Methodological study of directed differentiation of pluripotent stem cells into corneal endothelial cells
Introduction
The transparent outer surface of the eye, the cornea, is divided into five layers, which include, from outermost to innermost, the epithelial cell layer, Descemet’s membrane, the stromal layer, Descemet’s membrane, and the endothelial cell layer (1). The layers of the cornea are essential for the exertion of visual function, especially the corneal endothelial cell (CEC) layer. The CEC layer of the eye consists of a single layer of tightly connected hexagonal cells that can maintain the cellular matrix in a dehydrated state through the activity of Na+/K+-ATPase on the cell membrane and the presence of tight junctions, thereby maintaining corneal transparency (2,3). CECs do not have the capacity to regenerate in vivo and therefore cannot replace dead or damaged cells. Adult keratocyte density is about 2,500 cells/mm2, and CEC dystrophy and surgical trauma can decrease the density of CECs. If cell density is below 500 cells/mm2, corneal endothelial decompensation, progressive corneal edema, bullous keratopathy, and even blindness can result.
Corneal transplantation is the most effective clinical treatment for irreversible corneal endothelial decompensation. However, while visual rehabilitation can be achieved by corneal transplantation, corneal transplant rejection, poor postoperative visual acuity, and lack of suitable donor tissue are currently the greatest obstacles to corneal transplantation (4). Therefore, endothelial cell-based therapy has emerged as an alternative to corneal transplantation.
Because human CECs are difficult to culture and expand in vitro (5-8), pluripotent stem cell (PSC)-induced differentiated endothelial cells are an ideal source for cell-based endothelial cell transplantation. PSCs include embryonic stem cells (ESCs) and induced PSCs (iPSCs). ESCs are able to induce differentiation of CECs in vitro. However, this approach is ethically controversial and can result in rejection reactions, limiting its clinical application. The use of CECs obtained by induced differentiation of human iPSCs (hiPSCs) from autologous or other allogeneic donor sources reduces the risk of immune rejection and avoids socio-ethical issues. This method has become the main direction for clinical application of in vitro induced differentiation of CECs.
At present, many corneal endothelial differentiation methods and practices simulate the process of corneal endothelial development and differentiation in vitro. Neural crest cells (NCCs) can differentiate into CECs during embryonic development (9), and this process could provide a strategy for inducing multifunctional stem cells into NCCs, after which, NCCs are induced into CECs. In a previous study, epithelial cell-conditioned medium and/or corneal stroma cell-conditioned medium was included with the induction medium (10) (the conditioned medium). However, because the chemical composition of the culture medium was not clear, the components that play a role in inducing differentiation could not be clearly obtained by these methods. With clear and simple components without serum or immunogenicity problems, the feeder-free induction method, using recombinant protein or small-molecule substances, is more suitable for future clinical applications.
In this study, the differentiation of hiPSCs into NCCs was induced by regulating transforming growth factor beta (TGF-β) and Wnt signaling pathways by adding SB4315542 and CHIR99021. The differentiation of NCCs into human CECs (hCECs) was then induced by adding B27, platelet-derived growth factor (PDGF)-BB, and XAV939 to CEC culture medium. This approach provided a simple and efficient method for directed differentiation of iPSC into CEC with clear chemical composition and serum-free media. We present the following article in accordance with the MDAR reporting checklist (available at https://atm.amegroups.com/article/view/10.21037/atm-22-1586/rc).
Methods
Cell culture and differentiation
Differentiation from hiPSC to NCC
- HiPSCs and ncEpic hPSC medium were obtained from Nuwacell Biotechnologies Co., Ltd. (Anhui, China). HiPSCs were seeded in Matrigel-coated 6-well plates and digested with ethylenediamine tetraacetic acid (0.5 mM EDTA; Nuwacell Biotechnologies Co., Ltd.) for 7.5 minutes. Once the degree of fusion reached 80%, 2.5 µM non-muscle myosin II inhibitor (Sigma-Aldrich, St. Louis, MO, USA) was added to the medium.
