Turning ‘Cold’ tumors ‘Hot’: immunotherapies in sarcoma
Introduction
A number of immunotherapeutic agents have shown improved outcomes in advanced cancers. Anti PD-1 (programmed death) and anti-PD-L1 (programmed death ligand), as well as anti-CTLA-4 (cytotoxic T-lymphocyte-associated protein 4), have already led to FDA approvals for melanoma, lung cancer, urothelial carcinomas, renal cell carcinoma, Hodgkin’s lymphoma, GI cancers and head and neck cancers (1). Microsatellite instability (MSI) high or deficient mismatch repair has also emerged as a biomarker leading to approval of pembrolizumab, an anti -PD1 drug treatment (2).
However, targeted immunotherapy for sarcomas has proved challenging. Sarcoma is a rare malignancy with many sub-types that comprises approximately 1% of all malignancies (3). Diagnosis of advanced soft tissue sarcoma carries a median overall survival of one year, with only 10% of patients surviving to five years after diagnosis (3). Surgical resection remains the mainstay of treatment, with radiation therapy and chemotherapy playing roles in certain neo-adjuvant settings; however, the need for re-invigorated treatment approaches to improve patient outcomes remains. Lack of consistent response to immunotherapies has been attributed to the number and diversity of tumor subtypes. Herein we explore the challenges and possible strategies to bypassing intrinsic tumor characteristics to improve efficacy of immunotherapies in sarcoma.
Immunotherapy as a monotherapy in sarcoma
PD-L1 expression has been well correlated with the presence of tumor infiltrating lymphocytes in several soft tissue sarcoma subtypes and its expression has been reported in 12–65% of sarcomas (4,5). Together with this information and given the encouraging results in other cancer types, immunotherapy as a monotherapy was explored in the SARC028 trial, a non-randomized multicenter phase II trial in which patients received the anti-PD-1 antibody pembrolizumab at a dose of 200 mg IV every 3 weeks in the setting of metastatic or surgically unresectable soft tissue and bone sarcomas (6). Multiple subtypes were selected based on prevalence, with a primary endpoint of objective response and secondary endpoints consisted of overall and progression-free survival. It was hypothesized that pembrolizumab would show clinical benefit with partial response or better. Of the 40-patient soft tissue sarcoma cohort, 18% reached clinically meaningful response. Median progression-free survival was 18 weeks. One patient with undifferentiated pleomorphic sarcoma achieved a complete response, indicating potential for profound effects of immunotherapy in the right clinical context. Within the trial, only 11% of patients experienced serious events and none were fatal (6).
A smaller study used ipilimumab as a single agent at a dose of 3 mg/kg every 3 weeks for 3 cycles in the treatment of synovial sarcomas. In 6 patients treated the median overall survival was 8.7 months (0.8–19.7 months). The study was closed prematurely due to all patients demonstrating radiographic or clinical evidence of disease progression after three cycles of therapy and no objective responses (7).
D’Angelo et al. conducted a phase II trial in which one of the treatment arm used nivolumab as monotherapy in the setting of metastatic sarcoma, enrolling 43 patients with a primary endpoint of objective response (8). Confirmed responses in the nivolumab group were 5%; thus, investigators concluded that nivolumab did not warrant further study as a monotherapy agent.
An additional multi-institutional retrospective review performed by Monga et al. included 88 soft tissue sarcoma patients who received immunotherapy as monotherapy either on trial or as off label (9). Study participants on average had already received a median of two prior therapies. In those patients receiving pembrolizumab alone, 23% of patients experienced clinical benefit as defined by overall or partial response; progression-free survival was 4.1 months. Rate of adverse effects requiring discontinuation of immunotherapy was 16.7% (9). In a study by Quiroga et al, activity of PD-1 inhibitors was explored as monotherapy in sarcomas including bone sarcomas in which 56 patients received pembrolizumab versus nivolumab monotherapy (10). Overall response rate was 11.5%, comparable to that seen in the prior studies mentioned above. Median progression free survival was 11.3 weeks (10).
PD-L1 expression on tumor cells has predicted responses to immunotherapy in multiple tumor types including non-small cell lung cancer (NSCLC) and esophageal cancer (11,12). The SARC 28 trial showed that tumors likely to respond to pembrolizumab had higher expression of PD-L1 receptors on T cells and a higher expression PD-L1 on tumor associated macrophages (13). Looking further into the microenvironment of sarcomas in these studies offers a possible rationale. Petitprez et al. reviewed the histology and genomics of 608 tumors of various sarcoma subtypes (14). Based on gene expression profiles, tumors were subtyped based on sarcoma immune classification (SIC) with notable distinctions in response to immunotherapy. The investigators categorized phenotypes into immune-low or “cold” phenotypes, which had low expression related to immune cells as well as low expression related to vasculature, and immune-high, or “hot” phenotypes, which scored comparably higher or the “hot” phenotypes. Histologically, they correlated with increased densities of CD3+, CD8+, and CD20+ cells in the immune-high phenotypes. A significant difference in survival was noted between SIC immune-high and -low phenotypes, reinforcing the importance of the expression of these immune cells and their microenvironment to patient survival (14). Interestingly, the immunotherapy responsive subgroup was also particularly rich in follicular dendritic cells and B cells. While not completely analogous, a similar effect was seen in a case series involving a particularly rare sarcoma subtype, follicular dendritic cell sarcoma (15). Despite deriving from lymphatic tissue, this neoplasm and other histiocytic and dendritic neoplasms are more pathologically similar to sarcoma. Lee et al. attempted treatment in two patients with ipilimumab and nivolumab on a standard dosing schedule. Both patients demonstrated a sustained complete response lasting more than 6 months, likely due to the fact that this malignancy is comprised almost entirely of phenotypically immunoresponsive cells. In addition to potentially converting a disease with 40% mortality into one with the possibility of complete response, this report underscores the implications of a better understanding of the tumor microenvironment (16). Further studies in these rarer tumor type would be beneficial.
