. Biomed Pharmacother 2018;97:594-9. [Crossref] [PubMed]
- Hassan SSU, Samanta S, Dash R, et al. The neuroprotective effects of fisetin, a natural flavonoid in neurodegenerative diseases: Focus on the role of oxidative stress. Front Pharmacol 2022;13:1015835. [Crossref] [PubMed]
- Du WW, Fang L, Yang W, et al. Induction of tumor apoptosis through a circular RNA enhancing Foxo3 activity. Cell Death Differ 2017;24:357-70. [Crossref] [PubMed]
(English Language Editor: L. Huleatt)