- The ncEpic medium (2 mL) was changed daily. After 48 hours, the cells reached 30% confluence and were replaced with NCC cell culture medium. The ingredients were: 50% Iscove’s modified Dulbecco’s medium (IMDM; 13390, Sigma-Aldrich), 50% F12 (N-6658, Sigma-Aldrich), 1× chemically defined lipid concentrate (11905-031, Thermo Fisher Scientific, Waltham, MA, USA), 5 µg/mL transferrin (T8158, Sigma-Aldrich), 5 µg/mL insulin (Sigma-Aldrich), 5 µg/mL fibroblast growth factor 2 (FGF2; PeproTech, Windsor, NJ, USA), 5 mg/mL bovine serum albumin (BSA; Sigma-Aldrich), 0.5× ncB27 Serum-Free Supplement (ncB27; Nuwacell Biotechnologies Co., Ltd.), 10 µM SB431542 (A8249, ApexBio, Houston, TX, USA), and 0.5 µM CHIR99021 (ApexBio). The medium was changed daily until day 7.
Differentiation from NCC to CEC
- NCCs were inoculated with laminin-511 (5 µg/well) coated in 6-plate wells at 0.1 mL/well. Once cell fusion reached 80%, cells were digested with 0.125% trypsin (Sigma-Aldrich) for 2–3 minutes, and an equal volume of trypsin inhibitor (Sigma-Aldrich) was added. NCCs were collected, and 2.5 µM non-muscle myosin II inhibitor (Sigma-Aldrich) was added to the CEC01 medium. The CEC01 medium was composed of: 50% IMDM (13390, Sigma-Aldrich), 50% F12 (Sigma-Aldrich), 1× chemically defined lipid concentrate (Thermo Fisher Scientific), 5 µg/mL transferrin (Sigma-Aldrich), 5 µg/mL insulin (Sigma-Aldrich), 5 mg/mL BSA (Sigma-Aldrich), 10 ng/mL FGF1 (PeproTech), 10 ng/mL PDGF-BB (PeproTech), 1 µM XAV939 (Sigma-Aldrich), and 0.5× ncB27 (Nuwacell Biotechnologies Co., Ltd.).
- After 24 hours, the medium was changed into CEC02, composing of: 50% IMDM (13390, Sigma-Aldrich), 50% F12 (Sigma-Aldrich), 1× chemically defined lipid concentrate (Thermo Fisher Scientific), 1 µg/mL transferrin (Sigma-Aldrich), 5 µg/mL insulin (Sigma-Aldrich), 5 mg/mL BSA (Sigma-Aldrich), 5 ng/mL FGF1 (PeproTech), 10 ng/mL PDGF-BB (PeproTech), 0.5 µM XAV939 (Sigma-Aldrich), and 0.5× ncB27 (Nuwacell Biotechnologies Co., Ltd.). The medium was changed every 2 days.
- When the fusion reached 80%, the cells were passaged at a ratio of 1:6 by trypsin (Sigma-Aldrich).
Immunofluorescence staining
On the 7th and 14th days of differentiation, the culture medium was discarded. The cells were then washed once with Dulbecco’s phosphate buffer saline (DPBS; Sigma-Aldrich) and fixed with 4% paraformaldehyde (Sigma-Aldrich) at room temperature for 15 minutes, washed 3 times with DPBS for 5 minutes each time, permeabilized with 0.3% TritonX-100 (Sigma-Aldrich) at room temperature for 20 minutes, and blocked with 10% goat serum at room temperature for 1 hour. Primary antibody incubation was first performed at room temperature for 30 minutes, kept overnight at 4 ℃, and then rewarmed at room temperature for 30 minutes. The cells were washed 3 times with DPBS for 5 minutes each time, and secondary antibody was added. Cells were then incubated at room temperature in the dark for 1 hour, washed 3 times for 5 minutes each time with DPBS, and 4',6-diamidino-2-phenylindole (DAPI; Sigma-Aldrich) staining solution was applied at room temperature for 10 minutes. The cells were then washed a further 3 times with DPBS for 5 minutes, and double distilled water was added once. Finally, the film was sealed with antifluorescent dampening agent. The primary antibodies used for photography with the laser confocal microscope (Olympus, Tokyo, Japan) were: rabbit anti-SRY-related HMG-box gene 10 (SOX10; 1:500; ab155279, Abcam, Cambridge, England), rabbit anti-β-catenin (1:200; 8480, Cell Signaling, Boston, MA, USA), and rabbit anti-zonula occludens-1 (ZO-1; 1:800; 18-7430, Invitrogen, Carlsbad, CA, USA), and the secondary antibody was goat anti-rabbit immunoglobulin G (IgG) antibody (1:1,000; ab150077, Abcam).
