This article has an erratum available at: http://dx.doi.org/10.21037/atm-2024-14 the article has been update on 2024-08-05 at here.
Butyrate ameliorates alcoholic fatty liver disease via reducing endotoxemia and inhibiting liver gasdermin D-mediated pyroptosis
Introduction
Alcoholic fatty liver disease (AFLD), which is characterized by hepatic steatosis and is associated with increased risk of cirrhosis and hepatocellular carcinoma, is a rising global health concern (1). Previous studies have shown that oxidative stress, hypoxia, and inflammatory cytokines play critical roles in the pathogenesis of AFLD (2-5). However, the mechanism of AFLD has yet to be elucidated in detail.
The microbiome, which has been referred to as the “forgotten organ”, influences the initiation, perpetuation, and aggravation of liver diseases through the gut-liver axis (6-8). Chronic alcohol exposure is believed to result in a compositional change in and/or dysbiosis of the microbiome (9). Short-chain fatty acids (SCFAs), including acetate, propionate, butyrate, and valerate, are predominantly produced by gut microbiota during the fermentation of dietary fiber and indigestible carbohydrates (10,11), and they are crucial for improving the inflammation in liver disease (12). Of note, butyrate, a key energy source for intestinal epithelial cells, has been shown to protect against insulin resistance, hepatic steatosis and inflammation in high-fat diet-induced obesity, (13-16). Furthermore, evidence from multiple studies has shown that butyrate protected against the development of colitis and colorectal cancer (17-19). In our previous study, we found butyrate supplementation ameliorated endotoxemia in db/db mice through maintaining the intestinal barrier integrity (20). However, the effects of butyrate on AFLD have yet to be determined.
Pyroptosis is a form of programmed cell death initiated by inflammatory caspase activation (21). Gasdermin D (GSDMD) is the ultimate executor of pyroptosis via active GSDMD N-terminal (GSDMD-NT), which results in the release of mature interleukin (IL)-1β, IL-18, and other inflammatory factors (22,23). Pyroptosis is vital to microbial infection control and modulates specific enteric pathogenic infections (24,25). A previous study found pyroptosis to be involved in the pathogenesis of alcoholic hepatitis (26). However, how alcohol exposure induces hepatocyte pyroptosis is still unclear. In this work, we aimed to investigate whether butyrate can attenuate hepatic steatosis and the related underlying mechanism by establishing a mouse model of AFLD. We found that butyrate ameliorated AFLD by reducing gut leakage and inhibiting GSDMD-mediated pyroptosis in liver.
We present the following article following the ARRIVE reporting checklist (available at http://dx.doi.org/10.21037/atm-21-2158).
Methods
Animals
Eight-week-old male specific pathogen-free (SPF) C57BL/6 mice were purchased from SPF Biotechnology Co., Ltd. (Beijing, China). The mice were randomly divided into the following four groups (n=6 per group): normal control (NC) group (0.9% saline); the alcohol group [30% vol/vol alcohol 5 g/(kg·d) by gavage]; the butyrate group [butyrate (Sigma-Aldrich, USA) dissolved in 0.9% saline solution, gavage with 0.5 g/(kg·d)]; and the alcohol + butyrate group [30% vol/vol alcohol 5 g/(kg·d) by gavage, followed by butyrate 0.5 g/(kg·d) by gavage after a 1-hour interval]. All interventions were conducted continuously for 6 months. All of the mice were fed a normal chow diet, and were bred and maintained in our certified animal facility under a 12-hour light-dark cycle at 22 °C. The animal experiments were approved by the Ethics Review Committee of Southwest Medical University (No. 2020ZRQNA009). All experiments were performed in accordance with the approved guidelines and followed the National Institutes of Health Guidelines for the Care and Use of Laboratory Animals.
