This article has an erratum available at: http://dx.doi.org/10.21037/atm-2024B-56 the article has been update on 2024-11-28 at here.
Myostatin is involved in skeletal muscle dysfunction in chronic obstructive pulmonary disease via Drp-1 mediated abnormal mitochondrial division
Introduction
Chronic obstructive pulmonary disease (COPD) is a preventable and treatable common disease characterized by persistent respiratory symptoms and airflow limitations in respiratory and critical care medicine, and major comorbidities may affect morbidity and mortality. In 2018, the results of a large-scale population study (i.e., the Chinese Adult Lung Health Study) by Wang et al. showed that the prevalence of COPD in China was 8.6% in adults aged 20 years and above, 13.7% in those aged 40 years and above, and more than 27% in those aged 60 years and above (1). COPD not only affects the lungs, but also causes multiple organ damage, including skeletal muscle dysfunction (SMD), and cardiovascular, nervous and digestive system dysfunction.
COPD patients mainly experience exercise limitations due to impaired lung function. However, in recent years, more and more studies have shown that about 40% of COPD patients with exercise limitations do not have severely impaired pulmonary function, but do present with significant SMD (2). Functionally, the SMD of COPD patients is characterized by insufficient muscle strength and reduced muscle endurance. Structurally, reduced muscle and a reduced cross-sectional area (muscle atrophy) have been observed (3). The skeletal muscle of COPD patients is characterized by changes in fiber type distribution, a decreased proportion of type I fibers, an increased proportion of oxidative and glycolytic fibers, changes in oxidative metabolic capacity (the attenuation of mitochondrial enzyme activity and expression), and capillary distribution oxygen modification. Skeletal muscle atrophy in COPD patients is correlated with diminished respiratory function, decreased activity tolerance, sub-health status, and increased mortality (4). Its mechanism is a combination of multiple molecular biological factors that are not yet fully understood. At present, it is thought that mitochondrial abnormalities, a protein synthesis/breakdown imbalance, muscle structural changes, muscle atrophy/waste, malnutrition/wasting, oxidative stress, and hypoxia, and hypercapnia contribute to SMD in COPD (4).
In previous study, our group confirmed that quadriceps mass and the cross-sectional area of muscle fibers are reduced, the proportion of type I fibers is decreased, while type IIa and IIx fibers are increased in COPD rats (5). The expression of myostatin is significantly increased in the quadriceps femoris of COPD rats, which is negatively correlated with quadriceps femoris weight, the quadriceps femoris cross-sectional area, and MyHC-I protein, and positively correlated with MyHC-IIa and MyHC-IIx protein expression.
Myostatin, also known as growth and differentiation factor 8 (GDF-8), is a secreted growth factor. It is a key member of the transforming growth factor-β superfamily, and is mainly expressed in skeletal muscle. Myostatin is an important regulator of body fat content and skeletal muscle growth in animals. It negatively regulates muscle development. Myostatin knockout mice have about 30% increased body weight and 2–3 times higher skeletal muscle weight than wild-type mice. Additionally, their number of muscle fibers is 86% higher than that of wild-type mice (6). Further, the overexpression of myostatin in vivo cause mice to develop manifestations similar to human cachexia.
In adult skeletal muscles, the maintenance of myostatin expression leads to proliferation inhibition, hypertrophy, and even muscle atrophy and consumption (7). Study has shown that chronic diseases, such as cancer, HIV infection, and liver disease, and muscle attenuation due to aging are all closely related to high expression levels of myostatin (8). Kneppers et al. found a significant increase in myostatin expression in the quadriceps muscle of COPD patients (9). Zhou et al. found a significant increase in myostatin expression and the apoptosis index in the diaphragm of COPD rats (10). In the experiments set up by our group, we observed upregulated myostatin expression in the quadriceps femoris tissue of COPD rats, which was negatively correlated with quadriceps weight, the cross-sectional area, and the endurance running distance of animals. However, the mechanism by which myostatin is involved in quadriceps dysfunction in COPD is not clear.
