EB1089 promotes the expression of vitamin D receptor in the intestinal epithelial cell line HT-29 and reduces lipopolysaccharide-induced inflammatory response
Introduction
Vitamin D receptor (VDR), one of the members of the steroid hormone receptor superfamily, is a ligand dependent transcription factor (1). After the active vitamin D3 form 1α,25(OH)2 D3 is combined with VDR, VDR becomes activated and begins to recruit many transcription factors, subsequently, it regulates the downstream gene transcription and expression (2). The synthetic novel 1,25-dihydroxy vitamin D3 analogue EB1089 has strong antiproliferative properties against a variety of malignant cells in vitro and in vivo. Its calcemic action is not as strong as 1,25-dihydroxyvitamin D3 [1,25(OH)2 D3], but its antiproliferative action is stronger. It has also been shown that EB1089 is also less effective than 1,25(OH)2 D3 in intestinal induction, but not the messenger RNA (mRNA) of renal calbindin-D9k (3,4). As a VDR agonist, EB1089 is more effective than vitamin D in anti-inflammatory function (5,6). The function of VDR is closely related to intestinal inflammation. The VDR knockout mice showed a stronger response to DSS, more severe colonic ulceration, and poorer wound healing than normal mice did (7). It has been reported that when VDR is activated, the expression of tight junction proteins in intestinal epithelial cells is up-regulated, and the permeability of intestinal epithelial cells is reduced, thereby minimizing the susceptibility of intestines to pathogens (8,9). We found that the expression of VDR and the number of CD4+ T cells in the peripheral blood of UC patients significantly decreased, while the expression of interleukin-4 (IL-4) significantly increased compared with that of healthy people, CD4+ T cells of VDR knockout mice easily differentiated into Th2 cells, and EB1089 promoted the transformation of CD4+ T cells to Treg cells in UC patients (10). It has been reported that VDR in CD4+ T cells could bind to NLRP3 inflammasome and reduce IL-4 expression (11). In macrophages induced from bone marrow, VDR binding to NLRP3 inhibits the deubiquitination of NLRP3 and promotes the ubiquitination degradation of inflammatory bodies; furthermore, vitamin D treatment decreases the expression of interleukin-1β (IL-1β) and mature caspase-1 in macrophages (12). Therefore, EB1089 may reduce intestinal inflammation through VDR.
In our study, we explored the anti-inflammatory effects of EB1089 on lipopolysaccharide (LPS)-induced inflammatory responses in the intestinal epithelial cell line HT-29 and the activation of NLRP3/caspase-1 and TLR4/NF-κB inflammatory pathways. This study could provide an experimental basis and theoretical support for using EB1089 in the treatment of UC and other intestinal inflammation-related diseases. We present the following article in accordance with the MDAR reporting checklist (available at https://atm.amegroups.com/article/view/10.21037/atm-22-1066/rc).
Methods
Cells
The intestinal epithelial cell line HT-29 (Purchased from Procell Life Science & Technology Co., Ltd., Wuhan, China). was cultured with McCoy’s 5A medium containing 10% fetal bovine serum (FBS) and 1% penicillin-streptomycin (P/S) at 37 ℃ with 5% CO2. The cells were divided into a control group, LPS group, and LPS + EB1089 group. In the LPS group, cells were treated with LPS (50 µg/mL) for 24 h. In the LPS + EB1089 group, cells were pretreated with EB1089 (0.27 nM) for 12 h followed by treatment with LPS (50 µg/mL) for 24 h. There was no treatment for the cells in the control group.
Cell Counting Kit-8 (CCK-8) detection
CCK-8 (Beyotime, Shanghai, China) was used to analyze cell viability. The cells were collected and incubated in a 96-well plate (5×103 cells/well) with a humidified incubator at 37 ℃ and 5% CO2 overnight. Then, add 10 µL of CCK-8 solution to each well. The optical density (OD)450 value was detected using an automatic microplate reader (WD-2102B, Beijing Liuyi Biotechnology Co., Ltd., Beijing, China). Cells from each group were detected with 6 repeated wells.