Hematoxylin-eosin (H&E) staining and immunohistochemistry
Liver and colon tissues collected from the mice were fixed in 4% paraformaldehyde for 24 hours and embedded in paraffin. For observation, 6-µm tissue sections were subjected to H&E staining and observed with a light microscope (Leica, Japan). For immunohistochemistry, sections were incubated firstly with primary antibodies overnight, then with biotin-conjugated secondary antibody. After that, the sections were treated with streptavidin-biotin-enzyme complex to develop a brown deposit (positive staining). Finally, Nucleotide binding and oligomerization domain-like receptor family pyrin domain-containing 3 (NLRP3) (CST, USA) and GSDMD (CST, USA) in the liver tissues and Zona Occludens protein-1(ZO-1) (Santa Cruz, USA) in the colon tissues were evaluated.
Analysis of serum parameters
Blood samples were collected from the mice and centrifuged at 3,000 revolutions per minute for 10 minutes at 4 °C. Then, the serum was collected, and the serum levels of ethanol (Bioassay, USA). ELISA kits for serum lipopolysaccharide (LPS), Interleukine-1 beta (IL-1β), and Interleukine-18 (IL-18) were purchased from Cheng Lin biotechnology company (Beijing, China). Biochemical parameters including AST, ALT and TG (Nanjing Jiancheng Bio, Nanjing, China) were also assessed. These parameters were determined using kits according to the manufacturers’ protocols.
Gut microbiota composition analysis
Fecal samples were suspended in Tris-EDTA buffer supplemented with 10% sodium dodecyl sulfate (SDS), and DNA was extracted according to a standard method. The V3–V4 regions of the bacterial 16S ribosomal RNA gene were amplified using Phusion® High-Fidelity PCR Master Mix with GC Buffer (New England Biolabs, USA). PCR products were extracted by 2% agarose gel electrophoresis. A TruSeq® DNA PCR-Free Sample Preparation Kit (Illumina, USA) was used to generate sequencing libraries. Finally, the sequence was analyzed on a HiSeq2500 PE250 platform. The sequencing data were processed with data filtering and operational taxonomic unit (OTU) (Novogene Technology Co., Ltd., Beijing, China).
Cell culture, treatment, and viability assay
Human normal hepatocytes (LO2 cells) were cultured in Roswell Park Memorial Institute 1640 Medium (Gibco, USA) with 10% fetal bovine serum (FBS) (Gibco, USA) and 1% penicillin/streptomycin (Beyotime, China). LO2 cells were seeded into a 96-well plates and treated with vehicle, different concentrations of alcohol, LPS, butyrate, alcohol + butyrate, or LPS + butyrate for 24 hours. Cell viability was determined using an MTT assay. According to the MTT results, 100 mM alcohol or 50 µg/mL LPS, with or without 0.1 mM butyrate, was used for the in vitro experiments.
Western blotting
Total protein was extracted from LO2 cells or liver tissue from mice using RIPA lysis buffer (Beyotime, China) according to the manufacturer’s instructions. A bicinchoninic acid (BCA) protein assay kit (Beyotime, China) was used to assess protein concentrations. Western blotting was conducted as previously described (27). The following primary antibodies were used: GSDMD (1:1,000, Abcam, UK), cleaved-GSDMD (1:1,000, CST,USA), NLRP3 (1:1,000, CST,USA), caspase-1 (1:1,000, Abcam, UK), IL-1β (1:1,000, Abcam, UK), β-Actin (1:1,000, Beyotime, China). The secondary antibodies used were mouse immunoglobulin (IgG) (1:5,000, Beyotime, China) and rabbit IgG (1: 5,000, Beyotime, China). The proteins were detected using a horseradish peroxidase chemiluminescent reagent (Millipore, USA). Images were captured with the UVP imaging system (Bio-Rad, USA), and quantified using Quantity One software.