One mechanism of SMD in COPD is mitochondrial abnormalities. As semi-autonomous organelles, mitochondria are not only involved in metabolic processes but also play a role in regulating cellular responses to dangers and injuries. The mitochondria are located at the hub of cellular metabolic flux, uniquely adapted to link to the nucleus, allowing cells to sense extracellular threats and respond to injury by releasing reactive oxygen species, changes in mitochondrial membrane polarity, and signaling of mitochondria-associated membranes and proteins (11). Due to the critical role mitochondria play in energy manufacturing, the normal structure and function of mitochondria are decisive factors in the survival of cells. In the preliminary experiment, the research group performed a proteomics analysis of the quadriceps femoris tissues of COPD rats and healthy control rats. The Gene Ontology (GO) enrichment analysis of the differential proteins revealed that GO functional classification was mainly related to the mitochondrial inner membrane, mitochondrial outer membrane, mitochondrial oxidative phosphorylation process, cell differentiation, cell cycle, and developmental process. Thus, we speculated that myostatin could be involved in the occurrence of COPD SMD by affecting mitochondrial function.
Mitochondrial dynamics include the division of mitochondria and mitochondrial fusion. The dynamic balance of the mitochondrial fusion/division movement can maintain the normal morphology and effective function of mitochondria, and contribute to mitochondrial homeostasis and adaptation to stress. Previous study (12) has suggested that mitochondrial fusion is beneficial, but that excessive mitochondrial fission is detrimental. As mitochondrial fusion is associated with increased mitochondrial function and adenosine-triphosphate (ATP) production, mitochondrial fission is associated with reduced mitochondrial function and increased reactive oxygen species (ROS) production, which participates in cell death pathways. Further, ROS-induced mitochondrial dysfunction can enhance ROS production, which in turn can create a vicious cycle and result in the persistent oxidative damage of mitochondria (13,14).
Dynamin-related protein 1 (Drp-1), which is mainly located in the cell matrix, is a key protein that initiates mitochondrial division. When it is activated, it can form a complex polymer with receptors and molecules on the mitochondrial membrane, including fission protein 1 (Fis1), mitochondrial fission factor (Mff), mitochondrial dynamics proteins of 49 kDa (MiD49), and mitochondrial dynamics proteins of 51 kDa (MiD51). Regulating GTPase activity in Drp-1 through a series of post-translational modifications could mediate the division process of mitochondria (15,16). When mitochondria divide, Drp-1 is transferred from the cytoplasm to the division site of the outer mitochondrial membrane, and by the end of the division, Drp-1 on the outer membrane returns to the cytoplasm. The reciprocal runs of Drp-1 ensure the normal progress of the mitochondrial division movement (17). Study has shown that in lung epithelial cells, cigarette smoke induces mitochondrial fragmentation, and accelerates mitophagy and necroptosis by increasing the expression of Drp-1 and reducing the expression of mitofusin-2 (18). Our group performed a protein analysis of the quadriceps femoris tissue of COPD and healthy rats, and found that Drp-1 expression in the quadriceps femoris cells of the COPD rats was significantly increased, which was similar to the trend observed in myostatin expression. Thus, myostatin may enhance mitochondrial division through Drp-1 overexpression.
Previous studies on COPD have mostly focused on the lung, and there are few studies on the damage of this disease to skeletal muscle. The study of skeletal muscle atrophy in COPD has mainly focused on clinical observational studies, with few relevant basic studies and even fewer studies on mitochondria-associated skeletal muscle atrophy in COPD. This study is mainly conducted at the cellular level, focusing on mitochondria. dynamin-related protein 1was introduced through previous studies to further connect the relationship between myostatin and mitochondrial dynamics. By observing mitochondrial morphology and dynamics in a cell model of COPD, one of the possible mechanisms of mitochondria in COPD skeletal muscle atrophy is preliminarily elucidated. Future studies can be further validated in animals. This study sought to explore the effects and potential mechanisms of myostatin on skeletal muscle atrophy, mitochondrial division, apoptosis, and the links between related processes in COPD. We found that cigarette smoke increases Drp-1 expression and enhances mitochondrial division via the upregulation of myostatin, which in turn promotes apoptosis and affects cellular energy metabolism, which leads to the development of SMD in COPD. This study also extends understandings of skeletal muscle function in COPD and provides a basis for using myostatin and Drp-1 as novel therapeutic targets for SMD in COPD.
