The effect of TLR-4 on the proliferation and differentiation of bone mesenchymal stem cells and its relationship with the Wnt signal transduction pathway during bone nonunion
Introduction
Nowadays, fractures have become a relatively common problem, but the treatment of fractures is becoming more expensive and complex. Furthermore, the epidemiology of fractures is poorly understood (1). As a complication of fracture, bone nonunion accounts for about 5–10% of all fractures (2,3). Bone nonunion is defined by the US Drug and Food Administration (FDA) as a fracture that has existed for more than 9 months and has shown no signs of healing in the last 3 months (4). This kind of fracture, which is slow or difficult to heal, is extremely painful and the patient’s quality of life is poor. The treatment of bone nonunion should start from the source, but research on the mechanism of bone nonunion is still insufficient at present.
Toll-like receptors (TLRs) are the most canonical pattern recognition receptors (PRRs) in the innate immune recognition mechanism (5). They have been the research topic of many studies (6-8). TLRs are believed to be associated with a considerable number of diseases and treatments, such as allergies (9), tick-borne encephalitis (10), and prostate cancer (11), among others. TLR-4 is a membrane-bound TLR that plays an important role in the treatment of severe sepsis associated with Gram-negative bacterial infections, autoimmune diseases, inflammation, and post-traumatic illness (5). However, its role in the development of bone nonunion remains unclear.
The Wnt gene was first identified in fruit flies, where it was thought to be a mutation that causes the loss of wings and limbs (12). However, it was later found that the gene expression product of Wnt is a glycoprotein that can modify lipid secretion and has functions of regulating cell polarity, morphogenetic movement, and axial development in vertebrates (13). The human genome contains 19 Wnt genes, each of which has different expression modes and plays different roles in the human body (14). They regulate many complex cascades of signaling pathways, which can be divided into β-catenin-dependent canonical pathways and β-catenin-independent non-canonical pathways (15). Wnt signaling pathways play different roles in many diseases (16-18), though the roles of the 2 Wnt gene pathways in the occurrence of bone nonunion are unknown.
In this study, we established a rat model of femoral nonunion, and detected the expression of TLR-3, TLR-4, β-catenin, nemo-like kinase (NLK), c-Jun N-terminal kinase (JNK), and other proteins during the occurrence of bone nonunion, so as to analyze the regulatory role of each protein in the occurrence of bone nonunion. Subsequently, we conducted in vitro experiments on bone marrow mesenchymal stem cells (BMSCs) to explore the effect of TLR-4 on the proliferation of BMSCs and the expression level of the Wnt signaling pathway under different culture conditions, so as to infer the role of TLR-4 in the occurrence of bone nonunion and whether the Wnt signaling pathway is involved in its regulation. The results showed that TLR-4 could inhibit the proliferation and differentiation of BMSCs, and the Wnt signal transduction pathway might be involved in the regulation of bone nonunion. In this process, the canonical/non-canonical Wnt signal transduction pathways may undergo pathway interconversion. Our study will provide new ideas for the treatment of bone nonunion. We present the following article in accordance with the ARRIVE reporting checklist (available at https://atm.amegroups.com/article/view/10.21037/atm-22-1261/rc).
Methods
Establishment of the rat model of femoral nonunion
According to the method used in the literature (19), the rat femoral nonunion model was constructed. Twelve Sprague Dawley (SD) rats with an average age of 9 weeks (within 4 days) and a body weight of about 300 g were selected (purchased from Shanghai Slack Laboratory Animal Co., Ltd., China). All animal experiments were conducted in Guangzhou General Biomedical Technology Co., Ltd. The rats were anesthetized by injecting 100 mg/kg ketamine and 10 mg/kg thiazide into the intraperitoneal cavity. The hair on the legs of the rats was shaved, then rats were treated with povidone iodine and ethanol. A lateral incision was made along the middle shaft of the femur and the vastus was cut vertically, using forceps to separate the femur. The soft tissue of the lateral femur was stripped. A transverse osteotomy of the middle femur was performed with a micro high-speed drill. The femoral shaft was secured with a miniature external fixation bracket. After fixation, femur fracture was manually performed on the lateral side of the cortical osteotomy site. The lateral periosteum of the fracture site was cauterised proximally to 2 mm and distally to 2 mm. Finally, the lateral femoral incision was closed with absorbable sutures. The femoral nonunion model rats and the control rats were examined by X-ray at 0, 2, 4, 6, 8, 10, and 12 weeks. Hematoxylin and eosin (HE) staining was performed on both the nonunion group and the control group at 12 weeks.