Flow cytometry detection
Differentiated cells from day 7 were digested with 0.25% Trypsin-0.5 mM EDTA (Sigma-Aldrich) into individual cells, and 100,000 single cells were removed, washed with DPBS, and centrifuged in a palm centrifuge. The supernatant was absorbed and discarded, fluorescence-activated single cell sorting (FACS) buffer was added to resuspend the cell pellet, and FITC-p75 (345104, BioLegend, San Diego, CA, USA) and PE-human natural killer-1 (HNK-1) flow antibody (359612, BioLegend) were added. The cells were incubated in the dark at 4 ℃ for 20 minutes, washed with DPBS once, and FACS buffer was added. Flow cytometry was then performed (FACSCalibur, BD Biosciences, Franklin Lakes, NJ, USA).
Identification by quantitative reverse transcription polymerase chain reaction (qRT-PCR)
RNA was extracted from cells differentiating on days 7 and 14 using TRIzol reagent (Invitrogen) in accordance with the manufacturer’s protocol. Reverse transcription with the TaqMan Reverse Transcription Kit (Applied Biosystems, Foster City, CA, USA) was performed using 1 µg of template RNA. QRT-PCR analysis was performed according to the manufacturer’s protocol using SYBR Green Mastermix and the 7500 real-time PCR System (Applied Biosystems, Carlsbad, CA, USA). Primers identified by qRT-PCR were NCC-specific primers [SOX9, SOX10, nerve growth factor receptor (NGFR), HNK-1, and β-catenin], CEC-specific primers [collagen type IV alpha 1 (COL4A1), COL8A1, COL8A2, and ZO-1] with retinal pigment epithelial cell-specific primers (RPE65) (Table 1).
Table 1
Cell type | Primer | Forward sequences | Reverse sequences |
---|---|---|---|
NCC | SOX9 | GCCAGGTGCTCAAAGGCTA | TCTCGTTCAGAAGTCTCCAGAG |
SOX10 | CACAAGAAAGACCACCCGGA | AAGTGGGCGCTCTTGTAGTG | |
NGFR | AAGAAAAGTGGGCCAGTGTG | AACAGTCCTTTGCAGGGTTG | |
HNK-1 | AATGGGTGGGTTGTGAGTGC | GCCAGACAGTGATGAGCAGA | |
β-catenin | GACGGAGGAAGGTCTGAGGA | TGGCCATGTCCAACTCCATC | |
CEC | COL4A1 | GGCAGATTCGGACCACTAGG | GGGCCATTTCCGTGGTTTCT |
COL8A1 | CCTGGGTCAGCAAGTACCTC | TTGTTCCCCTCGTAAACTGG | |
COL8A2 | ATCGCTGGGGACTCTGACAC | GGTAGAGGCATTTCCAGGTACT | |
ZO-1 | ACCAGTAAGTCGTCCTGATCC | TCGGCCAAATCTTCTCACTCC | |
RPE | RPE65 | GCATCCTGCTGGTGGTTACA | TTCAAAGAGTCCTGGCCCAC |
qRT-PCR, quantitative reverse transcription polymerase chain reaction; NCC, neural crest cell; CEC, corneal endothelial cell; RPE, retinal pigment epithelium; SOX10, SRY-related HMG-box gene 10; NGFR, nerve growth factor receptor; HNK-1, human natural killer-1; COL4A1, collagen type IV alpha 1; ZO-1, zonula occludens-1.