We present the following article in accordance with the MDAR reporting checklist (available at https://atm.amegroups.com/article/view/10.21037/atm-22-377/rc).
Methods
Materials
Mouse C2C12 myoblast cell lines were obtained from the Shanghai Institute of Cell Biology, Chinese Academy of Sciences. Dulbecco’s Modified Eagle Medium, a high-glucose medium, and fetal bovine serum (FBS) were purchased from Gibco. The Mito-TrackerGreen kit and JC-1 kit were purchased from Beyotime. Total ribonucleic acid (RNA) extraction reagent TRIzol was purchased from Life Technologies. Antibodies against Drp-1 and myostatin were obtained from Cell Signaling Technology. The ROS assay kit was obtained from Beyotime, and the apoptosis detection kit was purchased from Becton, Dickinson, and Company. The reverse transcription kit and quantitative polymerase chain reaction (qPCR) kit were purchased from Accurate Biology.
Cell culture and cell transfection
The mouse C2C12 myoblast cell lines (purchased from Shanghai Institute of Cell Biology, Chinese Academy of Sciences) were cultured with high-glucose medium containing 10% FBS, and the cells were observed to be shuttle-shaped with a clear cytoplasm. The cells cultured to the fifth generation were seeded into 6-well plates. Cells in the logarithmic growth phase were used for the cell transfection. Gene fragments, such as negative control and myostatin small interfering RNA (siRNA), which were used for cell transfection, were synthesized by Accurate Biology. Cell transfection was performed according to the instructions of the test kit. Real-time PCR (RT-PCR) and Western blot were performed to detect gene expression in the transfected and un-transfected cells.
Cellular intervention
Cigarette smoke extracts (CSE) were prepared and purified before the experiment, 5% CSE was used for the cell interventions according to the results of previous studies (19). The cells were divided into the culture of blank control (CON) group, the myostatin siRNA (MS) group, the 5% CSE (CSE) group, and the myostatin siRNA + 5% CSE (MSC) group for 24 hours, and the intervened cells were stored for subsequent assays.
Mitochondrial division and mitochondrial membrane potential detection
Mito-Tracker Green and 5,5',6,6'-tetrachloro-1,1'3,3'-tetrathylbenzimidazolyl-carbocyanine iodide (JC-1) staining (both from Beyotime) were performed according to the methods in the kits’ instructions, and observed under an inverted fluorescence microscope (Nikon TI-S).
Detection of ROS by flow cytometry
ROS was detected using a ROS Assay Kit (Beyotime). 2,7-Dichlorodihydrofluorescein diacetate (DCFH-DA) probes were loaded in situ, and cell stimulation was performed according to the instructions of kit. Next, the cells were collected and detected by flow cytometry, using an excitation wavelength of 488 nm and an emission wavelength of 525 nm to detect changes in the ROS in each group of cells.
Apoptosis by flow cytometry
The apoptotic cells were detected using the Anncxin V-FiTC/PI kit (BD). The cells were processed as follows: after trypsin digestion and resuspension in Phosphate Buffered Saline (PBS), AnnexinV-FITC (5 μL) and Propidium iodide (PI) (5 μL) was successively added to 1×105 cells from each group, and the cells were then incubated for 15 min in the dark. The flow cytometer (Beckman CytoFLEX) was used at an excitation wavelength of 488 nm, with red fluorescence for PI and green fluorescence for Annexin V-FITC.
Detection of mRNA and protein expression
Real-time PCR and Western blot were used to examine the myostatin, Drp-1 mRNA and protein expression levels. Rabbit anti-monoclonal antibody (1:1,000), and Horseradish Peroxidase (HRP)-labeled mouse anti-rabbit IgG (1:3,000) were purchased from Cell Signaling Technology (CST). A reverse transcription kit and qPCR kit were purchased from Accurate Biology, and the primers were synthesized by Accurate Biology according to the study design (Table 1).