Cell apoptosis detection
Apoptosis was detected using an AnnexinV-PI Analysis Kit (Beyotime Biotechnology, Shanghai, China) in accordance with the manufacturer’s instructions. These cells were inoculated in 6-well plate. They were collected, digested, and washed 3 times with ice-cold phosphate-buffered saline (PBS). Then, cells at a density of 1×106 cells/mL were collected and resuspended in 300 µL 1× of binding buffer. Afterward, 195 µL Annexin V-FITC, 5 µL Annexin V-FITC, and 10 µL propidium iodide (PI) were added according to the protocol of the kit. Pre-cooled 1× binding buffer (200 µL) was added after 10 min of incubation in a refrigerator in the dark at 4 ℃. The apoptosis rate was detected by flow cytometry. The results were analyzed by CELLQUEST software [Becton, Dickinson, and Co., (BD) Biosciences, Franklin Lakes, NJ, USA]. All tests were repeated 3 times.
Enzyme-linked immunosorbent assay
The lactate dehydrogenase (LDH), interleukin 18 (IL-18), and IL-1β levels were detected using enzyme-linked immunosorbent assay (ELISA) kits in accordance with the instructions. The OD450 value was detected using an automatic microplate reader (WD-2102B, Beijing Liuyi Biotechnology Co., Ltd., China). Cells from each group were detected with 6 repeated wells. Calculate the linear regression equation of the standard curve according to the concentration and OD value of the reference material. Substitute the OD value of the sample into the equation to calculate the concentration of the sample, and then multiply it by the dilution ratio, that is, the actual concentration of the sample.
Western blot
The cells were lysed by Thermo Scientific RIPA buffer (89900, Thermo Fisher, Shanghai, China), centrifuged at 4 ℃ (1,000 rpm) for 5 min, and the supernatant was collected. After extraction of proteins, their concentration was measured with bicinchoninic acid (BCA). Proteins (50 µg/lane) were separated with 10% sodium dodecyl sulfate polyacrylamide gel electrophoresis (SDS-PAGE), then they were transferred to a polyvinylidene difluoride (PVDF) membrane (IPVH00010, Merck Millipore, Burlington, MA, USA). The PVDF membrane was washed and blocked by buffer. Primary antibodies (1:2,000 Anti-GAPDH, TA-08, ZSGB-Bio, Beijing, China; 1:100 Anti Caspase-1, 22915-1-ap, Proteintech Group, Inc., Rosemont, IL, USA; 1:500 Anti NF-κB, 15506-1-ap, Proteintech Group, Inc., USA; 1:1,000 Anti TLR4, 19811-1-ap, Proteintech Group, Inc., USA; 1:1,000 Anti VDR, 67192-1-1g, Proteintech Group, Inc., USA; 1:1,000 Anti ASC, df6304, Affinity Biosciences Ltd., Cincinnati, OH, USA; 1:1,000 Anti GSDMD, AF4012, Affinity Biosciences Ltd., USA; 1:500 Anti Myd88, bs-1047R, Beijing Bioss Biotechnology Co., Ltd., Beijing, China; 1:1,000 Anti NLRP3, bs-10021R, Beijing Bioss Biotechnology Co., Ltd, China) were added and they were incubated at 4 ℃ overnight. The membrane was rinsed and the secondary antibody horseradish peroxidase labeled Goat anti Rabbit IgG (H + L) (ZB-2301 ZSGB-Bio, China) was added, they were incubated at room temperature for 1 h. They were determined using an enhanced chemiluminescence kit (Perkin-Elmer Inc., Waltham, MA, USA). They were quantified with Quantity One software (Bio-Rad China, Beijing, China).
