No association between systemic complement activation and intensive care unit-acquired weakness
Introduction
The pathogenesis of intensive care unit-acquired weakness (ICU-AW) is probably multi-factorial (1). The main risk factors are sepsis, systemic inflammatory response syndrome (SIRS) and multiple organ dysfunction syndrome (MODS) (1). Activation of the complement system plays an important role in these risk factors and is associated with increased occurrence of shock and fatal outcomes in sepsis (2-4). Complement activation also plays a role in the pathogenesis of acute inflammatory polyneuropathies and myopathies (5-7). Thus, muscle and nerve damage in ICU-AW might also be complement-mediated.
Complement activation may lead to ICU-AW by anaphylatoxins (C3a, C4a, C5a), which can induce unbalanced systemic and local inflammatory responses, leading to MODS (8,9) and probably to ‘failure’ of muscles and nerves. C5a can also increase vascular permeability, leading to tissue edema and possibly nerve and muscle tissue damage (9). The final pathway of complement activation results in the membrane attack complex (MAC), causing direct cell damage (9). MAC depositions have been found in muscles of patients with ICU-AW (10-12).
In this pilot study, we tested the hypothesis that patients who develop ICU-AW have increased systemic complement activation compared to critically ill patients who do not develop ICU-AW.
Methods
This was a sub-study of a prospective observational cohort study (BASIC study, Biomarker Analysis in Septic Intensive Care patients), performed on the mixed medical-surgical intensive care unit (ICU) of the Academic Medical Center Amsterdam. The institutional review board approved the BASIC study protocol (No. NL34294.018.10). Informed consent from patients or their legal representatives was obtained before study participation.
Patients newly admitted to the ICU having sepsis or SIRS [Bone criteria (13)], mechanically ventilated for ≥48 hours, and in whom muscle strength assessment was performed, were eligible for inclusion. Exclusion criteria included antibiotic treatment for >48 hours, expected ICU stay <24 hours, no informed consent within 24 hours after ICU admission, pre-existing poor functional status [Modified Rankin score ≥4 (14)] and any central nervous system disorder, spinal cord injury or neuromuscular disorder as reason for ICU admission.
Blood samples were collected as soon as possible after ICU admission, and thereafter daily (about 3:00 PM) for 6 consecutive days. Blood was collected in vacutainer tubes, containing an inhibitor mix (with final concentrations of 10 mM benzamidine, 100 µg/mL soy bean trypsin inhibitor and 10 mM ethylene-diamine-tetra-acid) to prevent in vitro complement activation. Samples were centrifuged (1,500 g, 15 min, room temperature) within 1 hour after collection and plasma was stored in aliquots at −80 °C until assayed.
To determine complement activation of the common pathway and initial classical/lectin or alternative pathway, plasma levels of complement activation products C3b/c and C4b/c were measured using previously described enzyme-linked immunosorbent assays (ELISAs) (Sanquin, Amsterdam, The Netherlands) (15,16). These ELISAs do not distinguish C3b from C3bi and C3c, and C4b from C4bi and C4c and are therefore referred to as C3b/c and C4b/c. The normal reference values (from local healthy controls) are <57 nmol/L for C3b/c and <8 nmol/L for C4b/c.
Further downstream complement activation was assessed by measuring levels of C5a (no reference value available), using a commercial ELISA kit (MicroVue, Quidel, San Diego, CA, USA).
All measurements were done batch-wise and in duplo. Samples were analyzed blinded to all patients’ data. Measurements with a coefficient of variation (CV) value of >30% were excluded from the analysis. The ELISA was successfully performed (CV of <30%) in 99% of C3b/c measurements, 98% of C4b/c measurements and 100% of C5a measurements. The lower limit of detection [determined by the mean of blanks plus 3 times the standard deviation (SD) of the blanks] for the C3b/c, C4b/c and C5a assays were 0.001 nmol/L, 0.002 nmol/L and 0.004 ng/mL, respectively.