Table 1
Name | Primer sequence (5'-3') | Product (bp) |
---|---|---|
MSTN | F: GCTCAAACAGCCTGAATCCAAC | 161 |
R: CAAAGTCTCTCCGGGACCT | ||
Dnm1l | F: CAACAGGCAACTGGAGAGGAATG | 145 |
R: GCAACTGGAACTGGCACATCT | ||
GAPDH | F: GGCCTCCAAGGAGTAAGACC | 122 |
R: AGGGGAGATTCAGTGTGGTG |
RT-PCR, real-time polymerase chain reaction.
Mitochondrial electron microscopy
The intervened cells were digested with trypsin, collected by centrifugation at 3,000 rpm for 5 minutes, discarded, and fixed by the gentle addition of glutaraldehyde, and left to stand for 1 hour at room temperature, and then transferred to 4 °C for storage. Next, the mitochondria of the cells were observed under a transmission electron microscope in sections.
Statistical analyses
The data are presented as mean ± standard deviation. The differences between the 2 groups were analyzed by unpaired t-tests, and the differences between 3 or more groups were analyzed by a 1-way analysis of variance. Pearson correlation analyses were conducted. GraphPad Prism Version 9.0.0[86] was used for multiple comparison testing. Three experiments were performed on a sample for technical replication, and one experiment was performed on different cells from three generations for biological replication. Mean values were obtained from 3 independent experiments. A P value <0.05 was considered significant.
Results
The expression of myostatin and Drp-1 was consistent under CSE stimulation
To determine whether myostatin is involved in the development of SMD in COPD via the regulation of Drp-1 expression, myostatin siRNA-transfected skeletal muscle cells were cultured and intervened using CSE, and Western blot and PCR were performed to observe whether the expression of myostatin and Drp-1 in the skeletal muscle cells stimulated by CSE was consistent. The results showed that there was a correlation between the expression of both at the level of protein and mRNA (Figures 1,2).
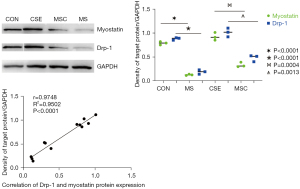
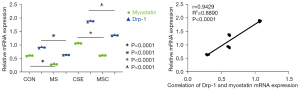
CSE caused excessive mitochondrial division, decreased cell viability, and increased apoptosis; however, reduced the expression of myostatin could reduce these changes
To investigate the effect of cigarette smoke on mitochondrial division, CSE were fabricated according to previous research methods (19), and the skeletal muscle cells were exposed to different concentrations of CSE. According to the response of the skeletal muscle cells to the CSE concentrations in the preliminary experiment, massive cell death was observed under CSE stimulations at concentrations >10%. Thus, the experiment was performed at a concentration of 5% CSE. Compared to the myostatin siRNA-transfected cells, the un-transfected cells showed lower density under CSE stimulation, the long shuttle shape of the cells extending into typical skeletal muscle cells was reduced, and cell rounding and refraction were increased (Figure 3). The results of the cell transmission electron microscopy showed that the number of mitochondria in cells stimulated by CSE increased, but the morphology became short, the cristae decreased, and most of them showed fragmentation and vacuolization (Figure 4). The results of the flow cytometry suggested that CSE significantly increased ROS production and apoptosis in normal cells (Figures 5,6). The cells inhibited by myostatin were relatively resistant to CSE stimulation. The results of the green fluorescence staining of the mitochondria showed that cellular green fluorescence was significantly reduced, which indicated increased mitochondrial division and decreased cellular activity (Figure 7). The results of the JC-1 staining showed a significant shift from red fluorescence to green fluorescence, indicating a decrease in mitochondrial membrane potential and a lateral reflection of increased early apoptosis (Figure 8).