The rats were given sufficient water and rat food during the experiment. This experiment was performed under a project license (No. G202105) granted by the Experimental Animal Ethics Committee of Guangzhou General Biomedical Technology Co., Ltd., in compliance with the guidelines of Guangzhou General Biomedical Technology Co., Ltd. for the care and use of animals.
Construction of in vitro experiments
Adult SD rats were sacrificed under routine anesthesia. Bilateral femurs and tibias of the rats were collected and then soaked in 75% ethanol for 15 minutes. The femur and tibia were separated on an ultra-clean worktable and the surface fur muscle and fascia tissue were removed, followed by washing with phosphate buffer saline (PBS) 3 times. The 2 ends of the long bone were cut off. DMEM medium containing 20% FBS (Shanghai Yuchun Biological Technology Co., Ltd.) was injected into the bone marrow cavity with a syringe, and the cells were flushed. The culture medium containing bone marrow cells were aspirated, placed in a test tube, and mixed to form a cell suspension. Cells were centrifuged at 1,000 rpm for 5 minutes, the liquid was stratified, the nuclear cell layer (white) was aspirated with a pipette, then cells were centrifuged with complete medium. DMEM medium was added to resuspend the cells, and the cell concentration was adjusted to 1×105/mL. Cells were then inoculated in a culture flask containing 20% calf serum and cultured in vitro at 37 ℃ with 5% CO2 and saturated humidity. After 48 hours, the medium was changed once every 3 days and PBS was added to remove non-adherent interfering cells. When the confluence degree reached 90%, the adherent cells were washed with PBS, digested with 0.25% trypsin (Merck, Germany) for 5 minutes, and then passage was performed at a ratio of 1:3. The third-generation cells were obtained and divided into 4 groups for culture. The first group was treated with 10 ng/mL LPS, the second group was treated with 10 ng/mL LPS and 10 ng/mL CLI095, the third group was the control group, and the fourth group was treated with 10 ng/mL of substance P (SP).
Flow cytometry
The third-generation cultured cells were digested with 0.25% trypsin for 5 minutes. After centrifugation at 1,000 rpm for 10 minutes, the cells were washed twice with PBS, and then the cells were resuspended with PBS to prepare cell suspensions. The cell concentration was adjusted to 2×106/mL. The cells were divided into flow centrifuge tubes at 500 µL per tube, and CD11B-PE (Abcam Cambridge, UK), CD29-PE (Abcam, UK), CD-34-FITC (Abcam, UK), CD45-PE (Abcam, UK), CD90-PE (Abcam, UK), CD105 PE (Abcam, UK), and the isotype control (Abcam, UK) were added. The amount of each added antibody was 10 µL. After incubation at 4 °C for 30 minutes, cells were washed twice with PBS, then 4% paraformaldehyde (Guangzhou Jibeisi Biological Technology Co., Ltd.) at 200 µL was added to each tube to resuspend the cells. A filter membrane was used to filter cell masses to prevent blockage of the flow cytometer. Cells were then fed into the flow cytometer for identification.
Immunohistochemistry
Tissues from the rat fracture area were fixed with 4% formalin. Then, the tissues were paraffin-embedded, sectioned, dewaxed, and antigen repaired. Catalase in tissues was blocked with 3% H2O2, and then tissues were washed with PBS twice. Serum was added to the tissue to block some non-specific binding sites. After 1 hour of blocking, anti-β-catenin antibody (Abcam, 1:500, UK), anti-NLK antibody (Abcam, 1:500, UK), anti-JNK antibody (Abcam, 1:800, UK), and anti-RhoA antibody (Abcam, 1:800, UK) were added and incubated overnight in a refrigerator at 4 ℃. The tissue sections were taken out from the refrigerator and washed in PBS 3 times for 5 minutes each time. The PBS around the tissue was dried and the secondary antibody was added, then tissues were placed in an incubator at 37 °C for 30 minutes. The slides were taken out of the incubator and washed in PBS 3 times for 5 minutes each. After drying the PBS around the tissues, DAB chromogenic agent (Maixin) was added for 5 minutes. The tissue sections were counterstained and sealed.