Manual muscle strength was assessed as soon as patients were awake and attentive. Using the Medical Research Council (MRC) scale, six muscle groups were tested, bilaterally. ICU-AW was defined by a mean MRC score <4 (17,18).
The following clinical characteristics were collected: age, gender, admission reason, presence of sepsis at admission, length of stay on the ICU, number of days with mechanical ventilation, days from admission to muscle strength assessment, ICU mortality, Acute Physiology and Chronic Health Evaluation IV (APACHE IV) score and Sequential Organ Failure Assessment (SOFA) scores at days of blood sampling.
This study is an exploratory pilot study. Therefore, no formal power calculation was performed.
Mean values are presented with SD, median values with interquartile range (IQR) and proportions with total numbers and percentages. Differences between proportions were assessed using Fisher’s exact test, between normally distributed continuous variables using Welch’s t-test and between non-normally distributed continuous variables using Mann-Whitney U test.
To assess our primary endpoint, the difference between systemic complement activation and the presence of ICU-AW, and to account for repeated measurements we used summary statistics, which included: admission complement levels, maximum, minimum and mean values per patient during the first 7 days in ICU. A P value <0.05 was considered statistically significant (P<0.004 after Bonferroni correction). Analyses were done using R (version: 3.0.2).
Results
Data and plasma samples of 27 patients were available; 13 patients who developed ICU-AW and 14 patients who did not develop ICU-AW. Patient characteristics are presented in Table 1. A total of 167 plasma samples were analyzed (median of seven samples/patients). The median time from ICU admission to the first sample was 15.8 hours (IQR, 12.8–22.5 hours) in the ICU-AW group versus 17.3 hours (IQR, 14.1–22.2 hours) in the no ICU-AW group (P=0.74).
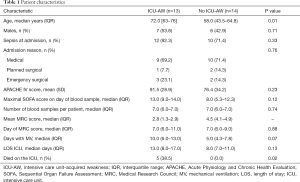
Full table
Levels of C3b/c, C4b/c and C5a fluctuated considerably in individual patients during the first 7 days in ICU. Median levels and IQR of C3b/c, C4b/c and C5a at each time point are presented in Figure 1.
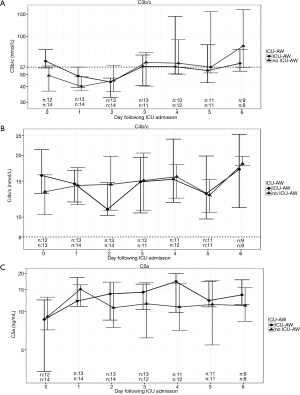
There was no difference in admission, maximum, minimum or mean levels of C3b/c, C4b/c or C5a between patients who developed and did not develop ICU-AW (Table 2).
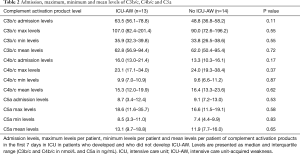
Full table
Discussion
This pilot study shows no difference in systemic complement activation in the first 7 days after ICU admission between patients who did and who did not develop ICU-AW.
All patients showed increased complement activation, as shown by C4b/c levels of nearly twice the reference value. The in- and exclusion criteria were rather strict and it is likely that we have selected a severely ill subpopulation with a high inflammatory state at admission. The severity of illness in the ICU-AW and no ICUAW group were comparable; both groups had high APACHE IV and SOFA scores.
Complement levels were lower than previously described in a study with patients with severe sepsis and septic shock (19), possibly due to a different case mix: not all patients in our cohort had sepsis, and patients might have died earlier in other cohorts, before muscle strength could have been measured.
Although we did not find a difference in complement activation, activated complement can still play a role in the pathophysiology of ICU-AW in the presence of another yet unknown factor, for example expression of membrane complement regulatory proteins (the sensitivity to complement-mediated injury) (20).
The difference in systemic complement levels between patients with and without development of ICU-AW has never been studied before. Previously, no correlation has been found between plasma C3 and C4 levels and compound muscle action potential (CMAP) amplitudes of three nerves in ICU patients, but muscle strength was not measured in this study (21).