Sarcoma microenvironment
Immunosuppression phenotypes within the microenvironment
In addition to malignant cells, a variety of cell types make up the tumor microenvironment. Chief among them, and a key player in the resistance to immunotherapy efficacy, is the myeloid-derived suppressor cell (MDSC) (17). MDSCs include several immature non-macrophage cells sharing the ability to suppress T-cell function and T-cell activation (18). A precursor of normal myeloid development, terminal differentiation of these cells may result in granulocytes, monocytes, or dendritic cells, and play an essential role in fighting infections. However, characteristics of a cell prior to terminal differentiation results in an immunosuppressive function, an effect utilized in malignancy. If associated with a tumor, most MDSCs that differentiate will become tumor-associated macrophages (TAMs), which also share immunosuppressive tendencies (19). Multiple growth factors classically associated with tumor growth also serve as chemo attractants and inducers for MDSCs. While not all-inclusive, specific examples include VEGF, IL-6, IL-1beta, TNF-alpha, and estrogen (20-24). Once within the microenvironment, MDSCs exert immunosuppressive effects through production of VEGF, Arg1 (leading to depletion of arginine critical to T-cell activation), and ROS (inducing apoptosis of target cells via oxidative stress) (18). Efforts to deplete and differentiate MDSCs have been attempted in an effort to remove the immune inhibition and creating a more favorable microenvironment for immunotherapies to take hold (17). Several studies have attempted to limit MDSCs by interfering with CCR5, CXRC2, and CXCR4, chemokine receptors found to play a crucial role in MDSC presence (25-27). Direct depletion of MDSCs with systemic chemotherapies has been investigated with 5-FU, paclitaxel, docetaxel, cisplatin, and gemcitabine with varying degrees of success. Direct depletion with an anti-CD33 immunotherapy (commonly expressed on MDSCs) was shown to be effective in eliminating MDSCs (28,29). Differentiation with the goal of transitioning to a less immunosuppressive phenotype and proinflammatory phenotype was explored with all-trans retinoic acid (ATRA) and vitamin D, demonstrating successful differentiation in in vivo models (30,31). As the impact of MDSCs as pivotal immune regulators was minimized in these studies, combination therapies with checkpoint inhibitors in multiple oncological subtypes have progressed to clinical trials (NCT00217672, NCT03200847, NCT03161431, NCT02907099, NCT03274804, NCT02922764) (29). A successful phase II clinical trial conducted by Wilky et al. targeted MDSCs in sarcoma using a VEGF tyrosine kinase inhibitor axitinib in addition to pembrolizumab, enrolling 33 patients (32). Progression-free survival was reported at 66% at 3 months, and median follow-up of the study was 14.7 months, comparing favorably to other monotherapy studies.
ATRA plays an important role in the cell growth, differentiation, and apoptosis. Pre-clinical study revealed improved efficacy of chimeric antigen receptor therapy (CAR-T) with the addition of ATRA in the setting of sarcoma potentially due to decreased MDSCs and subsequent decreased phenotype of the tumor (33). Zhou et al. also demonstrated decreased osteosarcoma metastases with the use of ATRA via inhibition of M2 macrophage migration and decreased M2 gene transcription, a TAM previously established as playing a critical role in cancer metastases (34). Devalaraja et al. examined a retinoic acid-sensitive pathway of monocyte differentiation to TAMs as opposed to previously alluded to dendritic cells (found to be associated with immunotherapy responsiveness) (35). The investigators demonstrated that dendritic differentiation was inhibited via ATRA and blocking retinoic acid led to enhancement of T-cell immunity. Drugs blocking retinoic acid production in the sarcoma tumors in combination with immunotherapy should be explored.
TAMs are divided into two subgroups: M1 TAMs are a cytotoxic and proinflammatory phenotype and M2 TAMs are an anti-inflammatory and repair phenotype, more beneficial to the tumor (36). Importantly, TAMs demonstrate plasticity and the ability to move from one phenotype to the other, depending on cytokine and interleukin stimulation (e.g., IFN-γ for M1, IL-6 for M1, IL-10 for M2, TGF-β for M2) (36,37). Once associated with the tumor, elevated macrophages are correlated with worse prognosis in a variety of cancers including sarcomas (38). A study by D’Angelo et al. identified macrophages in 90% of sarcoma subtypes analyzed, including gastrointestinal stromal tumors (GIST) and leiomyosarcoma (4). The Cancer Genome Atlas notes that increased macrophages are also present in undifferentiated pleomorphic sarcoma (UPS) and liposarcoma, among others (39). Van de Rijn et al. reported that a subset of leiomyosarcoma was found to secrete macrophage colony-stimulating-factor leading to the production of TAM, possibly providing an explanation for poor response to immunotherapy (40). Dancsok et al. used a receptor subtype to characterize tissue expression of anti-phagocytic receptors (CD47) and polarization disposition of macrophages (CD68 vs. CD163+) in a series of over 1,200 sarcoma specimens (37). Sarcoma specimens as a whole had a tendency to stain anti-phagocytic with CD163+ M2 macrophages which are anti-inflammatory in function. Additionally, macrophage related immune checkpoint CD47/SIRPα was noted to have high expression in chordoma, angiosarcoma and pleomorphic liposarcoma. Hence drugs targeting CD47 or IDO-1 inhibitors in combination with anti PD-1 immunotherapies which is already showing promise in pre-clinical study (41) would be worth exploring in sarcoma clinical trials.