Quantitative real-time PCR (qRT-PCR)
The cell suspension was collected and 1 c Trizol reagent was added. Subsequently, 500 µL isopropyl alcohol was added, mixed well, and the suspension was stood on ice for 10 minutes. After centrifugation at 12,000 rpm at 4 ℃ for 10 minutes, the supernatant was discarded. Then, 1 mL 75% ethanol prepared by ddH2O was added, followed by centrifugation at 10,000 rpm at 4 ℃ for 5 minutes. The supernatant was removed, the pellet was dried, and 20 µL ddH2O was added and mixed repeatedly to obtain the RNA solution. Using the TAKARA kit (Merck, Germany), 1 µg RNA was reverse transcribed according to the instructions. After completion, cDNA was removed and 180 µL ddH2O was added. The 10 µL reaction mixture was composed of 2.5 µL of 10-fold diluted cDNA, 5 µL of the fluorescent dye SYBR Green, 0.5 µL of upstream and downstream primers, and 1.5 µL of DEPC water. Finally, the obtained data were imported into statistical software for analysis. The reaction conditions were as follows: 50 ℃ for 2 minutes, 95 ℃ for 2 minutes, 95 ℃ for 15 seconds, 60 ℃ for 32 seconds, total 40 cycles.
The primer sequences were as follows: alkaline phosphatase (ALP): forward, 5'-ACACCTTGACTGTGGTTACTG-3' and reverse, 5'-CCATATAGGATGGCCGTGAAG-3'; osteocalcin: forward, 5'-AATAGCCCTGGCAGATTCCC-3' and reverse, 5'-CTCTCATGGTGTCTCGGTGG-3'.
Western blot
Cell suspensions were collected and centrifuged at 13,000 rpm at 4 ℃ for 3 minutes and the supernatant was obtained. A standard protein solution was used to prepare a series of protein solutions with concentration gradients. Then, 100 µL of each concentration was taken and added to 10 µL of Coomassie Bright Blue (Yeasen, USA) solution. After detection under a spectrophotometer, the standard curve was drawn with statistical data and the protein concentration was calculated. Subsequently, 100 µL supernatant was taken, 20 µL loading buffer was added, and water was boiled at 100 ℃ for 5 minutes to denature the proteins. After being placed on ice for 3 minutes, the sample was centrifuged at 13,000 rpm at 4 ℃ for 15 minutes. SDS-PAGE gel was used for electrophoresis with the concentrated gel running at 80 V and the separating gel running at 120 V. The PVDF membrane of appropriate size was cut, soaked in methanol for 2 minutes, and pretreated with membrane transfer solution. The PVDF membrane and PAGE gel after electrophoresis were assembled, and the membrane was transferred at 0 ℃ and 70 V for 2 hours. The membrane was covered with 5% skim milk powder on a shaker for 1 hour and incubated overnight with the primary antibody at 4 ℃. The protein expression levels of β-catenin, c-Myc, NLK, and JNK were detected by specific antibodies. The antibodies used are as follows: rabbit anti-β-catenin (1:800 dilution; Invitrogen, USA), rabbit anti-c-Myc (1:1,000 dilution; Invitrogen, USA), rabbit anti-JNK (1:1,000 dilution; Invitrogen, USA), and rabbit anti-NLK (1:1,000 dilution; Invitrogen, USA). Membranes were washed in PBST 5 times, and the secondary antibody was added for incubation for 2 hours. Membranes were washed again in PBST 5 times and then washed with pure water 5 times. The chemiluminescence system was used for 3 minutes, and the images were recorded.
Alizarin Red staining
The paraffin sections were dewaxed in 95% ethanol, then the slides were placed upright and thoroughly air-dried. The slices were immersed and dyed in the Alizarin Red S staining solution (Beijing Solebo Technology Co., Ltd.) and rinsed quickly with distilled water. The slices were dehydrated, made transparent, and sealed with neutral gum. The calcium deposits were orange-red.