Statistical analysis
All data were expressed as means ± standard deviations (SDs). GraphPad Prism 6.2 software (GraphPad Software Inc., USA) was used for the statistical analyses. An independent-samples t test was used to compare 2 groups, and unpaired t test with Welch’s correction was used, if heterogeneity of variance, with P<0.05 indicating a significant difference
Results
Butyrate ameliorated AFLD in mice
To investigate the effects of butyrate on AFLD, mice were treated with saline or alcohol with or without butyrate for 6 months. During the intervention, serum ethanol levels were measured to evaluated the reliability of the animal model. As shown in Figure 1A, the serum ethanol levels in the alcohol group and alcohol + butyrate group were significantly increased after 3 months. The body weight of mice was monitored each month (Figure 1B). Compared with the NC group, the body weight in the alcohol group was decreased, but there was no significant change in the body weight of the butyrate group. Subsequently, H&E and Oil Red O staining showed that fat accumulation in the liver was increased in the alcohol group compared to the NC group, but butyrate rescued fat accumulation (Figure 1C,D). Furthermore, compared to NC group, in the alcohol group serum ALT was elevated without significant difference, while serum AST was increased significantly. And compared to the alcohol group, butyrate supplementation decreased the serum ALT and AST with no difference (Figure 1E,F). In addition, in the alcohol group serum TG was just increased without significant difference and intrahepatic TG was increased significantly compared with NC group, while butyrate supplementation significantly decreased the intrahepatic TG content (Figure 1G,H). These findings indicated that butyrate ameliorated AFLD in mice.
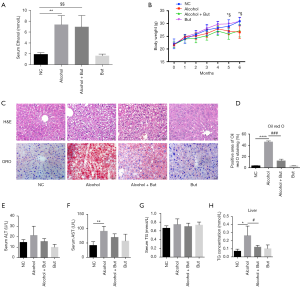
Butyrate improved AFLD via inhibiting GSDMD-mediated pyroptosis
Inflammation has been revealed as a vital player in the pathological progression of AFLD (28). To further explore the underlying mechanism of butyrate’s amelioration effect on AFLD, we detected the levels of inflammatory factors in mouse serum. We found that butyrate supplementation reduced the serum IL-1β and IL-18 levels induced by chronic alcohol feeding (Figure 2A,B). Furthermore, compared to the NC group, the alcohol group displayed significantly increases in the protein expression of NLRP3 and GSDMD (Figure 2C,D). Accordingly, western blot showed that active caspase-1, GSDMD-NT and IL-1β were elevated significantly in the alcohol group, while butyrate rescued the increase in liver inflammation (Figure 2E,F). These results indicated that butyrate supplementation inhibited GSDMD-mediated inflammation in mice with AFLD.
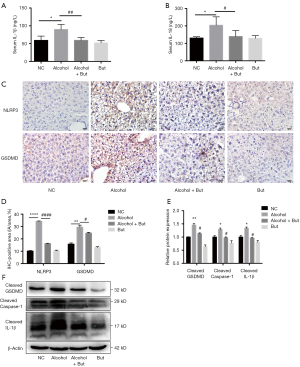
To verify whether butyrate plays a direct role on hepatocytes, we conducted in vitro experiments in LO2 cells. According to MTT assay, the dose of LPS above 100 µg/mL, butyrate above 1 mM and alcohol above 200 mM decreased cell viability significantly (Figure 3A,B,C). Since we found the dose of butyrate at 0.1 mM increased significantly cell viability (Figure 3B), we administrated gradient dose of butyrate under 0.5 mM to explore the protective effect of butyrate on LO2 cells with 100 µg/mL LPS or 200 mM alcohol (Figure 3D). So we chose 100 mM alcohol or 50 µg/mL LPS with or without 0.1 mM butyrate to treat LO2 cells in following experiment. Compared to the NC group, alcohol or LPS up-regulated the expression of cleaved GSDMD in LO2 cells, but the administration of butyrate did not inhibit GSDMD activation (Figure 3E,F,G,H). And transmission electron microscopy showed alcohol or LPS treatment induced the ultrastructural changes of pyroptosis in LO2 cells, such as cellular swelling (Figure 3I). Therefore, we speculated that butyrate may attenuate AFLD via reducing the absorption of LPS.