Statistical analyses
Statistical tests were conducted using SPSS 20.0 software (IBM Corp., Armonk, NY, USA). The differences between groups were analyzed by one-way analysis of variance (ANOVA) or Student’s t-test. The P value <0.05 was considered statistically significant.
Results
EB1089 pretreatment reduced LPS-induced apoptosis of HT-29 cells
As shown in Figure 1, the cell proliferation in the LPS and LPS + EB1089 groups was less than that in the control group, and the apoptosis rates in the LPS and LPS + EB1089 groups were higher than those in the control group (P<0.05). There was no significant difference in cell proliferation between LPS group and LPS + EB1089 group; however, the apoptosis rate in the LPS + EB1089 group was lower than that in the LPS group (P<0.05).
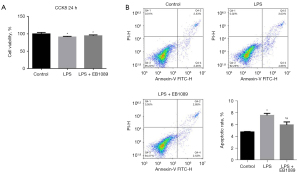
Pretreatment with EB1089 promoted VDR expression and inhibited LPS-induced inflammation in HT-29 cells
After LPS treatment, the ELISA results of LDH, IL-18, and IL-1β in the supernatant of HT-29 cells in each group are shown in Figure 2. The contents of IL-18 and IL-1β in the LPS and LPS + EB1089 groups were higher than that in the control group (P<0.05), but there was no significant difference between LPS group and LPS + EB1089 group. The LDH content in the LPS + EB1089 group was evidently lower than that in the LPS group (P<0.05).
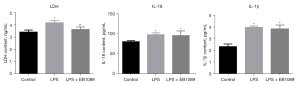
The protein expression levels of ASC, caspase-1, NLRP3, VDR, TLR4, MyD88, and NF-κB in each group are shown in Figure 3. The protein expression levels of ASC, caspase-1, NLRP3, TLR4, MyD88, and NF-κB in the LPS group were evidently higher than those in the control group, while the VDR expression in the LPS group was evidently lower than that in the control group (P<0.05). The protein expression levels of ASC, caspase-1, NLRP3, TLR4, MyD88, and NF-κB in the LPS + EB1089 group were evidently lower than those in the LPS group, while the VDR expression in LPS + EB1089 group was evidently higher than that in the LPS group (P<0.05). Therefore, EB1089 pretreatment inhibited LPS-induced inflammation in HT-29 cells.
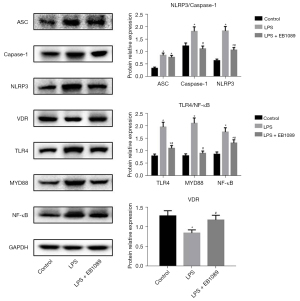
Discussion
Toll-like receptor 4 (TLR4) is typical in the innate immune system of the human body. After recognizing pathogen-related molecules, the membrane pattern recognition receptors activate their cytoplasmic part and interact with the adaptor protein MyD88. Then, IL-1 receptor associated kinase-4 (IRAK-4) is recruited to activate the pathway, leading to the activation of MAPK and NF-κB; then, IRAK-4 enters the nucleus to regulate the transcriptional expression of downstream genes, and the expression of proteins constituting inflammatory bodies will also increase (13,14). A previous study a demonstrated that treatment with the active form of vitamin D (1,25(OH)D3) of colonic epithelial cells stimulated by LPS reduced tumor necrosis factor-α (TNF-α) and increased IL-10 (15). Typical inflammatory bodies include a cytoplasmic pattern recognition receptor (such as NLRP3), an adaptor protein (ASC), and pro-caspase-1. Inflammasome can process and activate caspase-1 and the pro-inflammatory cytokines IL-1β and IL-18 to induce an inflammatory response (16-18), so we detected IL-1β and IL-18 in this study. Our results indicated that EB1089 pretreatment significantly reduced the effect of LPS on the assembly of NLRP3 inflammasome in intestinal epithelial cells, and increased the VDR expression. Conversely, the LPS treatment significantly inhibited the VDR expression. It was reported that VDR promoted the ubiquitination of NLRP3 (12), so it could be speculated that EB1089 promoted the VDR expression and the degradation of inflammatory bodies in intestinal epithelial cells, resulting in the reduction of inflammatory response.