ALP staining
The sterile slides were put into a petri dish, and the appropriate amount of cell suspension was added during passaging to make cell slides. The sterile slides were removed from the petri dish after being covered with the cells. After washing with PBS, slides were fixed with cold propanol for 10 minutes, then rinsed several times with distilled water. The cell slides were placed in the incubation solution and incubated at 37 ℃ for 4–6 hours. The cell slides were soaked in 2% cobalt nitrate for 3–5 minutes and washed with distilled water several times. The cell slides were then placed in 1% ammonium sulfide for 2 minutes, washed with running water, dried naturally, and sealed.
MTT assay
Logarithmic phase cells were collected and the cell suspension concentration was adjusted. Then, 100 µL cell suspension was added to each well and the density of the cells was adjusted to 1,000–10,000 cells/well. The cells were incubated at 5% CO2 and 37 ℃ until the cells were in a monolayer at the bottom of the well (96-well flat plate). The cells were treated with appropriate drugs according to the groups. Cells were incubated at 5% CO2 and 37 ℃ for 16–48 hours and observed under an inverted microscope. Then, 20 µL MTT solution at 5 mg/mL (Merck, Germany) was added to each well, and the culture was continued for 4 hours. The culture was terminated and the culture fluid was carefully aspirated from the well. Subsequently, 150 µL dimethyl sulfoxide was added to each well, and the crystals were fully dissolved by shaking at low speed for 10 minutes. The optical density (OD) value of each well was measured at OD 450 nm by a microplate reader.
Statistical analysis
All data in this study were analyzed using GraphPad Prism 8 (GraphPad Software, USA), and significance was assessed using either the t-test or one-way ANOVA. P<0.05 indicates that the difference is statistically significant.
Results
The expression of TLR-3, TLR-4, and neuropeptide SP in bone nonunion
The femoral nonunion model rats and the control group were examined by X-ray at 0, 2, 4, 6, 8, 10, and 12 weeks. The results showed that the femurs in the nonunion group were still fractured at 12 weeks, while the femurs in the normal control group had basically healed completely (Figure 1A). HE staining was performed on both the nonunion group and the control group at 12 weeks, and the results showed that there were significant fractures in the femurs of the nonunion group, while there were almost no fractures in the control group (Figure 1B). As shown by the X-ray results, the bone nonunion model was successfully established. The mRNA expression levels of TLR-3, TLR-4, SP, and Calcitonin Gene-Related Peptide (CGRP) were detected at 1 and 3 days and at 4, 6, 8, and 12 weeks. The results showed that the mRNA expression levels of TLR-3 and TLR-4 in the bone nonunion group were much higher than those in the control group, and the expression of TLR-3 showed a trend of increasing first and then decreasing, while the expression of TLR-4 increased over the whole period (Figure 2A). These results suggest that both factors may play a positive role in the occurrence of bone nonunion. The expression levels of SP and CGRP were far lower than the normal levels, and the difference was statistically significant (P<0.05; Figure 2B).
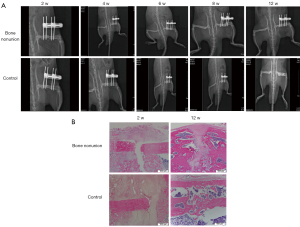
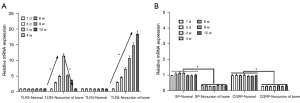
The expression of core genes and proteins in the atypical Wnt signaling pathway and typical Wnt signaling pathway during the occurrence of bone nonunion
The expression levels of β-catenin, NLK, JNK, RhoA, Rac, and neuropeptides SP and Nerve Peptide Y (NPY) were detected on the 1st and 3rd day and at the 2nd, 4th, 6th, 8th, and 12th weeks. Western blot electrophoresis bands are shown in Figure 3A. Western blot results showed that the protein expression of β-catenin in the canonical Wnt signaling pathway during bone nonunion increased rapidly from 0 to 2 weeks and decreased rapidly from 2 to 8 weeks. The expression of NLK, RhoA, Rac, and JNK in the non-canonical Wnt signaling pathway was constantly on the rise, while the expression of neuropeptide SP was significantly different in the first 2 weeks and after 2 weeks (P<0.05). However, the expression of NPY showed no significant fluctuation (Figure 3B). These results suggest that the non-canonical Wnt signaling pathway and canonical Wnt signaling pathway may play a role in the process of bone nonunion. The mRNA expression levels of β-catenin, NLK, and JNK were detected by qRT-PCR. The results were basically the same as those of western blot (Figure 3C). Immunohistochemical detection results showed that the expression of β-catenin decreased during the 2nd to 8th week, while the expressions of NLK, JNK, and other proteins increased (Figure 4), which was also confirmed by the qRT-PCR and western blot results. These results showed that in the occurrence of bone nonunion, the expression of β-catenin increased in the first 2 weeks, and gradually decreased after 2 weeks, while the expression of other non-canonical Wnt signaling pathway core proteins did not change significantly in the first 2 weeks, and the expression of all proteins increased after 2 weeks.