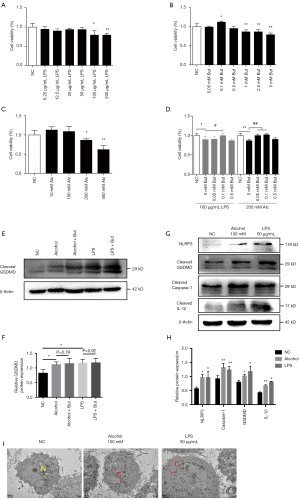
Butyrate stabilized the intestinal barrier and reduced endotoxemia induced by alcohol
Studies have shown that endotoxin from gut microbiota can induce a chronic inflammatory response, which is crucial for pathogenies of alcoholic liver disease (29,30). Therefore, we detected the levels of LPS in serum from the mice. Compared with the NC group, the alcohol group exhibited an increase in serum LPS, while butyrate reduced this increase (Figure 4A). H&E staining showed that inflammatory cell infiltration was increased in the colon tissue of mice in the alcohol group, while butyrate reduced alcohol-induced inflammatory cell infiltration (Figure 4B). Furthermore, alcohol was found to significantly decrease the expression of tight junction protein ZO-1 in the colon, while butyrate rescued ZO-1 expression (Figure 4C,D). These findings suggested that butyrate stabilized the intestinal barrier and reduced serum LPS levels induced by alcohol feeding.
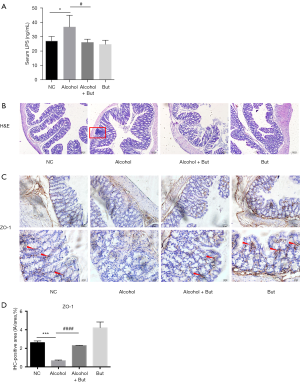
Butyrate failed to alleviate the dysbiosis and dysfunction of microbiome caused by chronic alcohol feeding
Considering that the gut microbiota is a major source of serum LPS, we wondered whether butyrate can modulate microbiota. Thus, we analyzed the composition of gut microbiota by high-throughput sequencing of 16S rRNA. Principal coordinates analysis plot (PCA Plot) was used to evaluated the phylogenetic similarities between microbial communities (Figure 5A). Venn diagram showed that 6,200 OTUs were shared in all of the four group. And the OUTs appeared in the alcohol group were fewer than those in NC group, while OUTs in the alcohol + but group were more than those in the alcohol group, which indicated butyrate increased the species of bacteria induced by alcohol (Figure 5B). Then the bacterial distribution in the different groups was analyzed (Figure 5C). And the relative abundance of bacteria at the phylum level showed that the main bacteria in NC group were Bacteroidetes, Firmicutes, Proteobacteria, and Cyanobacteria, and in the alcohol group mice, the levels of Proteobacteria and Cyanobacteria were reduced, but that of Actinobacteria was increased. Furthermore, in the alcohol + butyrate group, the abundance of Verrucomicrobia was increased (Figure 5C). As shown in the heatmap, the levels of normal bacteria were largely reduced in the alcohol group, while butyrate supplementation only reduced the abundance of Parabacteroides, Murbaculum, Cyanobacteria, and Klebsiella caused by alcohol exposure (Figure 5D). These findings indicated that butyrate didn’t significantly improve the imbalance of bacteria induced by alcohol. Additionally, the functional prediction showed that chronic alcohol exposure led to dysfunction of the gut microbiota, but the administration of butyrate failed to significantly alleviate this disorder (Figure 5E). These observations suggested that butyrate ameliorated alcohol-induced liver injury and inflammation in the mice by protecting the intestinal barrier and reducing endotoxemia (Figure 6).