Statistical analysis
QRT-PCR was performed for three parallel assays. The obtained data were statistically analyzed by SPSS 17.0, and the results are expressed as mean ± standard deviation (SD). The differences between the two groups were examined by the Student’s t-test. P<0.05 was considered statistically significant.
Results
Differentiation of hiPSC into NCC
During development, CECs originate from NCCs. NCCs are a pluripotent progenitor cell population that are transiently present during development and can differentiate into many cell types, including neuronal cells, colloid cells, connective tissue, bone, CECs, corneal stromal cells, and other anterior segment cells. Therefore, our strategy involved first inducing the differentiation of hiPSCs into NCCs using two small-molecule compounds, CHIR99021 [glycogen synthase kinase 3 (GSK-3) inhibitor, Wnt pathway activator] and SB431542 (TGF-β/SMAD pathway inhibitor), based on a previously published induction protocol by Menendez et al. (11).
HiPSCs were seeded in Matrigel-coated 6-well plates, and the fusion degree reached about 30% to induce differentiation. The cells gradually lost the morphology of monoclonal hiPSCs, switching from cells with a high nucleocytoplasmic ratio to cells with a shuttle shape. Differentiation on day 7 was induced, cell confluence reached 100%, there were a large number of bulging cells between the cell pellets, and cell morphology was significantly changed (Figure 1A). Immunofluorescence staining for β-catenin, a positive correlation protein of the Wnt pathway, along with SOX10 staining, an indicator of NCC specificity, demonstrated that about 60% of the cells were positive for SOX10 expression (Figure 1B). QRT-PCR results demonstrated that cells harvested from induced differentiation on day 7 significantly expressed neural crest specific parameters (SOX9, SOX10, NGFR, HNK-1, and β-catenin) compared with undifferentiated hiPSCs (Figure 1C). The results of flow cytometry demonstrated that more than 80% of cells expressed p75/NGFR with HNK-1 protein (Figure 1D). These results indicated that cells harvested from day 7 of hiPSC-induced differentiation were NCCs in response to neural crest inducing differentiation medium.
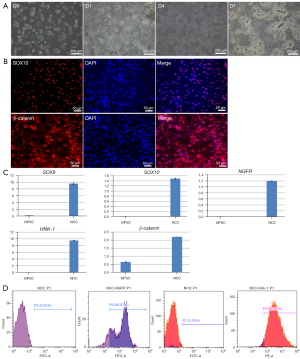
Differentiation into CEC induced by NCC
As described in a number of previous induction protocols (12-14), neural growth supplement (B27), endothelial mitogen (PDGF-BB), and FGF were added to the CEC culture medium. In addition, several studies (12-14) have reported that dickkopf-related protein 2 (DKK 2), a Wnt pathway inhibitor, is involved in NCC-induced differentiation to CEC. Our experiments confirmed that inhibition of the Wnt pathway was involved in NCC-induced differentiation to CEC, and the Wnt pathway inhibitor XAV939 was added to the medium, rather than DKK 2. XAV939 is a small-molecule Wnt signaling pathway inhibitor that selectively inhibits β-catenin-mediated transcription and inhibits the Wnt signaling pathway by stabilizing axin (15).
NCCs were revived and seeded in Matrigel-coated well plates and changed to CEC02 medium after 24 hours of culture in CEC01 medium. On differentiation day 11, the cells no longer demonstrated an elongated morphology of NCC (the cell morphology became flat), and on differentiation day 14, irregular hexagonal cells with tight junction structures could be observed (Figure 2A). The hexagonal cells of tight junctions were identified by immunofluorescence staining with qRT-PCR, and the cells were ZO-1-positive cells, with positive cells accounting for about 90% (Figure 2B). QRT-PCR analysis of specific genes (COL4A1, COL8A1, COL8A2, and ZO-1) expressed early in the corneal endothelium of the eye demonstrated that COL8A1 and COL8A2 expression increased nearly 10- to 100-fold, respectively, compared with undifferentiated hiPSCs. Furthermore, RPE65, a specific indicator of retinal pigment epithelial cells, was not expressed (Figure 2C). The results of immunofluorescence and qRT-PCR demonstrated that the CECs were harvested from hiPSC-induced differentiation on day 14 in the presence of corneal endothelium-inducing differentiation medium.
