Potential mechanisms of Qili Qiangxin capsule to prevent pulmonary arterial hypertension based on network pharmacology analysis in a rat model
Introduction
Pulmonary arterial hypertension (PAH) is a complex and lethal disorder characterized by remodeling of smaller pulmonary arteries and vascular proliferation that leads to right ventricular failure or death (1). At present, the first-line treatments for PAH mainly include endothelin receptor antagonist, the phosphodiesterase type-5 inhibitors, soluble guanylate cyclase agonist, calcium antagonist, prostacyclin receptor agonist. However, some western medications have been reported to be associated with numerous side effects and adverse reactions (2). Therefore, more feasible and safer therapeutic strategies are required for PAH management. In recent years, several exploratory studies demonstrated that THM, particularly some active components derived from THM (i.e., quercetin, baicalein, ursolic acid and berberine), could be used as therapeutic candidates to prevent or cure PAH (3-6).
Qili Qiangxin capsule (QQC) is a well-known traditional Chinese medicine formulation comprising 11 herbs that is commonly used to treat heart failure (7,8). Nonetheless, its precise targets remain unclear. It is thought to work through a variety of mechanisms, including decreasing the remodeling of cardiac fibrosis, enhancing the function of the heart, and modulating inflammation (9,10).
In view of this, the objective of the present research was to identify the fundamental active constituents of QQC and possible pharmacological pathways that contribute to its anti-pulmonary hypertension functions. In addition, in vivo experiments were carried out to validate the results in this article. Meanwhile it is also the first time that network pharmacology and experimental validation were conducted to explore the mechanism of QQC on PAH therapy. Figure 1 depicts the overall design of the present research and its workflow. We present the following article in accordance with the ARRIVE reporting checklist (available at https://atm.amegroups.com/article/view/10.21037/atm-22-901/rc)
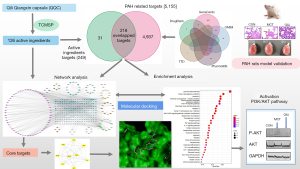
Methods
Identification of active ingredients and target proteins
The active ingredients of QQC were determined by using the Traditional Chinese Medicine Systems Pharmacology (TCMSP) database and analysis platform (11). The rate and degree of absorption of medicine in the human bloodstream are referred to as oral bioavailability (OB). Drug-like (DL) features describe the properties of a drug with a specified functional group or physical characteristics that are the same or comparable. To evaluate the transportation and absorption of drugs within the intestinal epithelial cells, the human intestinal cell line Caco-2 was used. The half-life of a medication is an essential measure for determining the dosage interval, the dosage that patients receive, and drug buildup since it represents the proportion of drugs in the bloodstream or the body (12). To identify those ingredients with the highest activity were subsequently tested if their OB was greater than 30% and their DL features were greater than 0.18. The SMILE configurations of the components were also acquired from the PubChem database.
Possible active ingredients targets and PAH
The ingredient-related targets were estimated with the aid of the TCMSP database. On 30 May 2021, five commonly used databases, namely the PharmGKB, GeneCards, Online Mendelian Inheritance in Man (OMIM), Therapeutic Target Database (TTD), and DrugBank database were used to determine the known PAH treatment targets. Several ingredient-related targets were identified as genes associated with PAH after duplicate entries were eliminated. After an intersection of QQC and PAH targets was found, the possible targets associated with both active components and PAH were identified. The study was conducted in accordance with the Declaration of Helsinki (as revised in 2013).
Protein-protein interaction (PPI)
As described in a previous study, a PPI analysis was carried out to search for important protein targets exhibiting therapeutic benefits against PAH (13). A database called STRING (https://cn.string-db.org/, downloaded on 30 May 2021) was used to investigate possible targets associated with both components and PAH. Four kinds of confidence ratings were used to assess PPI, namely low (0.1), medium (0.4), high (0.7), and highest (0.9). To facilitate subsequent investigations, prospective therapeutic targets exhibiting scores ≥0.9 were chosen.