GIST is a uniquely survivable sarcoma that lends itself to the study of changes precipitated by imatinib in the tumor microenvironment and TAMs. One of the mainstays of GIST treatment is imatinib mesylate, a tyrosine kinase inhibitor that has been shown to improve progression-free survival from 1 to 5 years, by inhibiting an activated KIT receptor tyrosine kinase present in 80% of tumors (42). As expected, initial evaluation of the TAMs presents in GIST exhibited a protumoral phenotype with a predisposition to immunosuppress, repair tissue, and promote angiogenesis. In a murine study, mice with GIST administered imatinib mesylate eventually developed resistance to therapy; however, TAMs were depleted and existing macrophages turned toward an M1 phenotype (43). Tyrosine kinase inhibitors have exhibited consistent tumor microenvironment immunomodulatory effects through a variety of mechanisms and targets (e.g., EGFR, PDGFR, VEGFR) within several different malignancies (44). Examples of postulated mechanisms include c-Kit inhibition leading to depletion of MDSCs with sunitinib, c-fms kinase inhibition leading to TAM decreases with dasatinib, and JAK inhibition leading to decreased regulatory T-cells (Tregs) (44). This highlights the rationale of using anti VEGF TKI such as axitnib in combination with pembrolizumab immunotherapy which was successfully explored in phase 2 clinical trial in soft tissue sarcoma. A significant activity was noted in the ASPS subtype (32).
A mechanism in normal biology critical in preventing autoimmunity, Tregs can maintain immune tolerance via direct induction of cytotoxicity to activated CD8+ and CD4+ T-cells, and downregulation of the ligand stimulators of these same cells (45). Infiltration of Tregs within malignancies has been described and correlated with poor prognosis, including sarcomas (46,47). Presence of Tregs has been correlated with increasing tumor grade and suggests a possible explanation for less than optimal response to immunotherapy in sarcoma (48). Within a tumor, Tregs secrete TGF-β, IL-10, and IL-35, which enhances anti-tumor immunity, decreases antigen presentation of dendritic cells, and inhibits CD8+ T-cell function. Tregs also decrease the functionality of effector T-cells via interleukin secretion, which interferes with metabolism, killing effector T-cells with granzymes and perforin. Positive feedback loops lead to mutual expansion of MDSCs and Tregs to reinforce the immunosuppressive nature of the microenvironment (45). MDSCs are able to induce the development of Tregs via IL-10, IDO, and IFN-γ and Tregs promote the expansion of MDSCs primarily via TGF-β and IL-35 (49-51).
While it is clear the tumor microenvironment plays a vital role in treatment outcomes, the difficulty lies in finding an approach to safely and effectively manipulating it to convert “cold” tumors to “hot”. Drugs targeting MDSCs and Tregs may enhance the effect immunotherapies in sarcomas.
Early returns on strategies for potentiating immunotherapy in sarcoma
Radiation therapy
While wide-local resection remains the mainstay in treatment of localized sarcomas, adjuvant radiation therapy plays a pivotal role, especially in the treatment of high-grade soft tissue sarcomas. Radiation is also given as a palliative treatment of more widespread disease as well as treatment of lesions in unresectable areas. The mechanism of tumor destruction utilizes ionizing radiation to induce tumor DNA damage, creating a downstream effect of apoptosis and cell death (52). Interestingly, radiation may also play an immunomodulatory role via neoantigen exposure, inciting a systemic response to the tumor. A secondary additive effect could also induce an irradiated tumor to shift from an inert “cold” phenotype to an inflamed “hot” phenotype, infiltrated with immunologically active cells that are correlated with improved prognosis (53,54). Radiation therapy appears to cause this effect through secretion of interferons, leading to dendritic cell maturation and ultimately stimulation of both the innate and adaptive immune systems. In vitro analysis of sarcoma specimens following radiation has confirmed the presence of neoantigens unrecognized prior to radiation, as well as the crucial upregulation of MHC-I receptors, possibly due to the depletion of MDSCs in the tumor following radiation, another encouraging alteration of the tumor microenvironment.
The most dramatic clinical effect of radiation therapy and immune activation against a tumor may be the abscopal effect, wherein a clinical reduction in tumor burden is seen following irradiation of a second lesion at a different site (55). In melanoma, one such phase I trial evaluated non-irradiated lesions for possible abscopal response, finding an 18% response rate within the non-irradiated lesions. More importantly, 2-year overall survival of the patient cohort with radiation and ipilimumab was 35% compared to 23% with ipilimumab alone (56). Within soft tissue sarcoma, a case series performed by Callaghan et al. evaluated 5 patients (4 with soft tissue sarcoma) who underwent stereotactic radiation therapy with 21–54 Gy in 3–5 fractions, with the first dose given 1 to 4 weeks after starting pembrolizumab (57). This combination therapy was well tolerated, and high rates of local tumor regression as well as a possible abscopal effect were noted. Of note, one patient was initially diagnosed with undifferentiated pleomorphic sarcoma of the retroperitoneum and developed a peri-vesicular metastasis. Eight months after undergoing radiation therapy to the nephrectomy bed (and with <0.05 Gy to the bladder metastasis) and concurrent immunotherapy, the patient had >50% reduction by volume of the non-irradiated tumors (57).
Given these encouraging possibilities and, albeit limited, demonstration of clinical promise, more investigation is warranted. Clinical trials at several institutions around the world are actively recruiting to further explore different doses and delivery of radiation together with immunotherapy in sarcoma (NCT03463408, NCT03116529, NCT03548428, NCT03338959, NCT03307616, NCT02992912).