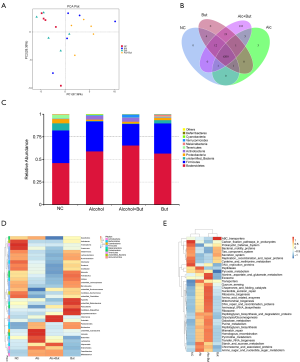
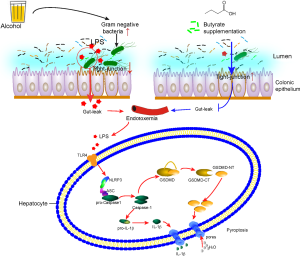
Discussion
In this study, we found that butyrate improved alcohol induced hepatic steatosis. In our mouse model, serum ALT showed no difference between the alcohol group and the control group, this is consistent with previous studies, which indicated that AFLD showed hepatic steatosis with or without elevation of transaminase (aspartate aminotransferase (AST)/alanine transaminase (ALT)) (31). In addition, H&E staining in our study showed that the liver in alcohol group just showed hepatocyte steatosis without obvious hepatocyte necrosis, so it’s reasonable that only intrahepatic TG in alcohol group increased significantly, while serum TG did not.
The intestinal microenvironment is critical for the treatments of liver disease. Recently, with the increasing researches on pre-/pro-/synbiotics, fecal bacterial transplantation, bile acid, and adsorbents, manipulation of the gut microbiota has been largely studied on alcoholic liver disease (32-34). Butyrate, a fermentation product of gut microbiota, has been reported to be decreased following acute ethanol exposure (35). Studies have also indicated that butyrate could protect mice from non-alcoholic steatohepatitis and alcoholic liver injury (15,35). In this work, we found that butyrate supplementation improved alcohol-induced hepatic steatosis and inflammation, and that chronic alcohol feeding resulted in intestinal microbiota dysbiosis and dysfunction. Briefly, alcohol reduced the abundance of Proteobacteria and Cyanobacteria, while increased Actinobacteria at the phylum level, which was inconsistent with previous studies. Yue et al. found that chronic alcohol feeding significantly reduced Verrucomicrobiaceae and Sutterellaceae at the family level (36). Furthermore, butyrate administration failed to significantly alleviate alcohol-induced intestinal microbiota disorder. However, butyrate did increase the abundance of Verrucomicrobia, while reducing that of Parabacteroides, Murbaculum, Cyanobacteria, and Klebsiella in mice with alcohol exposure. Ye et al. described that butyrate had a protective effect against non-alcoholic steatohepatitis in mice fed a methionine-choline-deficient diet via improving the gut microbiome and metabolome, and barrier function (15). We speculate that the reasons for the variation in different studies may be related to differences in the pattern and duration of alcohol feeding, and the different butyrate dose used. Moreover, the animal model of AFLD used in the present work differs from those in previous studies. In our study, chronic alcohol exposure by gavage lasted for up to 6 months; however, in Yue et al.’s research, mice were administrated an ethanol-containing Lieber-DeCarli liquid diet for 8 weeks.
Alcohol-induced endotoxemia is mainly caused by intestinal flora imbalance and intestinal barrier disruption (29,37). Although butyrate did not improve the alcohol-induced disorder of the gut microbiota, butyrate supplementation did reduce the levels of endotoxin and rescued the decrease in tight junction protein ZO-1 induced by chronic alcohol feeding; this observation was consistent with the findings of a previous study (38). Meanwhile, our previous work suggested butyrate promoted the proliferation of the gut epithelial cell line Caco-2 (20). Thus, we speculate that butyrate can ameliorate AFLD via maintaining the intestinal barrier. We also infer that butyrate reduces LPS levels, mainly by improving the intestinal barrier, and thus inhibits liver and systemic inflammation.
Liver parenchymal cells and macrophages respond to LPS via binding to Toll-like receptors, and induce liver damage (39,40). Studies have reported that pyroptosis occurs in response to microbial infection and modulates specific enteric pathogenic infections (24,25). Khanova et al. found caspase-11/4-GSDMD-mediated pyroptosis to be involved in alcoholic hepatitis (26). NLRP3-mediated pyroptosis has also been implicated in liver damage via its induction of severe liver inflammation and fibrosis (41). In this study, we found that caspase-1/GSDMD mediated canonical pyroptosis participated in the development of AFLD. Khanova et al. indicated that caspase-11 (caspase-4 in humans) involved in the non-canonical pyroptosis pathway was activated in mice and patients with alcoholic hepatitis but not in mice with chronic alcoholic steatohepatitis or healthy humans. We think that alcoholic hepatitis is an acute exacerbation stage of chronic AFLD with different pathological changes in the liver, and the hepatic bacterial load varied, which may be the reason for the inconsistent result in our study with other studies.