The use of daily measurements is a strength of this study because it enabled us to investigate the time course of complement activation and to use summary statistics, such as maximum levels.
This study has some limitations. The sample size of this pilot study was small, limiting the robustness of our results. Furthermore, it was impossible to determine the exact moment at which the inflammatory process was triggered in individual patients, since the onset of this process may take place before ICU admission (22). As complement activation occurs very early in the inflammatory response, peaks of complement activation within the first hours after ICU admission may be missed. Hemodilution may also have decreased complement levels (15), but this is a difficult factor to correct for. Furthermore, it can be debated whether plasma levels of complement activation products, indicating systemic activation, adequately reflect the levels in muscle or nerve tissue, since complement can also be activated locally (12).
Muscle strength assessment by MRC is the recommended test for diagnosing ICU-AW (18,23). A diagnosis of ICU-AW by MRC is often delayed due to impaired consciousness. Therefore, the moment at which ICU-AW developed is unknown. ICU-AW may develop very early, because electrophysiological signs of ICU-AW have been found already within 3 days after ICU admission (24). We did not perform electrophysiological investigations. Therefore it is unknown if patients had electrophysiological alterations at the time the blood samples were taken. Finally, muscle strength might have returned to normal at the time patients woke up, because early detected electrophysiological alterations can be rapidly reversible (25).
Conclusions
This pilot study shows that systemic complement levels are not different between patients with or without ICU-AW.
Acknowledgements
We thank Gerard van Mierlo from the department of immunopathology, Sanquin, Amsterdam for his technical assistance with the complement assays; and members of the BASIC study group: Friso M. de Beer, Lieuwe D. J. Bos, Gerie J. Glas, Roosmarijn T. M. van Hooijdonk, Janneke Horn, Tom van der Poll, Laura R. A. Schouten, Marcus J. Schultz, Marleen Straat, Lonneke A. van Vught, Luuk Wieske, Maryse A. Wiewel, Esther Witteveen.
Funding: This research was performed within the framework of CTMM, the Center for Translational Molecular Medicine (www.ctmm.nl), project MARS (grant 04I-201). L Wieske is supported by a personal grant from the Netherlands Organization for Health Research and Development [ZonMw-AGIKO grant (project number 40-00703-98-11636)].
Footnote
Conflicts of Interest: Prof. IN van Schaik received departmental honoraria for serving on scientific advisory boards and a steering committee for CSL-Behring. The other authors have no conflicts of interest to declare.
Ethical Statement: The institutional review board approved the BASIC study protocol (No. NL34294.018.10). Informed consent from patients or their legal representatives was obtained before study participation.
References
- Latronico N, Bolton CF. Critical illness polyneuropathy and myopathy: a major cause of muscle weakness and paralysis. Lancet Neurol 2011;10:931-41. [Crossref] [PubMed]
- Rittirsch D, Flierl MA, Ward PA. Harmful molecular mechanisms in sepsis. Nat Rev Immunol 2008;8:776-87. [Crossref] [PubMed]
- Hack CE, Nuijens JH, Felt-Bersma RJ, et al. Elevated plasma levels of the anaphylatoxins C3a and C4a are associated with a fatal outcome in sepsis. Am J Med 1989;86:20-6. [Crossref] [PubMed]
- Stöve S, Welte T, Wagner TO, et al. Circulating complement proteins in patients with sepsis or systemic inflammatory response syndrome. Clin Diagn Lab Immunol 1996;3:175-83. [PubMed]