Oncolytic viruses
Oncolytic viruses are often recombinantly engineered to select for neoplastic cells (58). As monotherapy, oncolytic viruses are able to exert their tumor destructive potential via a dual-mechanistic approach: viral replication within the tumor host leading to cell lysis, and indirect activation of the immune system toward the tumor via exposure to neoantigens from the lysed cell (59). It is this second, indirect effect that is most likely to be used in combination with immunotherapy to achieve more desirable clinical outcomes.
Kelly et al. reported a phase II trial of combination oncolytic virus and immunotherapy in 20 patients with locally advanced metastatic soft tissue sarcoma (60). Initial treatment of pembrolizumab and intravenous talimogene laherparepvec (TVEC) was followed by intratumoral injections of TVEC. The primary outcome was objective response as determined by RECIST criteria. Overall response rate of the study was 35%, with median duration of response being 56 weeks; progression-free survival was 17.1 weeks. Histological analysis of tumor specimens revealed increasing upregulation of PD-1 receptors following therapy, with 55% of patients turning PD-1 positive with paired tumor samples (60). Tumor-infiltrating lymphocyte (TIL) score was also analyzed, with higher TIL scores after treatment correlating with treatment response. These histological analyses underscore the importance of re-activating the immune microenvironment in these tumors and provide rationale for the encouraging results.
Chemotherapy
Traditional chemotherapy with adjunct immunotherapy to produce a synergistic effect, whether through neoantigen formation or immunomodulatory properties of the chemotherapy itself is a newer area of study. The PEMBROSARC study combined pembrolizumab and metronomic cyclophosphamide in a 15-patient cohort (61). The primary endpoints were non-progression and partial response, with results similar to those in the SARC028 trial (13.3% vs. 18.8%, respectively). Median progression-free survival was 1.4 months. While the sample size of the PEMBROSARC study was small, the comparable rate to immunotherapy alone does not lead to optimism towards this combination of therapy in sarcoma.
Conversely, Pollack et al. reported results of a phase I/II trial that evaluated doxorubicin with pembrolizumab immunotherapy (62). Although the primary outcome of response rate was not met, progression-free survival was significantly prolonged with combined chemo-immunotherapy (8.1 months) versus chemotherapy alone (4.1 months).
One currently recruiting clinical trial is investigating whether immunotherapy as a first-line treatment might provide changes in the microenvironment to allow for a more robust chemotherapeutic response (63). Early results of a phase I/II trial of trabectedin, ipilimumab, and nivolumab as first line treatment in advanced and metastatic soft tissue sarcoma showed some tumor necrosis and an increase in CD8+ killer T cells. Primary objective is safety, with secondary outcomes being overall response rate, progression free survival, and overall survival.
T-cell targeted approach
An alternative approach to alteration of the tumor microenvironment is the introduction of autologous T-cells with enhanced activity against a specific tumor epitope. Instead of systemic modification of the immune system with a receptor antibody such as an anti-PD1, this approach allows direct introduction of a confirmed tumor antigen in an effort to activate the immune system against the neoplasm (64). Currently two main technologies of adoptive T cell immunotherapies are being utilized; in one method chimeric antibody receptor engineered T cell (CAR-T) which focuses on helping the immune cells recognize the tumor antigens and then directing them to locate and kill the target tumor cells. In the second method antigen specific T cells are used to transfect the tumor specific genetically modified T cell receptors (TCR) and help to unblock the tumor immune tolerance (65). CAR-T targeting CD 19 antigen have proven to be very successful in treatment of refractory acute lymphoblastic leukemia/lymphoma, mantle cell lymphoma and large B cell lymphomas and is now FDA approved for their treatment (66-68). However, there are certain challenges with its use in solid tumors involving around penetration within the solid tumor and bringing an effect. TCR therapies on the other hand utilizes the natural mechanism that the T Cells use to recognize cancer antigen hence appear to be more promising in sarcomas.
This TCR concept was evaluated as a surrogate therapy in a clinical trial utilizing NY-ESO-1 SPEAR T cells in 42 patients with synovial sarcoma (69). NY-ESO-1 expression and HLA-A*02 positivity was confirmed prior to therapy, and response was measured according to RECIST criteria. Fifteen patients demonstrated partial or complete response, with 24 demonstrating stable disease at the time of publication. The tumor microenvironment showed heterogeneity between both responders and non-responder. This study demonstrates the potential of a highly activated immune system against sarcoma even without the ability to reset the tumor microenvironment. While limited in terms of HLA subtype and tumor antigen expression, T-cell targeted therapies are likely to remain a key part of the clinical approach to sarcoma.
Combination immunotherapy
The goal of combination immunotherapy is to further activate the immune system with a cascading blockade of critical receptors on cytotoxic cells to bypass the kill-switch mechanisms that lead to overactivation and induce an immune system dampened by the tumor microenvironment. In many cases, this cannot be overcome by a single immunotherapeutic agent.
A trial performed by D’Angelo et al. compared nivolumab (anti PD-1) at 3 mg/kg every 2 weeks to a combination of ipilimumab (1mg/kg every 3 weeks for 4 doses) and nivolumab (3 mg/kg every 2 weeks) in 85 patients, with a primary endpoint of confirmed objective response (8). Median progression-free survival was 1.7 months in the monotherapy arm compared to 4.1 months in the combination arm; overall survival was 10.7 and 14.3 months, respectively. Given the contrast in the study arms, the protocol was amended to allow crossover of 21 eligible patients to the combination therapy arm of the trial. There were 14% grade 3 and 4 treatment related adverse events in the combination arm but the authors concluded that a dose reduction of ipilimumab could alleviate the burden of these adverse effects (8). Independent of dose adjustments, the increased survival following additional immunotherapy by further decreasing tumor immunosuppression reiterates the importance of understanding and overcoming the profound effects identified by the tumor microenvironment.