In this study, we also found that EB1089 pretreatment restored the expression of TLR4, MyD88, NF-κB, and other proteins to normal levels. This result indicated that the TLR4/NF-κB pathway was also activated by inflammasome-induced inflammatory responses. The increased IL-1β secretion triggered by the increased assembly of inflammasomes activated the downstream NF-κB through the IL-1R receptor combined with MyD88 (19). In brain tissues, activation of the inflammasome is associated with seeding and spreading of amyloid-β pathology, NLRP3 inflammasome activity also leads to the release of assembled ASC specks, which, once released into the intercellular space, could be taken up by neighboring myeloid cells to maintain the ongoing immune response (20). In the present study, the EB1089 pretreatment had no significant effect on the secretion of IL-1β and IL-18. The expression of ASC did not significantly differ between the LPS and LPS + EB1089 groups. Therefore, EB1089 directly inhibited the TLR4/NF-κB pathway by activating VDR.
Conclusions
In summary, this study demonstrated that the EB1089 pretreatment reduced LPS-induced inflammatory response in intestinal epithelial cells. The mechanism of this effect might involve the inhibitory effect of EB1089 against NLRP3 inflammasome formation and the TLR4/NF-κB pathway activity by promoting VDR expression. Therefore, EB1089 could elicit a therapeutic effect on UC and other intestinal inflammatory diseases.
Acknowledgments
Funding: This study was supported by the Science and Technology Program of Fujian Provincial Health Commission (No. 2020CX0101), Fujian Natural Science Foundation Project (No. 2020J01120438), and Fujian Medical Innovation Project (No. 2020CX01010070).
Footnote
Reporting Checklist: The authors have completed the MDAR reporting checklist. Available at https://atm.amegroups.com/article/view/10.21037/atm-22-1066/rc
Data Sharing Statement: Available at https://atm.amegroups.com/article/view/10.21037/atm-22-1066/dss
Conflicts of Interest: All authors have completed the ICMJE uniform disclosure form (available at https://atm.amegroups.com/article/view/10.21037/atm-22-1066/coif). The authors have no conflicts of interest to declare.
Ethical Statement: The authors are accountable for all aspects of the work in ensuring that questions related to the accuracy or integrity of any part of the work are appropriately investigated and resolved.
Open Access Statement: This is an Open Access article distributed in accordance with the Creative Commons Attribution-NonCommercial-NoDerivs 4.0 International License (CC BY-NC-ND 4.0), which permits the non-commercial replication and distribution of the article with the strict proviso that no changes or edits are made and the original work is properly cited (including links to both the formal publication through the relevant DOI and the license). See: https://creativecommons.org/licenses/by-nc-nd/4.0/.