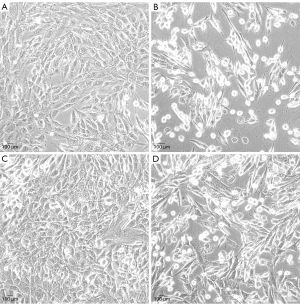
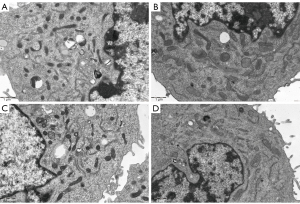
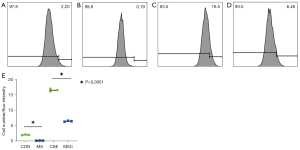
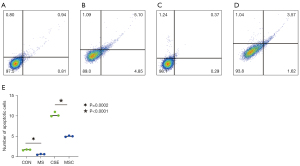
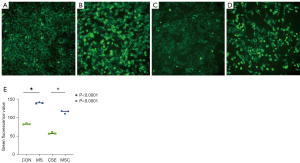
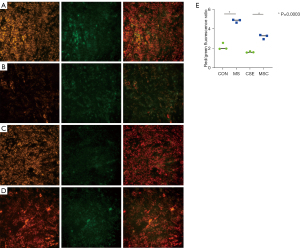
Discussion
The mitochondrion is the main hub of energy metabolism in a cell and plays a key role in various cell biological processes, including ATP production, Ca2+ homeostasis, ROS generation, and apoptosis. Mitochondria determine the normal physiological metabolic activity of cells by making energy via the direct uptake of oxygen in cells. Mitochondrial quality is an important prerequisite for normal cell growth and metabolism. Mitochondrial quality control includes altered morphology and energy production, amount of OXPHOS and mtROS production, mitochondrial membrane potential level, regulation of intracellular calcium flow, and activation/apoptosis of cell death mechanisms (20). Study have shown that cigarette smoke alters the structure of mitochondria, which leads to dysfunction. OPA1 is the major endo-mitochondrial gtp enzyme responsible for the fusion event and undergoes proteolytic cleavage from long to short forms during acute stress and mitophagy. Long OPA1 isoforms as well as SLP2 and prohibitions cause mitophagy/mitochondrial dysfunction in COPD in CS-induced lung injury (21). Mitochondrial dysfunction is thought to be involved in skeletal muscle atrophy in COPD. Mitochondrial damage in COPD may affect the efficiency of energy conversion and respiratory chain complexes in vivo (22). It may be related to the decreased concentration of mitochondria related active enzymes or the decreased coupling efficiency of oxidation/acidification in mitochondria (23). Mitophagy, mitochondrial biogenesis, and mitochondrial dynamics (fission and fusion) are essential to maintain cellular homeostasis. Mitophagy plays a crucial role in the repair of mitochondrial damage and is controlled by the PTEN-induced putative protein kinase 1 (PINK1) parkin RBR E3 ubiquitin protein ligase (PRKN) pathway. Araya et al. showed that PRKN protein levels are rate-limiting for PINK1-PRKN-mediated mitophagy during CSE exposure (24). The process of mitochondrial dysfunction resulting from CSE is dependent on the stabilization of PINK1, suggesting that PRKN induction alleviates the progression of COPD (25). Mitochondrial dynamics include fusion and fission, the balance of which control the number, shape, and size of mitochondria.
Drp-1 is a major protein regulating mitochondrial fission and belongs to the dynamin family of GTPases. The transfer of Drp-1 from cytoplasm to the outer mitochondrial membrane led to mitochondrial fission (26). The balance of mitochondrial dynamics plays a crucial role in maintaining mitochondrial morphology and function to meet the energy needs of skeletal muscle cells. However, it is not clear whether cigarette smoke affects mitochondrial dynamics in the skeletal muscle of COPD patients. Our results showed that the expression of Drp-1 was significantly increased in rat skeletal muscle cells after exposure to cigarette smoke in a concentration-dependent manner as measured by mRNA and protein levels. To determine the morphological changes of mitochondria by CSE, transmission electron microscopy was performed. The results revealed that the number of mitochondria increased, mitochondrial cristae decreased, vacuolated, and shorten or fragmented morphologically.