Another current clinical trial is investigating durvalumab (anti PD-1) and tremelimumab (anti CTLA-4) concurrent therapy in the setting of advanced and metastatic sarcoma (NCT02815995). The study will enroll 62 patients with primary outcomes of progression-free survival, tumor response, complete response, and overall survival. Patients having prior therapy with anti-PD1, anti-PD-L1, and CTLA-4 are excluded, but those having had prior local radiation therapy are eligible. A similar trial is being conducted in which patients will be randomized to a combination of durvalumab and tremelimumab versus doxorubicin as a first-line treatment in advanced or metastatic soft tissue sarcoma (70).
Future directions
Immunotherapy is showing early promise in the treatment of certain common subtypes of soft tissue sarcoma; however, monotherapy responses with anti-PD1 therapies are modest at best. Activity of immunotherapy in rarer sarcoma subtypes remains to be explored. Experiences in the form of early phase clinical trials and case reports will help understand the role of immunotherapy in rarer subtypes. Broadly, sarcomas are considered immunologically “cold;” however, combination approaches with traditional treatments such as radiation, chemotherapy and tyrosine kinase inhibitors have contributed to turning these cold tumors “hot” (Figure 1). The mechanisms of immunotherapy response are being uncovered specially the role of B cells in the immune responsive subtypes. The toxicities with these combinations have been manageable overall. Novel immunotherapy approaches such as adoptive T cell and chimeric antigen receptor T cell therapies have a role in sarcoma management as target antigens such as NY-ESO have been identified in certain subtypes of sarcomas (synovial sarcoma and myxoid round cell liposarcoma).
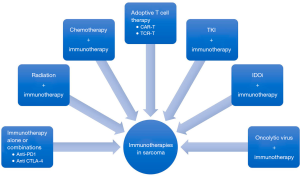
Photo or sonodynamic therapy has been explored in multiple cancer types but remains to be explored in sarcomas. Similar to the approach with using oncolytic viruses, photodynamic therapy may help expose more neoantigens and may improve responses to immunotherapy (71). In addition to tyrosine kinase inhibitors epigenetic therapies such as hypomethylating agents and pharmacological ascorbate may help potentiate the response to immunotherapies as shown in multiple clinical and pre-clinical studies in other tumor types (72-75).
Acknowledgments
Funding: This work is supported by Cancer Center Support Grant National Cancer Institute 3P30CA086862-14S1.
Footnote
Provenance and Peer Review: This article was commissioned by the Guest Editors (Umang Swami and Mohammed M. Milhem) for the series “Cancer Immunotherapy: Recent Advances and Challenges” published in Annals of Translational Medicine. The article has undergone external peer review.
Peer Review File: Available at http://dx.doi.org/10.21037/atm-20-6041
Conflicts of Interest: All authors have completed the ICMJE uniform disclosure form (available at http://dx.doi.org/10.21037/atm-20-6041). The series “Cancer Immunotherapy: Recent Advances and Challenges” was commissioned by the editorial office without any funding or sponsorship. MMM reports research funding from Amgen, Novartis, Merck, Pfizer, ER Squibb & Sons, and Prometheus, honoraria from Blueprint Medicine, Immunocore, Amgen, and Trieza, and serves in a consulting or advisory role for Blueprint Medicine, Amgen, Immunocore, and Trieza. VM serves in a consulting or advisory role for Forma therapeutics and reports research funding and travel expenses from Deciphera and GSK and research funding from Amgen. The authors have no other conflicts of interest to declare.
Ethical Statement: The authors are accountable for all aspects of the work in ensuring that questions related to the accuracy or integrity of any part of the work are appropriately investigated and resolved.
Open Access Statement: This is an Open Access article distributed in accordance with the Creative Commons Attribution-NonCommercial-NoDerivs 4.0 International License (CC BY-NC-ND 4.0), which permits the non-commercial replication and distribution of the article with the strict proviso that no changes or edits are made and the original work is properly cited (including links to both the formal publication through the relevant DOI and the license). See: https://creativecommons.org/licenses/by-nc-nd/4.0/.
