In vitro reactivity (“organ chamber”) of guinea pig tracheal rings—methodology considerations
Introduction
This review was designed and conducted to, briefly and precisely, describe the “organ chambers” techniques for in vitro experimentation on isolated trachea preparations. The “organ chambers” experiments and protocols are usually complex and diverse, and technical publications are scarce. One of the best, if not the best, publication about in vitro methods to study pharmacological airway properties is authored by Fedan and colleagues [2001] (1). Thus, this study became the “master” text of this review, combined with the Hall paper about the airway pharmacology (2), Moreover, only the “organ chambers” preparations that we used in our laboratory will be highlighted.
The present text was motivated by the difficulties faced by our postgraduate students when using airways studies protocols and will take into consideration the three mechanisms of relaxation: (I) guanosine 3,5-cyclic monophosphate (cGMP)/NO-dependent; (II) adenosine 3, 5-cyclic monophosphate (cAMP)/PGI2-dependent; and (III) hyperpolarization-dependent.
The pharmacological basis
Mechanisms of airway smooth muscle (ASM) relaxation
The cGMP/NO pathway
The cGMP and cyclic cAMP are second messengers capable of mediating the actions of a variety of drugs and hormones. There is a large body of evidence supporting the hypothesis that cAMP is the main airways mediator of the ASM drugs relaxant effects, such as alpha 2-adrenoceptor agonists (3). Although most attention has been focused on cAMP signal transduction pathways, recent evidence suggests that cGMP pathway is also an important second messenger with important relaxant and antiproliferative effects (3). In vitro and in vivo studies shows, in agreement with the cGMP role, that nitric oxide (NO) relaxes ASM with intermediate potency between the alpha-adrenoceptor agonist isoprenaline and the phosphodiesterase (PDE) inhibitor theophylline. In addition, epithelium-derived relaxing factor (EpDRF), an NO or NO-like substance, is an ASM relaxant in some species.
As already mentioned, agents that elevate cGMP levels can relax ASM preparations in organ bath systems. However, the potential importance of cGMP in ASM in vivo relaxant responses (as opposed to vascular) is unclear.
The cAMP pathway
The ASM cell is the primary effector cell governing the control of airway caliber in the human lung. The contractile state of the ASM cell is predominantly influenced by the balance of constrictor and relaxant stimuli. Agents such as histamine and acetylcholine (Ach) cause contraction, activating specific cell surface receptors engaged with signal transduction pathways and ion channels. The predominant contraction pathway is the phospholipase C (PLC) activation with increasing of intracellular calcium levels. Relaxation is mediated predominantly by stimulation of adenylyl cyclase-coupled receptors (e.g., the β-adrenoceptor) resulting in elevation of cell cAMP content (Table 1). Complex crosstalk occurs between these pathways and also ion channels expressed on the cell membrane, leading to careful regulation of ASM muscle tone (2).
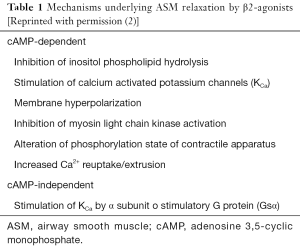
Full table
Hyperpolarization and ASM reactivity
Various types of potassium (K+) channels are expressed by ASM cells, which play a crucial role in determining the resting membrane potential, electrical stability and responsiveness to both contractile and relaxant agents. Moreover, K+ channels are also involved in modulation of neurotransmitter release from airway nerves. The most important K+ channels identified in airways include large and small Ca2+-activated, delayed-rectifier, and ATP-sensitive channels. These K+ channels are structurally and functionally different, thus playing distinct roles in airway electrophysiology and pharmacology. Many in vitro and in vivo studies, performed in both animals and humans, have shown that K+ channel openers can induce hyperpolarization of ASM cells, bronchodilation, suppression of airway hyperresponsiveness (AHR), and inhibition of neural reflexes.