A randomized controlled study has found that high-intensity training can reduce the levels of serum myostatin, increase muscle mass, improve exercise capacity, and improve the quality of life of COPD patients (27). However, there are more intriguing questions that still need to be answered, such as how is myostatin involved in SMD in COPD, and does enhance mitochondrial division mediated by Drp-1 lead to negative outcomes in skeletal muscle atrophy? We performed protein analyses of quadriceps femoris muscle tissues from COPD rats and healthy control rats, and found that Drp-1 expression and myostatin expression in COPD rats were significantly increased. After deficient myostatin expression in rat skeletal muscle cells by siRNA-transfection, a consistent trend of Drp-1 and myostatin expression was detected via the Western blot and PCR. The results indicated that myostatin knockdown cells also reduced Drp-1 expression. Further, the myostatin knockdown cells were better able to tolerate the CSE intervention, showed less ROS generation, had a lower apoptosis rate, and had relatively higher mitochondrial membrane potential. Thus, it can be speculated that the CSE intervention induced myostatin expression, led to the upregulation of Drp-1, and further enhanced mitochondrial division. In the end, a series of changes in cellular energy metabolism and apoptosis were caused.
A previous study has reported that mitochondrial fission is essential in apoptosis caused by different stimuli (28). Increased mitochondrial division produces excess ROS, which participate in cell death pathways, induce oxidative stress, and impair mitochondrial function, which in turn further enhance ROS production, leading to a vicious cycle. This is consistent with the findings of Zhang et al., (29) who found that under the supply of high glucose, insulinoma cell mitochondrial division was increased and fusion was decreased, and inhibiting Drp-1 led to reduced excessive mitochondrial division and decreased ROS generation. As apoptotic cells have a higher fission fusion rate, fission has been considered an essential prerequisite for apoptosis (30). Drp-1-deficient mice can resist apoptosis, which suggests that Drp-1 plays a key role in apoptosis (31). The movement of Drp-1 from the cytoplasm to the outer mitochondrial membrane increases mitochondrial division and induces mitochondrial fragmentation, which leads to increased apoptosis (32,33).
There is accumulating evidence that mitochondrial fragmentation is a hallmark of several diseases associated with increased apoptosis (34,35). We found that murine skeletal muscle cells exposed to CSE had increased mitochondrial fission and ROS generation, higher levels of mitochondrial division, lower membrane potential, and increased apoptosis. Thus, an imbalance in mitochondrial dynamics (and increased mitochondrial fission) may lead to an imbalance in cellular energy metabolism, which in turn may lead to increased apoptosis.
In summary, our findings suggest that cigarette smoke may perturb the stabilization of mitochondrial dynamics by promoting the expression of myostatin, which in turn leads to the upregulation of Drp-1 expression, which leads to enhanced mitochondrial division in patients with skeletal muscle atrophy in COPD. The increased mitochondrial division cause increased ROS production through negative feedback, which further promotes the mitochondrial division and apoptosis of the cells by affecting cellular energy metabolism. These results provide novel ideas of skeletal muscle disfunction in COPD, and reveal the potential of myostatin and Drp-1 as novel therapeutic targets to improve the impaired skeletal muscle function of COPD patients.
Acknowledgments
Funding: This work was supported by grants from the Guangzhou Science and Technology Plan Projects (grant No. 2018A0303130342), and the Guangdong Science and Technology Plan Projects (grant No. 2017A070701014).
Footnote
Reporting Checklist: The authors have completed the MDAR reporting checklist. Available at https://atm.amegroups.com/article/view/10.21037/atm-22-377/rc
Data Sharing Statement: Available at https://atm.amegroups.com/article/view/10.21037/atm-22-377/dss
Conflicts of Interest: All authors have completed the ICMJE uniform disclosure form (available at https://atm.amegroups.com/article/view/10.21037/atm-22-377/coif). The authors have no conflicts of interest to declare.
Ethical Statement: The authors are accountable for all aspects of the work in ensuring that questions related to the accuracy or integrity of any part of the work are appropriately investigated and resolved.
Open Access Statement: This is an Open Access article distributed in accordance with the Creative Commons Attribution-NonCommercial-NoDerivs 4.0 International License (CC BY-NC-ND 4.0), which permits the non-commercial replication and distribution of the article with the strict proviso that no changes or edits are made and the original work is properly cited (including links to both the formal publication through the relevant DOI and the license). See: https://creativecommons.org/licenses/by-nc-nd/4.0/.