References
- Kruger S, Ilmer M, Kobold S, et al. Advances in cancer immunotherapy 2019 - latest trends. J Exp Clin Cancer Res 2019;38:268. [Crossref] [PubMed]
- Le DT, Uram JN, Wang H, et al. PD-1 Blockade in Tumors with Mismatch-Repair Deficiency. N Engl J Med 2015;372:2509-20. [Crossref] [PubMed]
- Demetri GD, Antonia S, Benjamin RS, et al. Soft tissue sarcoma. J Natl Compr Canc Netw 2010;8:630-74. [Crossref] [PubMed]
- D'Angelo SP, Shoushtari AN, Agaram NP, et al. Prevalence of tumor-infiltrating lymphocytes and PD-L1 expression in the soft tissue sarcoma microenvironment. Hum Pathol 2015;46:357-65. [Crossref] [PubMed]
- Kim JR, Moon YJ, Kwon KS, et al. Tumor infiltrating PD1-positive lymphocytes and the expression of PD-L1 predict poor prognosis of soft tissue sarcomas. PLoS One 2013;8:e82870 [Crossref] [PubMed]
- Tawbi HA, Burgess M, Bolejack V, et al. Pembrolizumab in advanced soft-tissue sarcoma and bone sarcoma (SARC028): a multicentre, two-cohort, single-arm, open-label, phase 2 trial. Lancet Oncol 2017;18:1493-501. [Crossref] [PubMed]
- Maki RG, Jungbluth AA, Gnjatic S, et al. A Pilot Study of Anti-CTLA4 Antibody Ipilimumab in Patients with Synovial Sarcoma. Sarcoma 2013;2013:168145 [Crossref] [PubMed]
- D'Angelo SP, Mahoney MR, Van Tine BA, et al. Nivolumab with or without ipilimumab treatment for metastatic sarcoma (Alliance A091401): two open-label, non-comparative, randomised, phase 2 trials. Lancet Oncol 2018;19:416-26. [Crossref] [PubMed]
- Monga V, Skubitz KM, Maliske S, et al. A Retrospective Analysis of the Efficacy of Immunotherapy in Metastatic Soft-Tissue Sarcomas. Cancers (Basel) 2020;12:1873. [Crossref] [PubMed]
- Quiroga D, Liebner DA, Philippon JS, et al. Activity of PD1 inhibitor therapy in advanced sarcoma: a single-center retrospective analysis. BMC Cancer 2020;20:527. [Crossref] [PubMed]
- Hellmann MD, Paz-Ares L, Bernabe Caro R, et al. Nivolumab plus Ipilimumab in Advanced Non-Small-Cell Lung Cancer. N Engl J Med 2019;381:2020-31. [Crossref] [PubMed]
- Zayac A, Almhanna K. Esophageal, gastric cancer and immunotherapy: small steps in the right direction? Transl Gastroenterol Hepatol 2020;5:9. [Crossref] [PubMed]
- Keung EZ, Burgess M, Salazar R, et al. Correlative Analyses of the SARC028 Trial Reveal an Association Between Sarcoma-Associated Immune Infiltrate and Response to Pembrolizumab. Clin Cancer Res 2020;26:1258-66. [Crossref] [PubMed]
- Petitprez F, de Reyniès A, Keung EZ, et al. B cells are associated with survival and immunotherapy response in sarcoma. Nature 2020;577:556-60. [Crossref] [PubMed]
- Lee MY, Bernabe-Ramirez C, Ramirez DC, et al. Follicular dendritic cell sarcoma and its response to immune checkpoint inhibitors nivolumab and ipilimumab. BMJ Case Rep 2020;13:e234363 [Crossref] [PubMed]
- Gounder M, Desai V, Kuk D, et al. Impact of surgery, radiation and systemic therapy on the outcomes of patients with dendritic cell and histiocytic sarcomas. Eur J Cancer 2015;51:2413-22. [Crossref] [PubMed]
- Lala B, Kalbasi A, Singh AS. Role and Therapeutic Implications of MDSCs in Sarcomas. In: D'Angelo SP, Pollack SM, editors. Immunotherapy of Sarcoma. Cham: Springer International Publishing, 2019:3-15.
- Ostrand-Rosenberg S, Fenselau C. Myeloid-Derived Suppressor Cells: Immune-Suppressive Cells That Impair Antitumor Immunity and Are Sculpted by Their Environment. J Immunol 2018;200:422-31. [Crossref] [PubMed]
- Corzo CA, Condamine T, Lu L, et al. HIF-1α regulates function and differentiation of myeloid-derived suppressor cells in the tumor microenvironment. J Exp Med 2010;207:2439-53. [Crossref] [PubMed]
- Svoronos N, Perales-Puchalt A, Allegrezza MJ, et al. Tumor Cell-Independent Estrogen Signaling Drives Disease Progression through Mobilization of Myeloid-Derived Suppressor Cells. Cancer Discov 2017;7:72-85. [Crossref] [PubMed]
- Young MR, Kolesiak K, Wright MA, et al. Chemoattraction of femoral CD34+ progenitor cells by tumor-derived vascular endothelial cell growth factor. Clin Exp Metastasis 1999;17:881-8. [Crossref] [PubMed]
- Bunt SK, Sinha P, Clements VK, et al. Inflammation induces myeloid-derived suppressor cells that facilitate tumor progression. J Immunol 2006;176:284-90. [Crossref] [PubMed]
- Tu S, Bhagat G, Cui G, et al. Overexpression of interleukin-1beta induces gastric inflammation and cancer and mobilizes myeloid-derived suppressor cells in mice. Cancer Cell 2008;14:408-19. [Crossref] [PubMed]
- Zhao X, Rong L, Zhao X, et al. TNF signaling drives myeloid-derived suppressor cell accumulation. J Clin Invest 2012;122:4094-104. [Crossref] [PubMed]
- Halama N, Zoernig I, Berthel A, et al. Tumoral Immune Cell Exploitation in Colorectal Cancer Metastases Can Be Targeted Effectively by Anti-CCR5 Therapy in Cancer Patients. Cancer Cell 2016;29:587-601. [Crossref] [PubMed]
- Sano M, Ijichi H, Takahashi R, et al. Blocking CXCLs-CXCR2 axis in tumor-stromal interactions contributes to survival in a mouse model of pancreatic ductal adenocarcinoma through reduced cell invasion/migration and a shift of immune-inflammatory microenvironment. Oncogenesis 2019;8:8. [Crossref] [PubMed]