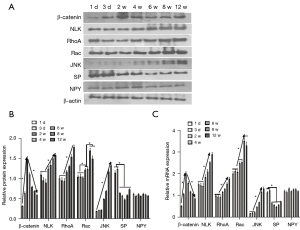
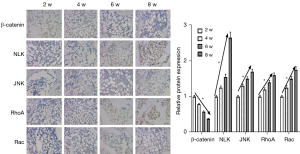
TLR-4 may regulate BMSC proliferation and differentiation through the Wnt signaling pathway
BMSCs were cultured in vitro. The cells were identified by flow cytometry and the results showed that the cultured cells were BMSCs. Cells were subcultured to the third generation. The third-generation cells were cultured into 4 groups. The first group was treated with a TLR-4 agonist (LPS), the second group was treated with a TLR-4 agonist and inhibitor (LPS + CLI095), the third group was the control group, and the fourth group was treated with TLR-4 agonist and SP (LPS + SP) to promote the proliferation of BMSCs. Alizarin Red staining was used to detect the osteogenic differentiation ability of cells in the 4 groups. The results showed that the osteogenic differentiation ability of cells in the LPS group was the lowest. The osteogenic differentiation ability of cells in the LPS + CLI095 group was slightly improved, but still lower than that in the control group. The LPS + SP group had the strongest osteogenic differentiation ability (Figure 5A). ALP staining was used to detect the expression of ALP, and the results were similar to Alizarin Red staining (Figure 5B). At the 1st, 2nd, 3rd, and 4th week of culture, the proliferation ability of BMSCs was detected, and the results showed that the number of cells in the LPS group and the LPS + CLI095 group was lower than that in the control group, while the number of cells in the LPS + SP group was higher than that in the control group (Figure 6A). The number of cells in the LPS group was significantly lower than that in the LPS + CLI095 group, and the difference was statistically significant (P<0.05). Furthermore, qRT-PCR was used to detect the mRNA expression levels of the osteogenesis-related genes collagen I, osteocalcin, and Runx2. The results showed that the expression levels of these 3 genes were similar in the 4 groups. Their expression levels were the lowest in the LPS group, and partially increased in the LPS + CLI095 group, but were still lower than the control group, while their expression levels were the highest in the LPS + SP group (Figure 6B).
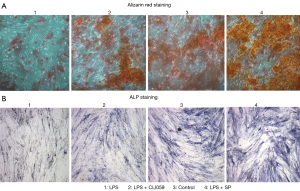
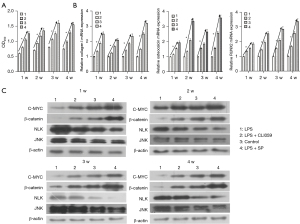
The protein expression levels of β-catenin, c-Myc, NLK, and JNK were detected by western blot. The results showed that the expression levels of c-Myc and β-catenin in the LPS + SP group were higher than those in the control group, while the expression levels of NLK and JNK were lower than those in the control group. In the LPS group and the LPS + CLI095 group, the protein expression levels of β-catenin and c-Myc were lower than those in the control group, while the protein expression levels of NLK and JNK were higher than those in the control group (Figure 6C). These results indicate that the proliferation of BMSCs may be positively correlated with the expression of β-catenin and negatively correlated with the expression of NLK and JNK. The Wnt signaling pathway may be involved in the occurrence of bone nonunion, and TLR-4 inhibited the proliferation and osteogenic differentiation of BMSCs.