References
- Wang C, Xu J, Yang L, et al. Prevalence and risk factors of chronic obstructive pulmonary disease in China (the China Pulmonary Health [CPH] study): a national cross-sectional study. Lancet 2018;391:1706-17. [Crossref] [PubMed]
- Chan SMH, Selemidis S, Bozinovski S, et al. Pathobiological mechanisms underlying metabolic syndrome (MetS) in chronic obstructive pulmonary disease (COPD): clinical significance and therapeutic strategies. Pharmacol Ther 2019;198:160-88. [Crossref] [PubMed]
- Jaitovich A, Barreiro E. Skeletal Muscle Dysfunction in Chronic Obstructive Pulmonary Disease. What We Know and Can Do for Our Patients. Am J Respir Crit Care Med 2018;198:175-86. [Crossref] [PubMed]
- Lee JE, Westrate LM, Wu H, et al. Multiple dynamin family members collaborate to drive mitochondrial division. Nature 2016;540:139-43. [Crossref] [PubMed]
- Yang S, Gao X, Wu J, et al. Oxidative damage of rat quadriceps femoris cells induced by cigarette smoke extract. International Journal of Respiration 2014;1005-9.
- Willis-Owen SAG, Thompson A, Kemp PR, et al. COPD is accompanied by co-ordinated transcriptional perturbation in the quadriceps affecting the mitochondria and extracellular matrix. Sci Rep 2018;8:12165. [Crossref] [PubMed]
- Harish P, Malerba A, Lu-Nguyen N, et al. Inhibition of myostatin improves muscle atrophy in oculopharyngeal muscular dystrophy (OPMD). J Cachexia Sarcopenia Muscle 2019;10:1016-26. [Crossref] [PubMed]
- Su CM, Hu SL, Sun Y, et al. Myostatin induces tumor necrosis factor-α expression in rheumatoid arthritis synovial fibroblasts through the PI3K-Akt signaling pathway. J Cell Physiol 2019;234:9793-801. [Crossref] [PubMed]
- Kneppers AEM, Langen RCJ, Gosker HR, et al. Increased Myogenic and Protein Turnover Signaling in Skeletal Muscle of Chronic Obstructive Pulmonary Disease Patients With Sarcopenia. J Am Med Dir Assoc 2017;18:637.e1-e11. [Crossref] [PubMed]
- Zhou X, Yi D, Wu Y, et al. Expression of diaphragmatic myostatin and correlation with apoptosis in rats with chronic obstructive pulmonary disease. Exp Ther Med 2018;15:2295-300. [Crossref] [PubMed]
- Naviaux RK. Metabolic features of the cell danger response. Mitochondrion 2014;16:7-17. [Crossref] [PubMed]
- Ding M, Feng N, Tang D, et al. Melatonin prevents Drp-1-mediated mitochondrial fission in diabetic hearts through SIRT1-PGC1α pathway. J Pineal Res 2018;65:e12491. [Crossref] [PubMed]
- Kang L, Liu S, Li J, et al. The mitochondria-targeted anti-oxidant MitoQ protects against intervertebral disc degeneration by ameliorating mitochondrial dysfunction and redox imbalance. Cell Prolif 2020;53:e12779. [Crossref] [PubMed]
- Suzuki S, Fujita N, Hosogane N, et al. Excessive reactive oxygen species are therapeutic targets for intervertebral disc degeneration. Arthritis Res Ther 2015;17:316. [Crossref] [PubMed]
- Nishikawa H, Enomoto H, Ishii A, et al. Elevated serum myostatin level is associated with worse survival in patients with liver cirrhosis. J Cachexia Sarcopenia Muscle 2017;8:915-25. [Crossref] [PubMed]
- Kong X, Yao T, Zhou P, et al. Brown Adipose Tissue Controls Skeletal Muscle Function via the Secretion of Myostatin. Cell Metab 2018;28:631-643.e3. [Crossref] [PubMed]