- D'Alterio C, Buoncervello M, Ieranò C, et al. Targeting CXCR4 potentiates anti-PD-1 efficacy modifying the tumor microenvironment and inhibiting neoplastic PD-1. J Exp Clin Cancer Res 2019;38:432. [Crossref] [PubMed]
- Lamba JK, Chauhan L, Shin M, et al. CD33 Splicing Polymorphism Determines Gemtuzumab Ozogamicin Response in De Novo Acute Myeloid Leukemia: Report From Randomized Phase III Children's Oncology Group Trial AAML0531. J Clin Oncol 2017;35:2674-82. [Crossref] [PubMed]
- Wang Y, Jia A, Bi Y, et al. Targeting Myeloid-Derived Suppressor Cells in Cancer Immunotherapy. Cancers (Basel) 2020;12:2626. [Crossref] [PubMed]
- Chen PT, Hsieh CC, Wu CT, et al. 1α,25-Dihydroxyvitamin D3 Inhibits Esophageal Squamous Cell Carcinoma Progression by Reducing IL6 Signaling. Mol Cancer Ther 2015;14:1365-75. [Crossref] [PubMed]
- Heine A, Flores C, Gevensleben H, et al. Targeting myeloid derived suppressor cells with all-trans retinoic acid is highly time-dependent in therapeutic tumor vaccination. Oncoimmunology 2017;6:e1338995 [Crossref] [PubMed]
- Wilky BA, Trucco MM, Subhawong TK, et al. Axitinib plus pembrolizumab in patients with advanced sarcomas including alveolar soft-part sarcoma: a single-centre, single-arm, phase 2 trial. Lancet Oncol 2019;20:837-48. [Crossref] [PubMed]
- Long AH, Highfill SL, Cui Y, et al. Reduction of MDSCs with All-trans Retinoic Acid Improves CAR Therapy Efficacy for Sarcomas. Cancer Immunol Res 2016;4:869-80. [Crossref] [PubMed]
- Zhou Q, Xian M, Xiang S, et al. All-Trans Retinoic Acid Prevents Osteosarcoma Metastasis by Inhibiting M2 Polarization of Tumor-Associated Macrophages. Cancer Immunol Res 2017;5:547-59. [Crossref] [PubMed]
- Devalaraja S, To TKJ, Folkert IW, et al. Tumor-Derived Retinoic Acid Regulates Intratumoral Monocyte Differentiation to Promote Immune Suppression. Cell 2020;180:1098-114.e16. [Crossref] [PubMed]
- Atri C, Guerfali FZ, Laouini D. Role of Human Macrophage Polarization in Inflammation during Infectious Diseases. Int J Mol Sci 2018;19:1801. [Crossref] [PubMed]
- Dancsok AR, Gao D, Lee AF, et al. Tumor-associated macrophages and macrophage-related immune checkpoint expression in sarcomas. Oncoimmunology 2020;9:1747340 [Crossref] [PubMed]
- Zhang QW, Liu L, Gong CY, et al. Prognostic significance of tumor-associated macrophages in solid tumor: a meta-analysis of the literature. PLoS One 2012;7:e50946 [Crossref] [PubMed]
- Comprehensive and Integrated Genomic Characterization of Adult Soft Tissue Sarcomas. Cell 2017;171:950-65.e28. [Crossref] [PubMed]
- Edris B, Fletcher JA, West RB, et al. Comparative gene expression profiling of benign and malignant lesions reveals candidate therapeutic compounds for leiomyosarcoma. Sarcoma 2012;2012:805614 [Crossref] [PubMed]
- Nafia I, Toulmonde M, Bortolotto D, et al. IDO Targeting in Sarcoma: Biological and Clinical Implications. Front Immunol 2020;11:274. [Crossref] [PubMed]
- Blanke CD, Demetri GD, von Mehren M, et al. Long-term results from a randomized phase II trial of standard- versus higher-dose imatinib mesylate for patients with unresectable or metastatic gastrointestinal stromal tumors expressing KIT. J Clin Oncol 2008;26:620-5. [Crossref] [PubMed]
- Cavnar MJ, Zeng S, Kim TS, et al. KIT oncogene inhibition drives intratumoral macrophage M2 polarization. J Exp Med 2013;210:2873-86. [Crossref] [PubMed]
- Tan HY, Wang N, Lam W, et al. Targeting tumour microenvironment by tyrosine kinase inhibitor. Mol Cancer 2018;17:43. [Crossref] [PubMed]
- Li C, Jiang P, Wei S, et al. Regulatory T cells in tumor microenvironment: new mechanisms, potential therapeutic strategies and future prospects. Mol Cancer 2020;19:116. [Crossref] [PubMed]
- Colombo MP, Piconese S. Regulatory-T-cell inhibition versus depletion: the right choice in cancer immunotherapy. Nat Rev Cancer 2007;7:880-7. [Crossref] [PubMed]
- Que Y, Xiao W, Guan YX, et al. PD-L1 Expression Is Associated with FOXP3+ Regulatory T-Cell Infiltration of Soft Tissue Sarcoma and Poor Patient Prognosis. J Cancer 2017;8:2018-25. [Crossref] [PubMed]
- Florou V, Wilky BA. Regulatory T cells. In: D'Angelo SP, Pollack SM, editors. Immunotherapy of Sarcoma. Cham: Springer International Publishing, 2019:33-45.
- Kochetkova I, Golden S, Holderness K, et al. IL-35 stimulation of CD39+ regulatory T cells confers protection against collagen II-induced arthritis via the production of IL-10. J Immunol 2010;184:7144-53. [Crossref] [PubMed]
- Lee CR, Kwak Y, Yang T, et al. Myeloid-Derived Suppressor Cells Are Controlled by Regulatory T Cells via TGF-β during Murine Colitis. Cell Rep 2016;17:3219-32. [Crossref] [PubMed]
- Huang B, Pan PY, Li Q, et al. Gr-1+CD115+ immature myeloid suppressor cells mediate the development of tumor-induced T regulatory cells and T-cell anergy in tumor-bearing host. Cancer Res 2006;66:1123-31. [Crossref] [PubMed]
- Wisdom AJ, Mowery YM, Kirsch DG. Radiation and Immunotherapy for Sarcoma. In: D'Angelo SP, Pollack SM, editors. Immunotherapy of Sarcoma. Cham: Springer International Publishing, 2019:47-65.