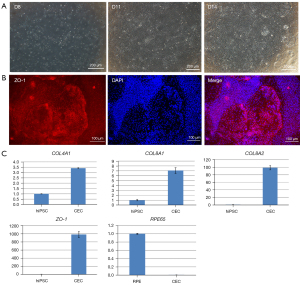
Discussion
During eye development, when the surface ectoderm and lens vesicle are separated, there are periocular cells that migrate and differentiate from NCCs, with the interocular cells differentiating into a variety of cells, including CECs, which remain in the G1 phase of the cell cycle (16). This process may occur in response to the TGF-β signaling system and/or the Wnt signaling system (17,18). Since the corneal endothelium is located between the corneal stroma and the lens and develops after the lens, CECs may be produced under the influence of lens epithelial cells and corneal stromal cells. Zhang et al. (10) exposed human ESCs to corneal stromal cells to stimulate the differentiation of corneal stromal cells into ocular cells. NCCs were placed in lens epithelial cell-conditioned medium for co-culture to derive corneal endothelial-like cells from human ESC. Na+/K+-ATPase alpha-1 and beta-1 subunits were upregulated in these corneal endothelial-like cells. Similarly, in a study by Chen et al. (19), mouse ESC and iPSC differentiation into CEC was induced using an embryonic development process, in which all-trans retinoic acid (RA) was added to the culture medium in the first step, followed by a second induction in conditioned culture of lens epithelial cells, leading to the generation of CEC-like cells. RA is an active product of fat-soluble vitamin A, which plays an important role in embryonic development. Selection of effective all-trans RA concentrations could upregulate interepithelial transition-related transcription factors and NNC marker gene expression (20,21). It has also been shown that (22) RA and GSK 3β inhibitors (Wnt/β-catenin signaling activators) can upregulate the expression of paired-like homeodomain 2 (Pitx2), a homeobox gene involved in anterior segment development. In addition to lens epithelial conditioned medium, Song et al. (23) used bovine corneal endothelial conditioned medium to induce differentiation of NCC induced by embryonic stem cells and obtained human corneal endothelial-like cells after 40 days of induced differentiation culture. These studies verify that the corneal endothelium is differentiated from NCC (10,16-23). Moreover, the components in the conditioned medium of lens epithelial cells or corneal stromal cells are capable of inducing differentiated CEC (9).
There are some drawbacks to the methods mentioned above, including unclear medium composition, long differentiation time, and low differentiation rate (24). Therefore, the use of culture methods with clear components has become an important research direction for CEC differentiation in vitro. This method is beneficial for revealing the regulatory mechanisms of embryonic development, and the induction of differentiation protocols through chemically well-defined media is simpler, more time-efficient, and does not require additional human corneal donors. Studies have shown that TGF-β, bone morphogenetic protein, and Wnt signaling pathways play important roles in NCC and corneal endothelial development (9,24-27). Thus, current methods of inducing differentiation mostly focus on the above signaling pathways. The corneal endothelial induction method proposed by McCabe et al. (13) is a two-step generation process; the first step is to induce differentiation from ESC to NCC with a TGF-β signaling blocker (SB431542) and Noggin (BMP inhibitor). As the TGF-β-SMAD-2/3 signaling pathway and the BMP SMAD-1/5/8 signaling pathway are blocked, the process is called “double SMAD inhibition”. Menendez et al. (11) induced NCCs positive for the NCC markers p75/NGFR and SOX10 using two small-molecule compounds, CHIR99021 (GSK-3 inhibitor) and SB431542 (TGF-β/SMAD pathway inhibitor). The cell morphology altered from high nucleocytoplasmic ratios to elongated structures with neuronal morphology, with 80–99% differentiation. In the first step of the study, NCCs were induced within 7 days and expressed NCC-specific markers from Menendez et al.’s induction method. Among them, β-catenin protein is an indicator of Wnt canonical pathway activation during neural crest formation (28). SOX10 protein was also produced by Wnt pathway regulation during NCC formation.