Discussion
Patients with bone nonunion will often feel pain, affecting their quality of life. They may also face physical disabilities and mental health problems (2). This makes the study of bone nonunion a necessity, and there have been many theories and studies on bone nonunion (20-22). From a macro perspective, there are many causes of bone nonunion, such as osteoporosis, obesity, smoking, and alcoholism, among others (23). However, the mechanism of bone nonunion is still poorly understood. Previous studies have shown that Wnt/β-catenin signaling pathway was involved in bone formation in nonunion (15). In this study, the influence of TLR-4 and Wnt signaling pathways on the occurrence of bone nonunion was investigated.
TLRs, due to their unique status in immune recognition, are considered to have special associations with a considerable number of diseases (24,25). A study has also shown that TLRs may be associated with bone nonunion (26). Therefore, we established a model of femoral nonunion in SD rats and detected the difference in TLR expression between the femoral nonunion model group and the control group. The results showed that the expression levels of TLR-3 and TLR-4 in bone nonunion model rats were significantly higher than those in the control group. The expression of TLR-3 was firstly increased and then decreased, while the expression of TLR-4 was constantly increased (Figure 2A). TLR-3 expression may be associated with bone nonunion to some extent, but from these findings, TLR-4 is more closely related to bone nonunion. Therefore, we designed controlled experiments related to TLR-4. In the culture of BMSCs, the TLR-4 agonist LPS was added in one group, while the TLR-4 inhibitor CLI095 and LPS were added in the other group, and the proliferation and differentiation of BMSCs were detected. The results showed that although the proliferation and differentiation ability of BMSCs in the LPS group and the LPS + CLI095 group were lower than those in the control group, there were still significant differences between the 2 groups. The proliferation and differentiation ability of BMSCs in the LPS group was lower than that in the LPS + CLI095 group, and the statistical analysis showed that the difference was significant (P<0.05) (Figure 6A). The results of osteogenic differentiation and ALP expression in BMSCs were also similar to the results of proliferation (Figure 5A,5B). These results indicated that TLR-4 could significantly inhibit the proliferation and differentiation of BMSCs, and thus affect the occurrence of bone nonunion.
The Wnt gene plays an important role in the development of cells, and since it was first discovered, it has been implicated in every aspect of developmental biology (27). Some studies have also shown that the Wnt signaling pathway seems to have a certain connection with bone nonunion (28,29), but the correlation between the two is still not clear. In order to further explore the relationship between the Wnt signaling pathway and bone nonunion, we detected the expression of Wnt signaling pathway proteins during the establishment of the bone nonunion model. The results of qRT-PCR, western blot, and immunohistochemistry were basically the same (Figures 3,4), and the expression of β-catenin, the core protein of the canonical Wnt signaling pathway, increased in the first 2 weeks of model construction, and then began to decrease. The expression levels of other non-canonical Wnt signaling pathway proteins, such as NLK, JNK, RhoA, and Rac, were basically unchanged in the first 2 weeks of model construction, and then gradually increased, indicating a certain causal association among the canonical Wnt signaling pathway, the non-canonical signaling pathway, and bone nonunion. We then carried out relevant in vitro experiments and detected the expression of various proteins in the LPS + SP group, LPS group, and LPS + CLI095 group. The results showed that in the group with poor proliferation and differentiation ability of BMSCs, the expression levels of β-catenin and c-Myc, core proteins of the canonical Wnt signaling pathway, were low, while the expression levels of non-canonical Wnt signaling pathway proteins NLK and JNK were high. Similarly, the expression of β-catenin and c-Myc was higher in the group with higher proliferation and differentiation ability, while the expression levels of NLK and JNK were lower (Figure 6C). This suggests that the Wnt signaling pathway may have a regulatory effect on BMSCs. The canonical Wnt signaling pathway promotes the proliferation of BMSCs, while the non-canonical Wnt signaling pathway inhibits the proliferation of BMSCs. Combined with the changes in β-catenin, NLK, JNK, and other proteins in the bone nonunion model, we hypothesized that the canonical Wnt signaling pathway may be transformed into the non-canonical Wnt signaling pathway during the construction of the bone nonunion model, which promotes the formation of bone nonunion. In this study, we hypothesized that TLR-4 affects the proliferation and differentiation of BMSCs by regulating the transformation of canonical Wnt signaling pathways.