Establishment and analysis of a network
Using the Cytoscape 3.8 program, a network of ingredient-target relationships was created to investigate the interaction between components and their possible therapeutic targets. In order to determine the relevance of nodes within the network, several of these analytic variables including degree were determined with the aid of the Network-Analyzer software. In the case where a node’s extent value exceeds twice the mean level of all nodes, it is considered to be an essential element of the network.
Enrichment analysis on possible treatment target
All possible treatment targets were input into the DAVID system (https://david.ncifcrf.gov/, v6.8), and we subsequently performed online Gene Ontology (GO) term enrichment and the Kyoto Encyclopedia of Genes and Genomes (KEGG) enrichment analysis to delve into their biological activities. Moreover, according to the gene ratio and the P value, the predominant signaling pathways and critical proteins involved in biological processes were determined.
Validation of essential active compounds and target interactions using molecular docking
Molecular docking analysis of the predominant active components of QQC and possible treatment targets was performed with the SYBYL-X software (version: 2.1.1; Tripos Company, USA) to measure the accuracy of the GO and KEGG enrichment analyses. X-ray crystallography was used to distinguish the crystal protein structures, which were then uploaded into SYBYL-X 2.1.1 and docked with the active compounds of QQC. To assess the outcomes, the docking metric DockScore was used.
Animal model and ethics approval
In the present research, a rat model was used (14). Animals were procured from the SLAC Experimental Animal Center in Shanghai, including 8-week-old Sprague Dawley males measuring 230–250 grams. The ethics committee of Xinhua Hospital affiliated to Shanghai Jiaotong University School of Medicine (Shanghai, China) granted approval for the experiment procedure (No. 81603099). Moreover, all procedures were performed in strict accordance with the National Institutes of Health Guidelines for the Care and Use of Experimental Animals.
In an ecologically closed chamber with a temperature ranging between 20–26 ℃ and a humidity range of 50%±5%, all rats were subjected to a 12-hour darkness/light cycle, and they had an unrestricted supply of water and food. Following 1 week of acclimatization, 24 rats were classified into three groups using a random classification: (I) the control group (n=8) was treated with isotonic sodium chloride solution; (II) the monocrotaline (MCT) group (n=8) was treated with MCT at 60 mg/kg; and (III) the QiLi group (n=8) was treated with MCT + QQC at 1 g/kg/day. The PAH model was induced as described previously (14). The control group rats received a subcutaneous injection of isotonic sodium chloride solution on day 1, followed by 4 weeks of water. The MCT group and QiLi group rats were injected subcutaneously with MCT (60 mg/kg) in the ventral thorax on day 1; based on previously reported method, drug powder was dissolved in sterile water at a concentration of 0.1 g/mL for QiLi group, and then, rats in QiLi group were administered by gavage (1 g/kg) once a day during the 4 weeks (15).
Hematoxylin and eosin (H&E) staining and western blotting
The rats were weighed and anesthetized intraperitoneally with sodium pentobarbital (30 mg/kg), followed by euthanasia by an intraperitoneally injection of sodium pentobarbital at a dosage of 150 mg/kg. The left lung tissues were sliced for histological and immunohistochemistry investigations, and the right lung tissues were frozen at −80℃ for western blot assay. Paraformaldehyde (4%) was used to fix the left lungs that had been isolated at room temperature for 48 hours before paraffin embedding and slicing of the tissues at a thickness of 4 µm. The lung tissue slices were subjected to staining with H&E in accordance with the instructions provided by the manufacturer. Microscopy was used to investigate the pulmonary arteries’ structural remodeling. In the present study, proteins were isolated from lung tissues by homogenizing them in lysis buffer in combination with phosphatase blockers (Cell Signaling Technology, Inc., Danvers, MA, USA) and enzyme protease (Beyotime Institute of Biotechnology, Haimen, China). The BCA Protein Assay kit was used to assess the percentage of protein in the sample. A comparable quantity (50 g) of protein lysate was isolated using 10% SDS-PAGE and subsequently loaded onto a polyvinylidene difluoride membrane for further analysis and characterization. In addition, blocking of the membranes was conducted for 2 hours at ambient temperature using 5% bovine serum albumin, followed by incubation with anti-GAPDH (1:1,000; Cell Signaling Technology 5174), anti-p-AKT473 antibody (1:1,000; Cell Signaling Technology 4060S), and anti-AKT antibody (1:1,000; Cell Signaling Technology 9272S) throughout the night at 4 ℃. Furthermore, TBST was used to wash the membranes, followed by incubation with IDye800CW conjugated secondary antibody (1:10,000; Cell Signaling Technology 5151) for 1 hour at ambient temperature. Protein bands were captured by the Odyssey imaging system (LICOR).