- Lessard SJ, MacDonald TL, Pathak P, et al. JNK regulates muscle remodeling via myostatin/SMAD inhibition. Nat Commun 2018;9:3030. [Crossref] [PubMed]
- Aravamudan B, Kiel A, Freeman M, et al. Cigarette smoke-induced mitochondrial fragmentation and dysfunction in human airway smooth muscle. Am J Physiol Lung Cell Mol Physiol 2014;306:L840-54. [Crossref] [PubMed]
- Tan Z, Li S, Zhu S, et al. Effect of cigarette smoke extract on mitochondrial division in mouse quadriceps femoris cells. Ann Transl Med 2021;9:1699. [Crossref] [PubMed]
- Larson-Casey JL, He C, Carter AB. Mitochondrial quality control in pulmonary fibrosis. Redox Biol 2020;33:101426. [Crossref] [PubMed]
- Maremanda KP, Sundar IK, Rahman I. Role of inner mitochondrial protein OPA1 in mitochondrial dysfunction by tobacco smoking and in the pathogenesis of COPD. Redox Biol. 2021;45:102055. [Crossref] [PubMed]
- Prakash YS, Pabelick CM, Sieck GC. Mitochondrial Dysfunction in Airway Disease. Chest 2017;152:618-26. [Crossref] [PubMed]
- Fonseca TB, Sánchez-Guerrero Á, Milosevic I, et al. Mitochondrial fission requires DRP1 but not dynamins. Nature 2019;570:E34-42. [Crossref] [PubMed]
- Araya J, Tsubouchi K, Sato N, et al. PRKN-regulated mitophagy and cellular senescence during COPD pathogenesis. Autophagy 2019;15:510-26. [Crossref] [PubMed]
- Mizumura K, Cloonan SM, Nakahira K, et al. Mitophagy-dependent necroptosis contributes to the pathogenesis of COPD. J Clin Invest 2014;124:3987-4003. [Crossref] [PubMed]
- Detmer SA, Chan DC. Functions and dysfunctions of mitochondrial dynamics. Nat Rev Mol Cell Biol 2007;8:870-9. [Crossref] [PubMed]
- Boeselt T, Nell C, Lütteken L, et al. Benefits of High-Intensity Exercise Training to Patients with Chronic Obstructive Pulmonary Disease: A Controlled Study. Respiration 2017;93:301-10. [Crossref] [PubMed]
- Xie LL, Shi F, Tan Z, et al. Mitochondrial network structure homeostasis and cell death. Cancer Sci 2018;109:3686-94. [Crossref] [PubMed]
- Zhang Q, Hu C, Huang J, et al. ROCK1 induces dopaminergic nerve cell apoptosis via the activation of Drp1-mediated aberrant mitochondrial fission in Parkinson's disease. Exp Mol Med 2019;51:1-13. [Crossref] [PubMed]
- Karbowski M, Lee YJ, Gaume B, et al. Spatial and temporal association of Bax with mitochondrial fission sites, Drp1, and Mfn2 during apoptosis. J Cell Biol 2002;159:931-8. [Crossref] [PubMed]
- Ishihara N, Nomura M, Jofuku A, et al. Mitochondrial fission factor Drp1 is essential for embryonic development and synapse formation in mice. Nat Cell Biol 2009;11:958-66. [Crossref] [PubMed]
- Cereghetti GM, Costa V, Scorrano L. Inhibition of Drp1-dependent mitochondrial fragmentation and apoptosis by a polypeptide antagonist of calcineurin. Cell Death Differ 2010;17:1785-94. [Crossref] [PubMed]
- Cereghetti GM, Stangherlin A, Martins de Brito O, et al. Dephosphorylation by calcineurin regulates translocation of Drp1 to mitochondria. Proc Natl Acad Sci U S A 2008;105:15803-8. [Crossref] [PubMed]
- Wang X, Su B, Lee HG, et al. Impaired balance of mitochondrial fission and fusion in Alzheimer's disease. J Neurosci 2009;29:9090-103. [Crossref] [PubMed]
- Lutz AK, Exner N, Fett ME, et al. Loss of parkin or PINK1 function increases Drp1-dependent mitochondrial fragmentation. J Biol Chem 2009;284:22938-51. [Crossref] [PubMed]
(English Language Editor: L. Huleatt)