- Ramaglia V, Tannemaat MR, de Kok M, et al. Complement inhibition accelerates regeneration in a model of peripheral nerve injury. Mol Immunol 2009;47:302-9. [Crossref] [PubMed]
- Hafer-Macko C, Hsieh ST, Li CY, et al. Acute motor axonal neuropathy: an antibody-mediated attack on axolemma. Ann Neurol 1996;40:635-44. [Crossref] [PubMed]
- Dalakas MC. Review: An update on inflammatory and autoimmune myopathies. Neuropathol Appl Neurobiol 2011;37:226-42. [Crossref] [PubMed]
- Zhou W. The new face of anaphylatoxins in immune regulation. Immunobiology 2012;217:225-34. [Crossref] [PubMed]
- Flierl MA, Schreiber H, Huber-Lang MS. The role of complement, C5a and its receptors in sepsis and multiorgan dysfunction syndrome. J Invest Surg 2006;19:255-65. [Crossref] [PubMed]
- Bazzi P, Moggio M, Prelle A, et al. Critically ill patients: immunological evidence of inflammation in muscle biopsy. Clin Neuropathol 1999;18:23-30. [PubMed]
- Bednarik J, Lukas Z, Vondracek P. Critical illness polyneuromyopathy: the electrophysiological components of a complex entity. Intensive Care Med 2003;29:1505-14. [Crossref] [PubMed]
- De Letter MA, van Doorn PA, Savelkoul HF, et al. Critical illness polyneuropathy and myopathy (CIPNM): evidence for local immune activation by cytokine-expression in the muscle tissue. J Neuroimmunol 2000;106:206-13. [Crossref] [PubMed]
- Bone RC, Balk RA, Cerra FB, et al. Definitions for sepsis and organ failure and guidelines for the use of innovative therapies in sepsis. Chest 1992;101:1644-55. [Crossref] [PubMed]
- van Swieten JC, Koudstaal P, Visser MC, et al. Interobserver agreement for the assessment of handicap in stroke patients. Stroke 1988;19:604-7. [Crossref] [PubMed]
- Bruins P, te Velthuis H, Yazdanbakhsh AP, et al. Activation of the complement system during and after cardiopulmonary bypass surgery: postsurgery activation involves c-reactive protein and is associated with postoperative arrhythmia. Circulation 1997;96:3542-8. [Crossref] [PubMed]
- Wolbink GJ, Bollen J, Baars JW, et al. Application of a monoclonal antibody against a neoepitope on activated C4 in an ELISA for the quantification of complement activation via the classical pathway. J Immunol Methods 1993;163:67-76. [Crossref] [PubMed]
- Stevens RD, Marshall SA, Cornblath DR, et al. A framework for diagnosing and classifying intensive care unit-acquired weakness. Crit Care Med 2009;37:S299-308. [Crossref] [PubMed]
- Fan E, Cheek F, Chlan L, et al. An Official American Thoracic Society Clinical Practice Guideline: The Diagnosis of Intensive Care Unit-acquired Weakness in Adults. Am J Respir Crit Care Med 2014;190:1437-46. [Crossref] [PubMed]
- Zeerleder S, Caliezi C, van Mierlo G, et al. Administration of C1 Inhibitor Reduces Neutrophil Activation in Patients with Sepsis. Clin Diagn Lab Immunol 2003;10:529-35. [PubMed]
- Kim DD, Song WC. Membrane complement regulatory proteins. Clin Immunol 2006;118:127-36. [Crossref] [PubMed]
- Mohammadi B, Schedel I, Graf K, et al. Role of endotoxin in the pathogenesis of critical illness polyneuropathy. J Neurol 2008;255:265-72. [Crossref] [PubMed]
- Oberholzer A, Oberholzer C, Moldawer LL. Sepsis syndromes: understanding the role of innate and acquired immunity. Shock 2001;16:83-96. [Crossref] [PubMed]
- Sharshar T, Citerio G, Andrews PJ, et al. Neurological examination of critically ill patients: a pragmatic approach. Report of an ESICM expert panel. Intensive Care Med 2014;40:484-95. [Crossref] [PubMed]
- Latronico N, Bertolini G, Guarneri B, et al. Simplified electrophysiological evaluation of peripheral nerves in critically ill patients: the Italian multi-centre CRIMYNE study. Crit Care 2007;11:R11. [Crossref] [PubMed]
- Novak KR, Nardelli P, Cope TC, et al. Inactivation of sodium channels underlies reversible neuropathy during critical illness in rats. J Clin Invest 2009;119:1150-8. [Crossref] [PubMed]