Statistical analysis
We used GraphPad Prism 5 software (GraphPad Software, Inc., LA Jolla, CA, USA) to perform statistical analyses on the obtained data. The data were expressed as the mean ± standard error of the mean. Results were considered statistically significant if P<0.05.
Results
Screening for bioactive components and QQC targets relevant to PAH therapy
First, the TCMSP database was used to screen 154 active compounds. Specifically, 126 active compounds were obtained as essential components as their OB and DL values were above the average. An additional study was conducted on 249 targets associated with the 126 active compounds. In addition, 5,155 targets for PAH treatment were obtained from TCMSP, DrugBank, OMIM, TTD, and PharmGKB (Figure 1). Using a Venn diagram to map QQC- and PAH-related targets (Figure 2A,2B), we identified 218 intersecting targets and their associated bioactive components. Moreover, Cytoscape was used to visualize 126 bioactive ingredients and 218 intersecting targets, which showed the compounds in QQC that were targets for treating PAH (Figure 2C,2D).
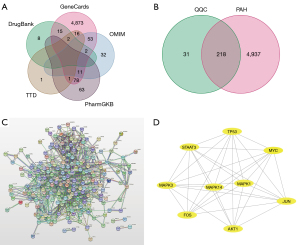
Establishment of a PPI network and identification of core targets
To investigate the treatment mechanism of QQC for PAH, a PPI network was established by inputting 218 intersecting targets into the STRING platform. The average node degree of the target network comprising 218 nodes as well as 61 edges was 8.64. In Cytoscape, the PPI network data generated in the STRING database was loaded, and the highest nine core targets were identified using the cytoHubba plug-in in decreasing order of degree (Figure 3). AKT1, JUN, MAPK1, and TP53 received the maximum possible ratings, therefore results showed that these may perform critical functions in the treatment of PAH.
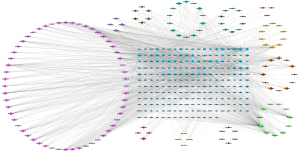
Enrichment analysis of GO and KEGG
The key biological activities and pathways were identified with GO and KEGG enrichment studies carried out using the R software. To treat PAH, findings showed that the functions of QQC were correlated with the reaction to oxidative stress, the responsiveness to medications, and the cellular response to chemical stress. Figure 4A shows a list of sample GO terms in a graphical format. The KEGG pathway enrichment analysis in the present research focused on atherosclerosis and lipid pathways, as well as the AGE-RAGE signaling pathway in hepatitis B and diabetes-related complications, all of which are depicted in Figure 4B.
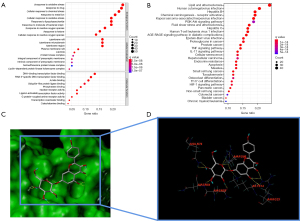
Molecular docking
Using SYBYL-X 2.1.1 software, a total of 26 molecule-target pairings were identified and verified using molecular docking. Consequently, 6 molecule-target pairs, which were quercetin/MAPK1, quercetin/JUN, luteolin/MAPK1, kaempferol/JUN, baicalein/TP53, and kaempferol/AKT1, demonstrated a strong binding association, as presented in Table 1. Figure 4C,4D depicts 3D molecule docking images of kaempferol/AKT pairs.