Most of the studies conducted to evaluate the effects of K1 channel opening on ASM contractility have been performed using KATP channel activators; at present, only a few molecules are known to selectively open maxi-KCa channels. In vitro studies have shown that KATP channel activators have some spasmolytic activity on ASM of several different species. In contrast, these drugs do not exert a significant protective effect against the response induced by high concentrations of contractile agonists. Moreover, there are significant differences among species concerning the protective action of KATP channel openers against bronchoconstrictive stimuli. In guinea pig ASM, these compounds show a reduced inhibitory effect on contractile responses elicited by cholinergic agents. In comparison with β-adrenoceptor agonists, KATP channel activators have a less potent in vitro relaxing activity on ASM, suggesting a questionable relevance (4).
Mechanisms of ASM contraction
Pathways involved in ASM contraction are quite similar to those participating in vascular vessels. Following binding of agonist to receptor in the cell membrane, the associated G protein which exists as a heterotrimeric complex of a, b and c subunit dissociates, and the free a subunit stimulates PLC, which in turn catalyses the breakdown of phosphatidylinositol 4,5 biphosphate (PIP2), resulting in the formation of the two intracellular messengers diacylglycerol (DAG) and inositol 1,4,5 triphosphate (IP3). IP3 can release calcium from intracellular stores via the IP3 receptor (IP3R), whereas DAG can stimulate calcium entry in addition to activating protein kinase C. The rise in cytosolic free calcium levels leads to contraction of the ASM. Some other contractile agonists coupled to receptors expressed on ASM are also able to stimulate contractile responses through the same intracellular signaling pathway. These agonists and the receptors through which they operate are shown in Table 2 and Figure 1 (2).
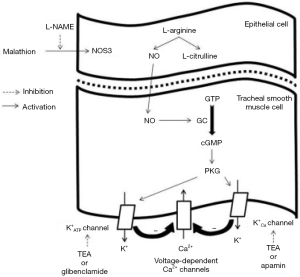
Role of epithelium
The airway epithelium removal affects the contractile and relaxant smooth muscle reactivity under in vitro experiments. Differently from the vascular endothelium, a non-NO and nonprostanoid factor named EpDRF, the airway reactivity is not explained by the epithelium barrier remotion (1). A variety of methods have been used to remove the epithelium, and they have been adapted to the size of the airway being studied. In large airways, for example, rubbing the surface with a cotton-tipped applicator is effective, but other gentle abrasives are equally valid. It is important that the epithelium is removed without damaging the smooth muscle.
The “organ chamber” setup
Muscle strips or tracheal rings are studied in an organ bath (“organ-chamber”) containing a gassed physiological salt solution. We prefer to consider tracheal rings because of their easier instrumental feasibility. Several solutions are used, which differ in the composition and concentrations of the salts and buffer systems employed. A bicarbonate-containing solution requires CO2 gassing to set the pH. The solution widely used is a modified Krebs-Henseleit solution, which consists of (mM): NaCl (113.0), KCl (4.8), CaCl2 (2.5), KH2PO4 (1.2), MgSO4 (1.2), NaHCO3 (25.0), and glucose (5.7), pH 7.4 (37 °C); gassed with 95% O2 + 5% CO2. The temperature is usually kept at 37 °C, but this may vary depending on the body temperature of the animal from which the tissue was derived, or other experimental needs. Allowing an ample equilibration period at 37 °C allows the muscle to generate ATP, reactivate ATP-dependent transport and restore resting membrane potential. This detail is mandatory if the tissue is stored in an iced solution or remains on laboratory room ambient for a long period.
- To measure responses of the smooth muscle preparation, one ligature or wire assembly is attached to a fixed holder at the bottom of the organ bath containing Krebs solution, while the other is attached to a force transducer to measure mechanical responses (i.e., contraction or relaxation) of the smooth muscle (Figures 2,3);
- After set up, the preparation is elongated to place it at a length (Lo) in which it develops maximal contractile force. While monitoring the output of the transducer on the chart recorder or computer screen, the preparations are slowly lengthened to remove slack in the connections between the anchor and the transducer and to reach a predetermined optimum passive or baseline force (i.e., tension);
- Following the application of passive force, a mandatory incubation period of at least 1 h should follow before any experimental intervention begins. The solution should be changed, at regular intervals (15 min), to prevent the buildup of metabolic products. This incubation period is also necessary to allow the muscle to reestablish the normal ion content that goes awry during tissue preparation. If many investigators use a 1 g optimum passive force on a like preparation to establish a baseline, it will not make sense to begin a length–tension study by applying 2 g of force at the outset. It is always important to emphasize that over adjusting the amount of applied force may eventually damage the smooth muscle;
- An agent or procedure that causes contraction of the muscle is needed. The choice can be eased by the pharmacological properties of the preparation as reported in the literature. Histamine or Ach (or analogs of Ach such as methacholine or carbachol) are useful agonists for this purpose (histamine does not contract rat airways). A concentration must be chosen for use and we recommend that it is less than or equal to the EC50 value for the agonist; use of high concentrations of these agents (10−4 M) can result in desensitization of the muscle and spurious results.