References
- Reis GV, Gontijo NA, Rodrigues KF, et al. Vitamin D receptor polymorphisms and the polycystic ovary syndrome: A systematic review. J Obstet Gynaecol Res 2017;43:436-46. [Crossref] [PubMed]
- Toussaint ND, Damasiewicz MJ. Do the benefits of using calcitriol and other vitamin D receptor activators in patients with chronic kidney disease outweigh the harms? Nephrology (Carlton) 2017;22:51-6. [Crossref] [PubMed]
- Park WH, Seol JG, Kim ES, et al. Induction of apoptosis by vitamin D3 analogue EB1089 in NCI-H929 myeloma cells via activation of caspase 3 and p38 MAP kinase. Br J Haematol 2000;109:576-83. [Crossref] [PubMed]
- Roy S, Martel J, Tenenhouse HS. Comparative effects of 1,25-dihydroxyvitamin D3 and EB 1089 on mouse renal and intestinal 25-hydroxyvitamin D3-24-hydroxylase. J Bone Miner Res 1995;10:1951-9. [Crossref] [PubMed]
- Cong L, Wang WB, Liu Q, et al. FokI Polymorphism of the Vitamin D Receptor Gene Is Associated with Susceptibility to Gastric Cancer: A Case-Control Study. Tohoku J Exp Med 2015;236:219-24. [Crossref] [PubMed]
- Olson KC, Kulling Larkin PM, Signorelli R, et al. Vitamin D pathway activation selectively deactivates signal transducer and activator of transcription (STAT) proteins and inflammatory cytokine production in natural killer leukemic large granular lymphocytes. Cytokine 2018;111:551-62. [Crossref] [PubMed]
- Li A, Yi B, Han H, et al. Vitamin D-VDR (vitamin D receptor) regulates defective autophagy in renal tubular epithelial cell in streptozotocin-induced diabetic mice via the AMPK pathway. Autophagy 2022;18:877-90. [Crossref] [PubMed]
- Zhao H, Zhang H, Wu H, et al. Protective role of 1,25(OH)2 vitamin D3 in the mucosal injury and epithelial barrier disruption in DSS-induced acute colitis in mice. BMC Gastroenterol 2012;12:57. [Crossref] [PubMed]
- Chen Y, Du J, Zhang Z, et al. MicroRNA-346 mediates tumor necrosis factor α-induced downregulation of gut epithelial vitamin D receptor in inflammatory bowel diseases. Inflamm Bowel Dis 2014;20:1910-8. [Crossref] [PubMed]
- Lu D, Lan B, Din Z, et al. A vitamin D receptor agonist converts CD4+ T cells to Foxp3+ regulatory T cells in patients with ulcerative colitis. Oncotarget 2017;8:53552-62. [Crossref] [PubMed]
- Huang H, Hong JY, Wu YJ, et al. Vitamin D receptor interacts with NLRP3 to restrict the allergic response. Clin Exp Immunol 2018;194:17-26. [Crossref] [PubMed]
- Rao Z, Chen X, Wu J, et al. Vitamin D Receptor Inhibits NLRP3 Activation by Impeding Its BRCC3-Mediated Deubiquitination. Front Immunol 2019;10:2783. [Crossref] [PubMed]
- Kawai T, Akira S. The role of pattern-recognition receptors in innate immunity: update on Toll-like receptors. Nat Immunol 2010;11:373-84. [Crossref] [PubMed]
- Rathinam VA, Fitzgerald KA. Inflammasome Complexes: Emerging Mechanisms and Effector Functions. Cell 2016;165:792-800. [Crossref] [PubMed]
- Gubatan J, Mehigan GA, Villegas F, et al. Cathelicidin Mediates a Protective Role of Vitamin D in Ulcerative Colitis and Human Colonic Epithelial Cells. Inflamm Bowel Dis 2020;26:885-97. [Crossref] [PubMed]
- Ketelut-Carneiro N, Silva GK, Rocha FA, et al. IL-18 triggered by the Nlrp3 inflammasome induces host innate resistance in a pulmonary model of fungal infection. J Immunol 2015;194:4507-17. [Crossref] [PubMed]
- Jo EK, Kim JK, Shin DM, et al. Molecular mechanisms regulating NLRP3 inflammasome activation. Cell Mol Immunol 2016;13:148-59. [Crossref] [PubMed]
- Shao BZ, Xu ZQ, Han BZ, et al. NLRP3 inflammasome and its inhibitors: a review. Front Pharmacol 2015;6:262. [Crossref] [PubMed]
- Swanson KV, Deng M, Ting JP. The NLRP3 inflammasome: molecular activation and regulation to therapeutics. Nat Rev Immunol 2019;19:477-89. [Crossref] [PubMed]
- Venegas C, Kumar S, Franklin BS, et al. Microglia-derived ASC specks cross-seed amyloid-β in Alzheimer's disease. Nature 2017;552:355-61. [Crossref] [PubMed]
(English Language Editor: J. Jones)