Table 1
Molecular name | Targets | Protein data bank ID | Docking score (kcal/mol) |
---|---|---|---|
Quercetin | MAPK1 | 6SLG | 6.1728 |
Quercetin | JUN | 6Y3V | 5.6552 |
Luteolin | MAPK1 | 6SLG | 5.628 |
Kaempferol | JUN | 6Y3V | 5.3342 |
Baicalein | TP53 | 3LW1 | 5.2171 |
Kaempferol | AKT1 | 1UNQ | 5.521 |
Histological evaluation and western blotting
We successfully established a rat model of PAH by injecting MCT subcutaneously. QQC was shown to be effective in suppressing MCT-induced pulmonary vascular remodeling. In the present research, we used H&E staining to quantify the small pulmonary arteries to assess pulmonary vascular remodeling. Following MCT therapy, the indicators of wall thickness (WT) of pulmonary arterioles in the MCT group were considerably higher as opposed to the ones of the control group (P<0.01). QQC (1 g/kg) treatment substantially suppressed these pathological alternations in the lungs as opposed to the MCT treatment (Figure 5). We identified several genes that may interact with QQC through. Moreover, we investigated whether QQC had an effect on the PI3K/AKT signaling pathway. The PI3K/AKT pathway is an essential proliferation-related signaling pathway. In this regard, we investigated the expression of AKT, which is a protein that performs a critical function in the PI3K/AKT signaling pathway. MCT substantially elevated the p-AKT phosphorylation levels of AKT in lung tissues in contrast with the control group (P<0.01). After the administration of QQC, the elevated phosphorylation of AKT was dramatically attenuated (P<0.01). Overall, QQC substantially attenuated the expression of phosphorylated AKT as well as the proportion of p-AKT/AKT induced by MCT (P<0.01).
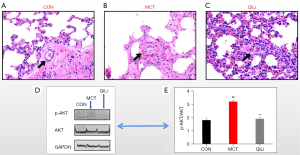
Discussion
Heart failure is highly prevalent in patients with PAH, especially those who have had the disease for a long time. A growing body of research shows that heart failure patients might benefit from the application of QQC in combination with conventional Western treatment, which has the potential to enhance treatment effectiveness and improve the quality of life of patients (16). Nevertheless, the material foundation of QQC and the mechanisms through which it can treat PAH are still being investigated.
Traditional Chinese medicine prescriptions are a complicated system that involves a broad variety of molecular targets and mechanisms. This makes it difficult to elucidate their effective constituents, therapeutic targets, and pharmacological mechanisms (17). Network pharmacology is an emerging discipline based on the theory of systems biology, which can accurately decipher the associations among the drugs, targets, and diseases at a systematic and comprehensive level (18). Increasing evidence supports the reliability of the network pharmacology method, which may be an effective way to study the pharmacological mechanisms of Traditional Chinese medicine (19).
In this study,Luteolin, kaempferol, baicalein, formononetin, and quercetin networks within QQC all had greater degree values calculated by Cytoscape software as opposed to the other components, and these critical components were all shown to impact the four hub targets, which were MAPK1, JUN, TP53, and AKT1. Moreover, molecular docking analysis showed that these 5 essential components had the highest binding affinity, suggesting that they could perform an integral function that enables QQC to reduce pulmonary hypertension. Kaempferol can prevent and reverse ventricular fibrosis and cardiac dysfunction by suppressing the production of inflammatory apoptosis and cytokines, enhancing antioxidant transcription factors, and promoting the generation of nitric oxide from the vascular endothelium (20-22). Quercetin can alleviate PAH by inhibiting cardiac fibrosis and balancing cell proliferation and apoptosis (23-25). Luteolin can protect against lipopolysaccharide (LPS)-induced cardiomyocyte hypertrophy and autophagy in rat cardiomyocytes (26). In addition, baicalein and formononetin may prevent PAH.