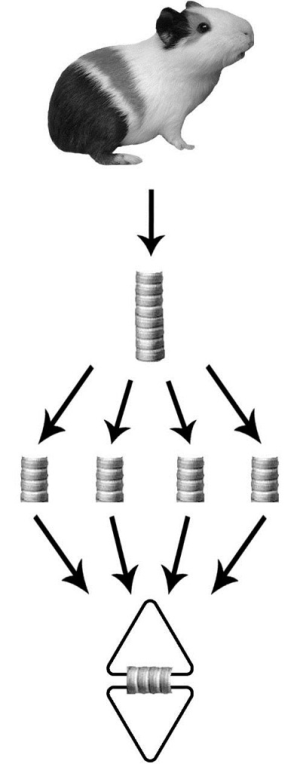
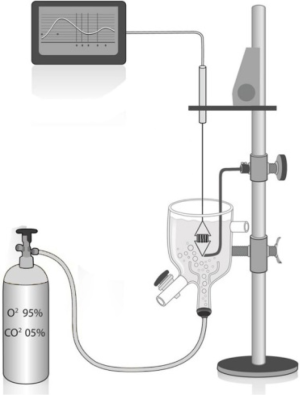
Note: the presence of a spontaneous tone in the preparation may complicate the establishment of basal conditions. The spontaneous tone reflects an intrinsic activation of the smooth muscle, which the investigator has not caused and, therefore, is probably due to the effects of released prostanoids (1).
The “organ chamber” setup is illustrated in Figures 2,3,4. Our protocol is summarized in Table 3.
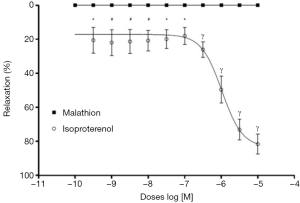

Full table
Acknowledgements
Sao Paulo Research Foundation (FAPESP), National Council of Scientific and Technological Development (CNPq), Coordination of Improvement of Higher Academic Staff (CAPES) Research performed at Laboratory of Cardiovascular and Endothelium Function, Ribeirao Preto School of Medicine, University of Sao Paulo (FMRP-USP), Ribeirao Preto-SP, Brazil. Part of PhD degree thesis, Experimental Medicine Program. Tutor: Paulo Roberto Barbosa Evora.
Footnote
Conflicts of Interest: The authors have no conflicts of interest to declare.
References
- Fedan JS, Van Scott MR, Johnston RA. Pharmacological techniques for the in vitro study of airways. J Pharmacol Toxicol Methods. 2001;45:159-74. [Crossref] [PubMed]
- Hall IP. Second messengers, ion channels and pharmacology of airway smooth muscle. Eur Respir J 2000;15:1120-7. [Crossref] [PubMed]
- Hamad AM, Clayton A, Islam B, et al. Guanylyl cyclases, nitric oxide, natriuretic peptides, and airway smooth muscle function. Am J Physiol Lung Cell Mol Physiol 2003;285:L973-83. [Crossref] [PubMed]
- Pelaia G, Gallelli L, Vatrella A, et al. Potential role of potassium channel openers in the treatment of asthma and chronic obstructive pulmonary disease. Life Sci 2002;70:977-90. [Crossref] [PubMed]
- Vieira AB, Coelho LP, Insuela DB, et al. Mangiferin prevents guinea pig tracheal contraction via activation of the nitric oxide-cyclic GMP pathway. PLoS One 2013;8:e71759. [Crossref] [PubMed]