Furthermore, our in vitro experiments demonstrated that alcohol or LPS induced pyroptosis in LO2 cells, and that butyrate did not relieve cell injury induced by alcohol or LPS. Therefore, we think that butyrate ameliorates AFLD via improving gut barrier integrity, reducing enterogenous endotoxemia, thus inhibits LPS induced GSDMD medicated pyrotopsis. In the development of alcoholic liver disease, hepatocytes and macrophages respond to LPS by binding to Toll-like receptors, and are involved in the inflammatory response. Therefore, further observation of the effects of butyrate on co-culture of hepatocytes and immune cells is needed. Research has also found that butyrate regulates the inflammatory immune response by inhibiting histone deacetylase (42,43). Therefore, it is likely that the protective effect of butyrate on hepatocytes depends on other mechanisms.
Conclusions
In summary, we observed that butyrate ameliorates alcohol-induced hepatic steatosis and inflammation by down-regulating GSDMD-mediated pyroptosis. We speculate that butyrate improves AFLD mainly by maintaining intestinal barrier function and alleviating gut leakage. We will further verify the mechanism underpinning the effect of butyrate on alcohol-induced intestinal barrier changes in our future study.
Acknowledgments
Funding: This work was supported by Sichuan Science and Technology Program (No. 2019YFS0537, 2020YFS0456) and Natural Science Foundation of China (No. 81970676).
Footnote
Reporting Checklist: The authors have completed the ARRIVE reporting checklist. Available at http://dx.doi.org/10.21037/atm-21-2158
Data Sharing Statement: Available at http://dx.doi.org/10.21037/atm-21-2158
Conflicts of Interest: All authors have completed the ICMJE uniform disclosure form (available at http://dx.doi.org/10.21037/atm-21-2158). The authors have no conflicts of interest to declare.
Ethical Statement: The authors are accountable for all aspects of the work in ensuring that questions related to the accuracy or integrity of any part of the work are appropriately investigated and resolved. The animal experiments were approved by the Ethics Review Committee of Southwest Medical University (No. 2020ZRQNA009). All experiments were performed in accordance with the approved guidelines and followed the National Institutes of Health Guidelines for the Care and Use of Laboratory Animals.
Open Access Statement: This is an Open Access article distributed in accordance with the Creative Commons Attribution-NonCommercial-NoDerivs 4.0 International License (CC BY-NC-ND 4.0), which permits the non-commercial replication and distribution of the article with the strict proviso that no changes or edits are made and the original work is properly cited (including links to both the formal publication through the relevant DOI and the license). See: https://creativecommons.org/licenses/by-nc-nd/4.0/.
References
- O'Shea RS, Dasarathy S, McCullough AJ. Alcoholic liver disease. Hepatology 2010;51:307-28. [Crossref] [PubMed]
- Purohit V, Gao B, Song BJ. Molecular mechanisms of alcoholic fatty liver. Alcohol Clin Exp Res 2009;33:191-205. [Crossref] [PubMed]
- Nishiyama Y, Goda N, Mai K, et al. HIF-1α induction suppresses excessive lipid accumulation in alcoholic fatty liver in mice. J Hepatol 2012;56:441-7. [Crossref] [PubMed]
- Kurose I, Higuchi H, Miura S, et al. Oxidative stress-mediated apoptosis of hepatocytes exposed to acute ethanol intoxication. Hepatology 1997;25:368-78. [Crossref] [PubMed]