The KEGG pathway enrichment analysis showed that a number of pathways were significantly correlated with the PAH pathological process. In general, the most significant pathways involved were atherosclerosis, PI3K/AKT, HIF-1, TNF, AGE-RAGE, and IL-17. A significant interaction between the PI3K/AKT signaling pathway and other proliferation-related signaling pathways was observed among these pathways (27). Furthermore, earlier research has shown that the progression of PAH is strongly correlated with abnormal transduction of the PI3K/AKT signaling pathway (28). Activation of the PI3K/AKT signaling pathway in hypoxic environments or in rats experiencing MCT-induced PAH results in the thickening of the pulmonary vascular medial wall, proliferation, and apoptosis of smooth muscle cells of the pulmonary artery in humans (29).
In recent years, network pharmacology analysis was applied in a few studies to explore single herbal components of Traditional Chinese medicine involved in the treatment of PAH. Chen et al. (30) reported that the anti-PAH effect of genistein may be closely related to peroxisome proliferator-activated receptor γ (PPARγ), apoptotic signaling pathway, and the nitric oxide synthesis process. The results of another research showed that the effect of resveratrol may be closely associated with targets such as AKT1, MAPK3, SIRT1 and SRC, as well as biological processes such as cell proliferation, inflammatory response, and redox balance (31). However, the above studies lacked experimental verification. Therefore, we decided to investigate the PI3K/AKT signaling pathway. We further illustrated that QQC reduced the relative expression of proteins for p-AKT/AKT in the local lung tissue of PAH rats in vitro. Finally, we observed that QQC ameliorated MCT-induced PAH, at least in part, by inhibiting the PI3K/AKT signaling pathways through its antiproliferative effects.
These findings indicated that five candidate compounds are a promising source of anti-PAH agents. Furthermore, molecular docking analysis suggested that the active compounds interacted strongly with the PI3K/AKT signaling proteins. This study is a preliminary attempt to explore the effects of active compounds on the PI3K/AKT pathway. The results of animal experiments were consistent with the results of network pharmacology mining, which verified the reliability of network pharmacology prediction and confirmed the accuracy of QQC in the treatment of PAH.
Conclusions
Our research findings indicated that QQC suppressed pulmonary arterial smooth muscle hyperplasia and lowered the level of expression of p-AKT/AKT proteins. However, QQC can act on multiple pathways and has multiple biological functions, such as immunomodulation, anti-hypoxia, and anti-inflammation. Hence, more well-designed animal research, in vitro test models, and human trials are necessary to better reveal the mechanisms by which QQC can treat heart disease.
Acknowledgments
Funding: None.
Footnote
Reporting Checklist: The authors have completed the ARRIVE reporting checklist. Available at https://atm.amegroups.com/article/view/10.21037/atm-22-901/rc
Data Sharing Statement: Available at https://atm.amegroups.com/article/view/10.21037/atm-22-901/dss
Conflicts of Interest: All authors have completed the ICMJE uniform disclosure form (available at https://atm.amegroups.com/article/view/10.21037/atm-22-901/coif). The authors have no conflicts of interest to declare.
Ethical Statement: The authors are accountable for all aspects of the work in ensuring that questions related to the accuracy or integrity of any part of the work are appropriately investigated and resolved. The study was conducted in accordance with the Declaration of Helsinki (as revised in 2013). The ethics committee of Xinhua Hospital affiliated to Shanghai Jiaotong University School of Medicine (Shanghai, China) granted approval for the experiment procedure (No. 81603099). Moreover, all procedures were performed in strict accordance with the National Institutes of Health Guidelines for the Care and Use of Experimental Animals.
Open Access Statement: This is an Open Access article distributed in accordance with the Creative Commons Attribution-NonCommercial-NoDerivs 4.0 International License (CC BY-NC-ND 4.0), which permits the non-commercial replication and distribution of the article with the strict proviso that no changes or edits are made and the original work is properly cited (including links to both the formal publication through the relevant DOI and the license). See: https://creativecommons.org/licenses/by-nc-nd/4.0/.