In the second step of differentiation from NCC to CEC, McCabe et al. (13) induced differentiation of corneal endothelial-like cells with a hexagonal structure from NCCs by inducers PDGF-B, DKK 2, and basic FGF. Previous studies have varied from 14–25 days for differentiation from hiPSC-NCC to CEC (12,14). DKK 2 is an antagonist of Wnt/β-catenin signaling, and it could play an important role in normal ocular development (29). The role of Wnt/β-catenin signaling in differentiation from NCC to CEC was confirmed in our study by replacing DKK 2 with another antagonist of Wnt/β-catenin signaling, XAV939. XAV939, described in a study in Nature in 2009 (15), is a novel small-molecule Wnt signaling pathway inhibitor that selectively inhibits β-catenin-mediated transcription and inhibits the Wnt signaling pathway by stabilizing axin. HiPSC-NCCs were differentiated for 7 days to produce CECs and showed high expression of CEC-associated markers. ZO-1 is a tight junction protein on the endothelial cell membrane, and collagen type 4 and type 8 are components of Descemet’s (30) and are secreted by endothelial cells. The assay results demonstrated that the use of XAV939 instead of DKK 2 induced differentiation of NCC into CEC, with an induced differentiation time of 7 days, which was shorter than that in previous studies (12,14).
In terms of in vivo tests, Japanese scholars (31) successfully injected allogeneic CECs expanded in vitro into the anterior chamber of patients and observed them for 24 weeks, finding that the endothelial cell count increased, the corneal thickness decreased, and visual acuity improved. This was the first demonstration that anterior chamber injection with primary cultured endothelial cells could maintain corneal transparency. In terms of an in vivo test of CECs induced by PSCs, in 2017 Shen et al. (32) cultured skin-derived precursors and B4G12 cells (an immortalized human endothelial cell) together to obtain CEC-like cells and then injected them into the anterior chamber of experimental monkeys with corneal endothelial decompensation. The cornea gradually became transparent 7 days after surgery, and the cornea remained transparent during 3 months of follow-up. Gong et al. (33) published a study in 2021 in which differentiation of hiPSC into NCC was induced by CHIR99021 and SB431542. NCCs were injected into the anterior chamber of model rabbits with corneal endothelial decompensation, with the results showing that the rabbit cornea gradually became transparent 7 days after injection. However, the effect disappeared 14 days after injection. The reappearance of corneal edema may be associated with improper maturation, senescence, and interepithelial transition of hiPSC-CEC (33). Therefore, on the basis that the mechanism of differentiation from NCC into CEC has not been fully clarified, there is still no optimal method for differentiation induced by small molecules or recombinant proteins in vitro (25). Further study of the function and stability of endothelial cells induced by stem cells with clear chemical composition in vitro, as well as the medium composition and steps of in vitro culture, is needed.
In our study, we used a differentiation method with clear chemical composition and no serum or serum substitutes to successfully induce hiPSCs to differentiate into NCCs (day 7) with high expression of β-catenin, p75, and HNK-1 protein. CECs expressing CEC-specific indicators COL4A1, COL8A1, COL8A2, and ZO-1 could be obtained by continuing differentiation for 5 to 7 days. In the future, further optimization of protocols for the differentiation of stem cells into CECs in vitro is required to keep the induced endothelial-like cells more stable in morphology and function. Studies on endothelial cell function in vitro, as well as in vivo animal experiments, are required to evaluate safety and efficacy.
Acknowledgments
Special thanks to Mr. Zhiqiang Xin, Ms. Caiyun Wang from Nuwacell Biotechnologies Co., Ltd. for their assistance in this study.
Funding: This study was supported by the grant from the National Key R&D Program of China (No. 2019YFA0110704) (Beijing, China) and The National Natural Science Foundation of China (No. 81770887).
Footnote
Reporting Checklist: The authors have completed the MDAR reporting checklist. Available at https://atm.amegroups.com/article/view/10.21037/atm-22-1586/rc
Data Sharing Statement: Available at https://atm.amegroups.com/article/view/10.21037/atm-22-1586/dss
Conflicts of Interest: All authors have completed the ICMJE uniform disclosure form (available at https://atm.amegroups.com/article/view/10.21037/atm-22-1586/coif). The authors have no conflicts of interest to declare.