- Szabo G, Csak T. Inflammasomes in liver diseases. J Hepatol 2012;57:642-54. [Crossref] [PubMed]
- Chassaing B, Etienne-Mesmin L, Gewirtz AT. Microbiota-liver axis in hepatic disease. Hepatology 2014;59:328-39. [Crossref] [PubMed]
- Tilg H, Cani PD, Mayer EA. Gut microbiome and liver diseases. Gut 2016;65:2035-44. [Crossref] [PubMed]
- Li B, Selmi C, Tang R, et al. The microbiome and autoimmunity: a paradigm from the gut-liver axis. Cell Mol Immunol 2018;15:595-609. [Crossref] [PubMed]
- Llopis M, Cassard AM, Wrzosek L, et al. Intestinal microbiota contributes to individual susceptibility to alcoholic liver disease. Gut 2016;65:830-9. [Crossref] [PubMed]
- Schönfeld P, Wojtczak L. Short- and medium-chain fatty acids in energy metabolism: the cellular perspective. J Lipid Res 2016;57:943-54. [Crossref] [PubMed]
- Priyadarshini M, Kotlo KU, Dudeja PK, et al. Role of Short Chain Fatty Acid Receptors in Intestinal Physiology and Pathophysiology. Compr Physiol 2018;8:1091-115. [Crossref] [PubMed]
- Jiang XW, Li YT, Ye JZ, et al. New strain of Pediococcus pentosaceus alleviates ethanol-induced liver injury by modulating the gut microbiota and short-chain fatty acid metabolism. World J Gastroenterol 2020;26:6224-40. [Crossref] [PubMed]
- Mattace Raso G, Simeoli R, Russo R, et al. Effects of sodium butyrate and its synthetic amide derivative on liver inflammation and glucose tolerance in an animal model of steatosis induced by high fat diet. PLoS One 2013;8:e68626 [Crossref] [PubMed]
- Zhou D, Pan Q, Xin FZ, et al. Sodium butyrate attenuates high-fat diet-induced steatohepatitis in mice by improving gut microbiota and gastrointestinal barrier. World J Gastroenterol 2017;23:60-75. [Crossref] [PubMed]
- Ye J, Lv L, Wu W, et al. Butyrate Protects Mice Against Methionine-Choline-Deficient Diet-Induced Non-alcoholic Steatohepatitis by Improving Gut Barrier Function, Attenuating Inflammation and Reducing Endotoxin Levels. Front Microbiol 2018;9:1967. [Crossref] [PubMed]
- Huang W, Zhou L, Guo H, et al. The role of short-chain fatty acids in kidney injury induced by gut-derived inflammatory response. Metabolism 2017;68:20-30. [Crossref] [PubMed]
- Vinolo MA, Rodrigues HG, Nachbar RT, et al. Regulation of inflammation by short chain fatty acids. Nutrients 2011;3:858-76. [Crossref] [PubMed]
- Sengupta S, Muir JG, Gibson PR. Does butyrate protect from colorectal cancer? J Gastroenterol Hepatol 2006;21:209-18. [Crossref] [PubMed]
- Donohoe DR, Holley D, Collins LB, et al. A gnotobiotic mouse model demonstrates that dietary fiber protects against colorectal tumorigenesis in a microbiota- and butyrate-dependent manner. Cancer Discov 2014;4:1387-97. [Crossref] [PubMed]
- Xu YH, Gao CL, Guo HL, et al. Sodium butyrate supplementation ameliorates diabetic inflammation in db/db mice. J Endocrinol 2018;238:231-44. [Crossref] [PubMed]
- Gaidt MM, Hornung V. Pore formation by GSDMD is the effector mechanism of pyroptosis. EMBO J 2016;35:2167-9. [Crossref] [PubMed]
- Sborgi L, Rühl S, Mulvihill E, et al. GSDMD membrane pore formation constitutes the mechanism of pyroptotic cell death. EMBO J 2016;35:1766-78. [Crossref] [PubMed]
- Man SM, Kanneganti TD. Gasdermin D: the long-awaited executioner of pyroptosis. Cell Res 2015;25:1183. [Crossref] [PubMed]
- Xia X, Wang X, Zheng Y, et al. What role does pyroptosis play in microbial infection? J Cell Physiol 2019;234:7885-92. [Crossref] [PubMed]