References
- Rabinovitch M. Molecular pathogenesis of pulmonary arterial hypertension. J Clin Invest 2012;122:4306-13. [Crossref] [PubMed]
- Condon DF, Nickel NP, Anderson R, et al. The 6th World Symposium on Pulmonary Hypertension: what's old is new. F1000Res 2019;8:eF1000 Faculty Rev-888.
- He Y, Cao X, Guo P, et al. Quercetin induces autophagy via FOXO1-dependent pathways and autophagy suppression enhances quercetin-induced apoptosis in PASMCs in hypoxia. Free Radic Biol Med 2017;103:165-76. [Crossref] [PubMed]
- Hsu WL, Lin YC, Jeng JR, et al. Baicalein Ameliorates Pulmonary Arterial Hypertension Caused by Monocrotaline through Downregulation of ET-1 and ETAR in Pneumonectomized Rats. Am J Chin Med 2018;46:769-83. [Crossref] [PubMed]
- Gao X, Zhang Z, Li X, et al. Ursolic Acid Improves Monocrotaline-Induced Right Ventricular Remodeling by Regulating Metabolism. J Cardiovasc Pharmacol 2020;75:545-55. [Crossref] [PubMed]
- Wande Y, Jie L, Aikai Z, et al. Berberine alleviates pulmonary hypertension through Trx1 and β-catenin signaling pathways in pulmonary artery smooth muscle cells. Exp Cell Res 2020;390:111910. [Crossref] [PubMed]
- Chinese Pharmacopoeia Commission. Pharmacopoeia of the People’s Republic of China. Beijing: China Medical Science Press, 2020:1088.
- Duan X, Wu J, Huang X, et al. Comparative Efficacy of Chinese Herbal Injections for Treating Acute Exacerbation of Chronic Obstructive Pulmonary Disease: A Bayesian Network Meta-Analysis of Randomized Controlled Trials. Evid Based Complement Alternat Med 2018;2018:7942936. [Crossref] [PubMed]
- Tao L, Shen S, Li X. Future prospects of Qiliqiangxin on heart failure: epigenetic regulation of regeneration. Front Genet 2013;4:221. [Crossref] [PubMed]
- Zou Y, Lin L, Ye Y, et al. Qiliqiangxin inhibits the development of cardiac hypertrophy, remodeling, and dysfunction during 4 weeks of pressure overload in mice. J Cardiovasc Pharmacol 2012;59:268-80. [Crossref] [PubMed]
- Ru J, Li P, Wang J, et al. TCMSP: a database of systems pharmacology for drug discovery from herbal medicines. J Cheminform 2014;6:13. [Crossref] [PubMed]
- Zhang J, Huang Q, Zhao R, et al. A network pharmacology study on the Tripteryguim wilfordii Hook for treatment of Crohn's disease. BMC Complement Med Ther 2020;20:95. [Crossref] [PubMed]
- Franceschini A, Szklarczyk D, Frankild S, et al. STRING v9.1: protein-protein interaction networks, with increased coverage and integration. Nucleic Acids Res 2013;41:D808-15. [Crossref] [PubMed]
- Gomez-Arroyo JG, Farkas L, Alhussaini AA, et al. The monocrotaline model of pulmonary hypertension in perspective. Am J Physiol Lung Cell Mol Physiol 2012;302:L363-9. [Crossref] [PubMed]
- He Y, Du B, Fan H, et al. Beneficial Effects of Qili Qiangxin Capsule on Lung Structural Remodeling in Ischemic Heart Failure via TGF-β1/Smad3 Pathway. Evid Based Complement Alternat Med 2015;2015:298631. [Crossref] [PubMed]
- Xu X, Yang Y, Zhou G, et al. Clinical Efficacy of Qili Qiangxin Capsule Combined with Western Medicine in the Treatment of Chronic Heart Failure: A Systematic Review and Meta-Analysis. Evid Based Complement Alternat Med 2021;2021:9761159. [Crossref] [PubMed]