Ethical Statement: The authors are accountable for all aspects of the work in ensuring that questions related to the accuracy or integrity of any part of the work are appropriately investigated and resolved.
Open Access Statement: This is an Open Access article distributed in accordance with the Creative Commons Attribution-NonCommercial-NoDerivs 4.0 International License (CC BY-NC-ND 4.0), which permits the non-commercial replication and distribution of the article with the strict proviso that no changes or edits are made and the original work is properly cited (including links to both the formal publication through the relevant DOI and the license). See: https://creativecommons.org/licenses/by-nc-nd/4.0/.
References
- DelMonte DW, Kim T. Anatomy and physiology of the cornea. J Cataract Refract Surg 2011;37:588-98. [Crossref] [PubMed]
- Bonanno JA. Molecular mechanisms underlying the corneal endothelial pump. Exp Eye Res 2012;95:2-7. [Crossref] [PubMed]
- Tuft SJ, Coster DJ. The corneal endothelium. Eye (Lond) 1990;4:389-424. [Crossref] [PubMed]
- Gain P, Jullienne R, He Z, et al. Global Survey of Corneal Transplantation and Eye Banking. JAMA Ophthalmol 2016;134:167-73. [Crossref] [PubMed]
- Chen S, Zhu Q, Sun H, et al. Advances in culture, expansion and mechanistic studies of corneal endothelial cells: a systematic review. J Biomed Sci 2019;26:2. [Crossref] [PubMed]
- Okumura N, Ueno M, Koizumi N, et al. Enhancement on primate corneal endothelial cell survival in vitro by a ROCK inhibitor. Invest Ophthalmol Vis Sci 2009;50:3680-7. [Crossref] [PubMed]
- Peh GS, Toh KP, Wu FY, et al. Cultivation of human corneal endothelial cells isolated from paired donor corneas. PLoS One 2011;6:e28310. [Crossref] [PubMed]
- Okumura N, Kay EP, Nakahara M, et al. Inhibition of TGF-β signaling enables human corneal endothelial cell expansion in vitro for use in regenerative medicine. PLoS One 2013;8:e58000. [Crossref] [PubMed]
- Hatou S, Shimmura S. Review: corneal endothelial cell derivation methods from ES/iPS cells. Inflamm Regen 2019;39:19. [Crossref] [PubMed]
- Zhang K, Pang K, Wu X. Isolation and transplantation of corneal endothelial cell-like cells derived from in-vitro-differentiated human embryonic stem cells. Stem Cells Dev 2014;23:1340-54. [Crossref] [PubMed]
- Menendez L, Kulik MJ, Page AT, et al. Directed differentiation of human pluripotent cells to neural crest stem cells. Nat Protoc 2013;8:203-12. [Crossref] [PubMed]
- Wagoner MD, Bohrer LR, Aldrich BT, et al. Feeder-free differentiation of cells exhibiting characteristics of corneal endothelium from human induced pluripotent stem cells. Biol Open 2018;7:bio032102. [Crossref] [PubMed]
- McCabe KL, Kunzevitzky NJ, Chiswell BP, et al. Efficient Generation of Human Embryonic Stem Cell-Derived Corneal Endothelial Cells by Directed Differentiation. PLoS One 2015;10:e0145266. [Crossref] [PubMed]
- Ali M, Khan SY, Vasanth S, et al. Generation and Proteome Profiling of PBMC-Originated, iPSC-Derived Corneal Endothelial Cells. Invest Ophthalmol Vis Sci 2018;59:2437-44. [Crossref] [PubMed]
- Huang SM, Mishina YM, Liu S, et al. Tankyrase inhibition stabilizes axin and antagonizes Wnt signalling. Nature 2009;461:614-20. [Crossref] [PubMed]
- Zavala J, López Jaime GR, Rodríguez Barrientos CA, et al. Corneal endothelium: developmental strategies for regeneration. Eye (Lond) 2013;27:579-88. [Crossref] [PubMed]