Other studies have shown that Wnt pathway related genes have certain effects on the process of bone regeneration, and this study also proved that TLR-4 can affect the process of bone regeneration by regulating the Wnt signaling pathway (28,29). This study provides a new research direction for exploring the mechanism of bone regeneration. There are some limitations in this study. We only studied the relationship between bone nonunion, TLR-4, and the Wnt signaling pathways, but did not investigate whether bone nonunion was regulated by other signaling pathways. Meanwhile, this study demonstrated that the transformation of Wnt signaling pathway inhibits osteoblast differentiation of BMSCs. However, further studies are needed to prove how the Wnt signaling pathway influences the bone regeneration process. This will be further investigated in future studies.
Conclusions
In conclusion, this study found that TLR-4 can significantly inhibit the proliferation and differentiation of BMSCs. The Wnt signaling pathway may regulate the occurrence of bone nonunion, and in this process, the canonical Wnt signaling pathway may be transformed into the non-canonical Wnt signaling pathway. This may provide a new way of thinking about bone nonunion.
Acknowledgments
Funding: The study was supported by Medical Scientific Research Foundation of Guangdong Province, China (No. B2021401) and Guangzhou Science and Technology Project of China (No. 201904010053).
Footnote
Reporting Checklist: The authors have completed the ARRIVE reporting checklist. Available at https://atm.amegroups.com/article/view/10.21037/atm-22-1261/rc
Data Sharing Statement: Available at https://atm.amegroups.com/article/view/10.21037/atm-22-1261/dss
Conflicts of Interest: All authors have completed the ICMJE uniform disclosure form (available at https://atm.amegroups.com/article/view/10.21037/atm-22-1261/coif). All authors report that this study was supported by Medical Scientific Research Foundation of Guangdong Province, China (No. B2021401) and Guangzhou Science and Technology Project of China (No. 201904010053). The authors have no other conflicts of interest to declare.
Ethical Statement: The authors are accountable for all aspects of the work in ensuring that questions related to the accuracy or integrity of any part of the work are appropriately investigated and resolved. This experiment was performed under a project license (No. G202105) granted by the Experimental Animal Ethics Committee of Guangzhou General Biomedical Technology Co., LTD, in compliance with guidelines of Guangzhou General Biomedical Technology Co., Ltd. for the care and use of animals.
Open Access Statement: This is an Open Access article distributed in accordance with the Creative Commons Attribution-NonCommercial-NoDerivs 4.0 International License (CC BY-NC-ND 4.0), which permits the non-commercial replication and distribution of the article with the strict proviso that no changes or edits are made and the original work is properly cited (including links to both the formal publication through the relevant DOI and the license). See: https://creativecommons.org/licenses/by-nc-nd/4.0/.
References
- Tuček M, Chochola A, Klika D, et al. Epidemiology of scapular fractures. Acta Orthop Belg 2017;83:8-15. [PubMed]
- Zura R, Xiong Z, Einhorn T, et al. Epidemiology of Fracture Nonunion in 18 Human Bones. JAMA Surg 2016;151:e162775. [Crossref] [PubMed]
- Calori GM, Mazza E, Colombo M, et al. Treatment of long bone non-unions with polytherapy: indications and clinical results. Injury 2011;42:587-90. [Crossref] [PubMed]
- Calori GM, Mazza EL, Mazzola S, et al. Non-unions. Clin Cases Miner Bone Metab 2017;14:186-8. [Crossref] [PubMed]
- Patra MC, Choi S. Recent progress in the development of Toll-like receptor (TLR) antagonists. Expert Opin Ther Pat 2016;26:719-30. [Crossref] [PubMed]