- Cai FF, Zhou WJ, Wu R, et al. Systems biology approaches in the study of Chinese herbal formulae. Chin Med 2018;13:65. [Crossref] [PubMed]
- Hopkins AL. Network pharmacology: the next paradigm in drug discovery. Nat Chem Biol 2008;4:682-90. [Crossref] [PubMed]
- Chen Z, Lin T, Liao X, et al. Network pharmacology based research into the effect and mechanism of Yinchenhao Decoction against Cholangiocarcinoma. Chin Med 2021;16:13. [Crossref] [PubMed]
- Liu Y, Gao L, Guo S, et al. Kaempferol Alleviates Angiotensin II-Induced Cardiac Dysfunction and Interstitial Fibrosis in Mice. Cell Physiol Biochem 2017;43:2253-63. [Crossref] [PubMed]
- Jin Y, Zhai Z, Jia H, et al. Kaempferol attenuates diquat-induced oxidative damage and apoptosis in intestinal porcine epithelial cells. Food Funct 2021;12:6889-99. [Crossref] [PubMed]
- Cui S, Tang J, Wang S, et al. RETRACTED: Kaempferol protects lipopolysaccharide-induced inflammatory injury in human aortic endothelial cells (HAECs) by regulation of miR-203. Biomed Pharmacother 2019;115:108888. Erratum in: Biomed Pharmacother 2022;146:112627. [Crossref] [PubMed]
- Wang L, Tan A, An X, et al. Quercetin Dihydrate inhibition of cardiac fibrosis induced by angiotensin II in vivo and in vitro. Biomed Pharmacother 2020;127:110205. [Crossref] [PubMed]
- Zhang W, Li Y, Luo Y, et al. Effects of quercetin on cytokines and pulmonary capillary cells in pulmonary arterial hypertension rats. Farmacia 2021;69:155-60. [Crossref]
- Li F, Li D, Tang S, et al. Quercetin Protects H9c2 Cardiomyocytes against Oxygen-Glucose Deprivation/Reoxygenation-Induced Oxidative Stress and Mitochondrial Apoptosis by Regulating the ERK1/2/DRP1 Signaling Pathway. Evid Based Complement Alternat Med 2021;2021:7522175. [Crossref] [PubMed]
- Li X, Liu J, Wang J, et al. Luteolin suppresses lipopolysaccharide-induced cardiomyocyte hypertrophy and autophagy in vitro. Mol Med Rep 2019;19:1551-60. [Crossref] [PubMed]
- Kudryashova TV, Goncharov DA, Pena A, et al. HIPPO-Integrin-linked Kinase Cross-Talk Controls Self-Sustaining Proliferation and Survival in Pulmonary Hypertension. Am J Respir Crit Care Med 2016;194:866-77. [Crossref] [PubMed]
- Archer SL, Weir EK, Wilkins MR. Basic science of pulmonary arterial hypertension for clinicians: new concepts and experimental therapies. Circulation 2010;121:2045-66. [Crossref] [PubMed]
- Zhang Q, Cao Y, Luo Q, et al. The transient receptor potential vanilloid-3 regulates hypoxia-mediated pulmonary artery smooth muscle cells proliferation via PI3K/AKT signaling pathway. Cell Prolif 2018;51:e12436. [Crossref] [PubMed]
- Chen Y, Chen D, Liu S, et al. Systematic Elucidation of the Mechanism of Genistein against Pulmonary Hypertension via Network Pharmacology Approach. Int J Mol Sci 2019;20:5569. [Crossref] [PubMed]
- Chen Y, Yuan T, Chen D, et al. Systematic analysis of molecular mechanism of resveratrol for treating pulmonary hypertension based on network pharmacology technology. Eur J Pharmacol 2020;888:173466. [Crossref] [PubMed]
(English Language Editor: C. Mullens)