- Zhou CB, Fang JY. The role of pyroptosis in gastrointestinal cancer and immune responses to intestinal microbial infection. Biochim Biophys Acta Rev Cancer 2019;1872:1-10. [Crossref] [PubMed]
- Khanova E, Wu R, Wang W, et al. Pyroptosis by Caspase11/4-Gasdermin-D Pathway in Alcoholic Hepatitis. Hepatology 2017;33:1206. [PubMed]
- Gao C, Chen G, Liu L, et al. Impact of high glucose and proteasome inhibitor MG132 on histone H2A and H2B ubiquitination in rat glomerular mesangial cells. J Diabetes Res 2013;2013:589474 [Crossref] [PubMed]
- Teschke R. Alcoholic steatohepatitis (ASH) and alcoholic hepatitis (AH): cascade of events, clinical aspects, and pharmacotherapy options. Expert Opin Pharmacother 2018;19:779-93. [Crossref] [PubMed]
- Antón M, Rodriguez-Gonzalez A, Ballesta A, et al. Alcohol binge disrupts the rat intestinal barrier: the partial protective role of oleoylethanolamide. Br J Pharmacol 2018;175:4464-79. [Crossref] [PubMed]
- Wheeler MD. Endotoxin and Kupffer cell activation in alcoholic liver disease. Alcohol Res Health 2003;27:300-6. [PubMed]
- Singal AK, Bataller R, Ahn J, et al. ACG Clinical Guideline: Alcoholic Liver Disease. Am J Gastroenterol 2018;113:175-94. [Crossref] [PubMed]
- Wiest R, Albillos A, Trauner M, et al. Targeting the gut-liver axis in liver disease. J Hepatol 2017;67:1084-103. [Crossref] [PubMed]
- Hong M, Han DH, Hong J, et al. Are Probiotics Effective in Targeting Alcoholic Liver Diseases? Are Probiotics Effective in Targeting Alcoholic Liver Diseases?
- Bajaj JS. Alcohol, liver disease and the gut microbiota. Nat Rev Gastroenterol Hepatol 2019;16:235-46. [Crossref] [PubMed]
- Chen J, Xuan YH, Luo MX, et al. Kaempferol alleviates acute alcoholic liver injury in mice by regulating intestinal tight junction proteins and butyrate receptors and transporters. Toxicology 2020;429:152338 [Crossref] [PubMed]
- Yue R, Wei X, Zhao J, et al. Essential Role of IFN-γ in Regulating Gut Antimicrobial Peptides and Microbiota to Protect Against Alcohol-Induced Bacterial Translocation and Hepatic Inflammation in Mice. Front Physiol 2021;11:629141 [Crossref] [PubMed]
- Purohit V, Bode JC, Bode C, et al. Alcohol, intestinal bacterial growth, intestinal permeability to endotoxin, and medical consequences: Summary of a symposium. Alcohol 2008;42:349-61. [Crossref] [PubMed]
- Cresci GA, Glueck B, McMullen MR, et al. Prophylactic tributyrin treatment mitigates chronic-binge ethanol-induced intestinal barrier and liver injury. J Gastroenterol Hepatol 2017;32:1587-97. [Crossref] [PubMed]
- Nolan JP. The role of intestinal endotoxin in liver injury: a long and evolving history. Hepatology 2010;52:1829-35. [Crossref] [PubMed]
- Mandrekar P, Szabo G. Signalling pathways in alcohol-induced liver inflammation. J Hepatol 2009;50:1258-66. [Crossref] [PubMed]
- Wree A, Eguchi A, Mcgeough MD, et al. NLRP3 inflammasome activation results in hepatocyte pyroptosis, liver inflammation, and fibrosis in mice. Hepatology 2014;59:898-910. [Crossref] [PubMed]
- Ye J, Wu W, Li Y, et al. Influences of the Gut Microbiota on DNA Methylation and Histone Modification. Dig Dis Sci 2017;62:1155-64. [Crossref] [PubMed]
- Li H, Gao H, Zhang J, et al. Sodium butyrate stimulates expression of fibroblast growth factor 21 in liver by inhibition of histone deacetylase 3. Diabetes 2012;61:797-806. [Crossref] [PubMed]