- Saika S, Saika S, Liu CY, et al. TGFbeta2 in corneal morphogenesis during mouse embryonic development. Dev Biol 2001;240:419-32. [Crossref] [PubMed]
- Zacharias AL, Gage PJ. Canonical Wnt/β-catenin signaling is required for maintenance but not activation of Pitx2 expression in neural crest during eye development. Dev Dyn 2010;239:3215-25. [Crossref] [PubMed]
- Chen P, Chen JZ, Shao CY, et al. Treatment with retinoic acid and lens epithelial cell-conditioned medium in vitro directed the differentiation of pluripotent stem cells towards corneal endothelial cell-like cells. Exp Ther Med 2015;9:351-60. [Crossref] [PubMed]
- Prasad MS, Sauka-Spengler T, LaBonne C. Induction of the neural crest state: control of stem cell attributes by gene regulatory, post-transcriptional and epigenetic interactions. Dev Biol 2012;366:10-21. [Crossref] [PubMed]
- Strobl-Mazzulla PH, Bronner ME. Epithelial to mesenchymal transition: new and old insights from the classical neural crest model. Semin Cancer Biol 2012;22:411-6. [Crossref] [PubMed]
- Hatou S, Yoshida S, Higa K, et al. Functional corneal endothelium derived from corneal stroma stem cells of neural crest origin by retinoic acid and Wnt/β-catenin signaling. Stem Cells Dev 2013;22:828-39. [Crossref] [PubMed]
- Song Q, Yuan S, An Q, et al. Directed differentiation of human embryonic stem cells to corneal endothelial cell-like cells: A transcriptomic analysis. Exp Eye Res 2016;151:107-14. [Crossref] [PubMed]
- Chambers SM, Fasano CA, Papapetrou EP, et al. Highly efficient neural conversion of human ES and iPS cells by dual inhibition of SMAD signaling. Nat Biotechnol 2009;27:275-80. [Crossref] [PubMed]
- Hackland JOS, Frith TJR, Thompson O, et al. Top-Down Inhibition of BMP Signaling Enables Robust Induction of hPSCs Into Neural Crest in Fully Defined, Xeno-free Conditions. Stem Cell Reports 2017;9:1043-52. [Crossref] [PubMed]
- Kim HM, Noh HB, Lee SH, et al. Fine-tuning of dual-SMAD inhibition to differentiate human pluripotent stem cells into neural crest stem cells. Cell Prolif 2021;54:e13103. [Crossref] [PubMed]
- Zhang W, Chen J, Fu Y, et al. The signaling pathway involved in the proliferation of corneal endothelial cells. J Recept Signal Transduct Res 2015;35:585-91. [PubMed]
- Zhao JJ, Afshari NA. Generation of Human Corneal Endothelial Cells via In Vitro Ocular Lineage Restriction of Pluripotent Stem Cells. Invest Ophthalmol Vis Sci 2016;57:6878-84. [Crossref] [PubMed]
- Gage PJ, Qian M, Wu D, et al. The canonical Wnt signaling antagonist DKK2 is an essential effector of PITX2 function during normal eye development. Dev Biol 2008;317:310-24. [Crossref] [PubMed]
- Fitch JM, Birk DE, Linsenmayer C, et al. The spatial organization of Descemet's membrane-associated type IV collagen in the avian cornea. J Cell Biol 1990;110:1457-68. [Crossref] [PubMed]
- Kinoshita S, Koizumi N, Ueno M, et al. Injection of Cultured Cells with a ROCK Inhibitor for Bullous Keratopathy. N Engl J Med 2018;378:995-1003. [Crossref] [PubMed]
- Shen L, Sun P, Zhang C, et al. Therapy of corneal endothelial dysfunction with corneal endothelial cell-like cells derived from skin-derived precursors. Sci Rep 2017;7:13400. [Crossref] [PubMed]
- Gong Y, Duan H, Wang X, et al. Transplantation of human induced pluripotent stem cell-derived neural crest cells for corneal endothelial regeneration. Stem Cell Res Ther 2021;12:214. [Crossref] [PubMed]
(English Language Editor: A. Muijlwijk)