- Fitzgerald KA, Kagan JC. Toll-like Receptors and the Control of Immunity. Cell 2020;180:1044-66. [Crossref] [PubMed]
- Liu CH, Liu H, Ge B. Innate immunity in tuberculosis: host defense vs pathogen evasion. Cell Mol Immunol 2017;14:963-75. [Crossref] [PubMed]
- Vijay K. Toll-like receptors in immunity and inflammatory diseases: Past, present, and future. Int Immunopharmacol 2018;59:391-412. [Crossref] [PubMed]
- Michels KR, Lukacs NW, Fonseca W. TLR Activation and Allergic Disease: Early Life Microbiome and Treatment. Curr Allergy Asthma Rep 2018;18:61. [Crossref] [PubMed]
- Moniuszko-Malinowska A, Penza P, Czupryna P, et al. Assessment of TLR-2 concentration in tick-borne encephalitis and neuroborreliosis. Scand J Clin Lab Invest 2019;79:502-6. [Crossref] [PubMed]
- Kalantari E, Abolhasani M, Roudi R, et al. Co-expression of TLR-9 and MMP-13 is associated with the degree of tumour differentiation in prostate cancer. Int J Exp Pathol 2019;100:123-32. [Crossref] [PubMed]
- Zhan T, Rindtorff N, Boutros M. Wnt signaling in cancer. Oncogene 2017;36:1461-73. [Crossref] [PubMed]
- De A. Wnt/Ca2+ signaling pathway: a brief overview. Acta Biochim Biophys Sin (Shanghai) 2011;43:745-56. [Crossref] [PubMed]
- Nusse R, Varmus H. Three decades of Wnts: a personal perspective on how a scientific field developed. EMBO J 2012;31:2670-84. [Crossref] [PubMed]
- Girardi F, Le Grand F. Wnt Signaling in Skeletal Muscle Development and Regeneration. Prog Mol Biol Transl Sci 2018;153:157-79. [Crossref] [PubMed]
- Stewart DJ. Wnt signaling pathway in non-small cell lung cancer. J Natl Cancer Inst 2014;106:djt356. [Crossref] [PubMed]
- Polakis P. Wnt signaling in cancer. Cold Spring Harb Perspect Biol 2012;4:a008052. [Crossref] [PubMed]
- Gajos-Michniewicz A, Czyz M. WNT Signaling in Melanoma. Int J Mol Sci 2020;21:4852. [Crossref] [PubMed]
- Huang Z, Gu H, Yin X, et al. Bone regeneration using injectable poly (γ-benzyl-L-glutamate) microspheres loaded with adipose-derived stem cells in a mouse femoral non-union model. Am J Transl Res 2019;11:2641-56. [PubMed]
- Elliott DS, Newman KJ, Forward DP, et al. A unified theory of bone healing and nonunion: BHN theory. Bone Joint J 2016;98-B:884-91. [Crossref] [PubMed]
- Toosi S, Behravan N, Behravan J. Nonunion fractures, mesenchymal stem cells and bone tissue engineering. J Biomed Mater Res A 2018;106:2552-62. [Crossref] [PubMed]
- Yeo JH, Kim JY. Surgical Strategy for Scaphoid Nonunion Treatment. J Hand Surg Asian Pac Vol 2018;23:450-62. [Crossref] [PubMed]
- Emara KM, Diab RA, Emara AK. Recent biological trends in management of fracture non-union. World J Orthop 2015;6:623-8. [Crossref] [PubMed]
- Han W, Chen X, Wang X, et al. TLR-4, TLR-5 and IRF4 are diagnostic markers of knee osteoarthritis in the middle-aged and elderly patients and related to disease activity and inflammatory factors. Exp Ther Med 2020;20:1291-8. [Crossref] [PubMed]
- McClure R, Massari P. TLR-Dependent Human Mucosal Epithelial Cell Responses to Microbial Pathogens. Front Immunol 2014;5:386. [Crossref] [PubMed]
- Szczęsny G, Olszewski WL, Zagozda M, et al. Genetic factors responsible for long bone fractures non-union. Arch Orthop Trauma Surg 2011;131:275-81. [Crossref] [PubMed]
- Willert K, Nusse R. Wnt proteins. Cold Spring Harb Perspect Biol 2012;4:a007864. [Crossref] [PubMed]
- Sun L, Li Z, Xue H, et al. MiR-26a promotes fracture healing of nonunion rats possibly by targeting SOSTDC1 and further activating Wnt/β-catenin signaling pathway. Mol Cell Biochem 2019;460:165-73. [Crossref] [PubMed]
- Long H, Zhu Y, Lin Z, et al. miR-381 modulates human bone mesenchymal stromal cells (BMSCs) osteogenesis via suppressing Wnt signaling pathway during atrophic nonunion development. Cell Death Dis 2019;10:470. [Crossref] [PubMed]