- Corso CD, Ali AN, Diaz R. Radiation-induced tumor neoantigens: imaging and therapeutic implications. Am J Cancer Res 2011;1:390-412. [PubMed]
- Yi M, Qin S, Zhao W, et al. The role of neoantigen in immune checkpoint blockade therapy. Exp Hematol Oncol 2018;7:28. [Crossref] [PubMed]
- Ngwa W, Irabor OC, Schoenfeld JD, et al. Using immunotherapy to boost the abscopal effect. Nat Rev Cancer 2018;18:313-22. [Crossref] [PubMed]
- Twyman-Saint Victor C, Rech AJ, Maity A, et al. Radiation and dual checkpoint blockade activate non-redundant immune mechanisms in cancer. Nature 2015;520:373-7. [Crossref] [PubMed]
- Callaghan CM, Seyedin SN, Mohiuddin IH, et al. The Effect of Concurrent Stereotactic Body Radiation and Anti-PD-1 Therapy for Recurrent Metastatic Sarcoma. Radiat Res 2020;194:124-32. [Crossref] [PubMed]
- Monga V, Maliske SM, Milhem M. Oncolytic Virus Immunotherapy in Sarcoma. In: D'Angelo SP, Pollack SM, editors. Immunotherapy of Sarcoma. Cham: Springer International Publishing, 2019:69-116.
- Zheng M, Huang J, Tong A, et al. Oncolytic Viruses for Cancer Therapy: Barriers and Recent Advances. Mol Ther Oncolytics 2019;15:234-47. [Crossref] [PubMed]
- Kelly CM, Antonescu CR, Bowler T, et al. Objective Response Rate Among Patients With Locally Advanced or Metastatic Sarcoma Treated With Talimogene Laherparepvec in Combination With Pembrolizumab: A Phase 2 Clinical Trial. JAMA Oncol 2020;6:402-8. [Crossref] [PubMed]
- Le Cesne A, Marec-Berard P, Blay JY, et al. Programmed cell death 1 (PD-1) targeting in patients with advanced osteosarcomas: results from the PEMBROSARC study. Eur J Cancer 2019;119:151-7. [Crossref] [PubMed]
- Pollack S, Redman MW, Wagner M, et al. A phase I/II study of pembrolizumab (Pem) and doxorubicin (Dox) in treating patients with metastatic/unresectable sarcoma. J Clin Oncol 2019;37:11009. [Crossref]
- Gordon EM, Chua-Alcala VS, Kim K, et al. SAINT: Results of a phase 1/2 study of safety/efficacy using safe amounts of ipilimumab, nivolumab, and trabectedin as first-line treatment of advanced soft tissue sarcoma. J Clin Oncol 2019;37:11016. [Crossref]
- Mata M, Gottschalk S. Adoptive cell therapy for sarcoma. Immunotherapy 2015;7:21-35. [Crossref] [PubMed]
- Harris DT, Kranz DM, Adoptive T. Cell Therapies: A Comparison of T Cell Receptors and Chimeric Antigen Receptors. Trends Pharmacol Sci 2016;37:220-30. [Crossref] [PubMed]
- Neelapu SS, Locke FL, Go WY. CAR T-Cell Therapy in Large B-Cell Lymphoma. N Engl J Med 2018;378:1065. [Crossref] [PubMed]
- Park JH, Rivière I, Gonen M, et al. Long-Term Follow-up of CD19 CAR Therapy in Acute Lymphoblastic Leukemia. N Engl J Med 2018;378:449-59. [Crossref] [PubMed]
- Wang M, Munoz J, Goy A, et al. KTE-X19 CAR T-Cell Therapy in Relapsed or Refractory Mantle-Cell Lymphoma. N Engl J Med 2020;382:1331-42. [Crossref] [PubMed]
- Ramachandran I, Lowther DE, Dryer-Minnerly R, et al. Systemic and local immunity following adoptive transfer of NY-ESO-1 SPEAR T cells in synovial sarcoma. J Immunother Cancer 2019;7:276. [Crossref] [PubMed]
- Grünwald V, Bauer S, Hermes B, et al. A randomized phase II study of durvalumab and tremelimumab compared to doxorubicin in patients with advanced or metastatic soft tissue sarcoma (MEDISARC, AIO-STS 0415). J Clin Oncol 2019;37:TPS11075 [Crossref]
- Yue W, Chen L, Yu L, et al. Checkpoint blockade and nanosonosensitizer-augmented noninvasive sonodynamic therapy combination reduces tumour growth and metastases in mice. Nat Commun 2019;10:2025. [Crossref] [PubMed]
- Luchtel RA, Bhagat T, Pradhan K, et al. High-dose ascorbic acid synergizes with anti-PD1 in a lymphoma mouse model. Proc Natl Acad Sci U S A 2020;117:1666-77. [Crossref] [PubMed]
- Nie J, Wang C, Liu Y, et al. Addition of Low-Dose Decitabine to Anti–PD-1 Antibody Camrelizumab in Relapsed/Refractory Classical Hodgkin Lymphoma. J Clin Oncol 2019;37:1479-89. [Crossref] [PubMed]
- Shenoy N, Bhagat TD, Cheville J, et al. Ascorbic acid-induced TET activation mitigates adverse hydroxymethylcytosine loss in renal cell carcinoma. J Clin Invest 2019;129:1612-25. [Crossref] [PubMed]
- Ghoneim HE, Fan Y, Moustaki A, et al. De Novo Epigenetic Programs Inhibit PD-1 Blockade-Mediated T Cell Rejuvenation. Cell 2017;170:142-57.e19. [Crossref] [PubMed]