This article has an erratum available at: http://dx.doi.org/10.21037/atm-2024-10 the article has been update on 2024-08-06 at here.
Prostaglandin F2α protects against pericyte apoptosis by inhibiting the PI3K/Akt/GSK3β/β-catenin signaling pathway
Introduction
As a common microvascular complication of diabetes, diabetic retinopathy (DR) is a prominent contributor to adult blindness in many industrialized nations (1). As many as 1 in 3 patients with diabetes develop DR, and it is associated with a heightened risk of life-threatening systemic vascular diabetic complications, such as coronary heart disease, stroke, and heart failure (2). In approximately 1/3 of cases, DR progresses to severe retinopathy or macular edema, which threatens the individual’s sight (3).
The onset characteristics of DR include basement membrane thickening, interendothelial tight junction loss, and selective loss of pericytes, subsequently leading to increased vascular permeability (4). Endothelial cells are wrapped by pericytes, which are an important component of retinal microvessels (5). Pericytes maintain the integrity of the inner blood-retinal barrier (BRB) through their regulatory involvement in microvascular functions (6,7). Their loss weakens the inner BRB, and aggravates vascular leakage and DR.
Apoptosis is central to DR development. Pericyte apoptosis directly triggered by hyperglycemia, which subsequently induces capillary occlusions and microaneurysms of retinal capillaries (8), has been shown be an important mechanism in early DR (9). Therefore, targeted elimination of pericyte apoptosis could be beneficial for primary prevention of DR.
Many studies have reported the favorable effects of polyunsaturated fatty acids and related metabolites on pathological processes in multiple diseases, including diabetes and its complications (10). Our previous study also demonstrated that prostaglandin F2α (PGF2α), the cyclooxygenase metabolites of arachidonic acid could protect against DR by regulating the mobility of pericytes through the prostaglandin F receptor (FP receptor) /RhoA pathway (11). However, the effect of PGF2α on pericytes apoptosis has yet to be considered. Therefore, the present work was designed to investigate the effects of PGF2α on pericyte apoptosis during early DR progression.
We present the following article in accordance with the ARRIVE reporting checklist (available at https://dx.doi.org/10.21037/atm-21-2717).
Methods
In vivo models
For the present research, we constructed 2 animal models: a high-fat diet (HFD) and streptozotocin (STZ)-induced type 2 diabetic mouse model and a spontaneous type 2 diabetic db/db mouse model. Beijing HFK Bioscience Co., Ltd. (Beijing, China) supplied all mice used in this study. The mice were housed at 24±2 °C, with free access to chow and water. Animal experiments were performed under the approval of Tianjin Medical University Experimental Animal Ethics Committee (No.2020018), in compliance with institutional guidelines for the care and use of animals. A protocol was prepared before the study without registration.
For the HFD/STZ diabetic mouse model, C57BL/6 mice (n=6) were fed an HFD for 8 weeks, after which they were given intraperitoneal injections of STZ (30 mg/kg; Sigma, MO, USA) with citrate buffer daily for 1 week; the control group (n=6) received equal volumes of citrate buffer. A random blood glucose level >16.7 mM was taken to indicate diabetes. The spontaneous diabetes model was established in male C57BL/KsJ-db/db mice without the leptin receptor (n=6); the non-diabetic control group comprised wild-type littermate db/m mice (n=6).
Cell culture
Human retinal pericytes (HRMVPCs, C1133) were obtained from WHELAB Bioscience (Shanghai, China). Cell culture was performed with low-glucose Dulbecco’s Modified Eagle Medium (DMEM) (HyClone, USA) supplemented with 10% fetal bovine serum (Gibco, USA) in a humidified 5% CO2 atmosphere at 37 °C. We synchronized all cells by incubating them without serum for 8 hours, after which we divided them into the following treatment groups: (I) normal-glucose (NG) group: incubation in DMEM containing 5.5 mM glucose; (II) high-glucose (HG) group: incubation in DMEM containing 33.3 mM glucose; (III) PGF2α (HG+PGF2α) group: 48-hour incubation in DMEM containing 33.3 mM glucose and 100 nM PGF2α (Cayman Chemical Co, Ann Arbor, MI); and (IV) AL8810 and PGF2α (HG + PGF2α + AL8810) group: 48-hour incubation in DMEM containing 33.3 mM glucose, 100 nM PGF2α, and 10 µM AL8810.
Hematoxylin and eosin staining
Eyeballs were fixed in 10% formalin, embedded in paraffin, and cut into 4-µm sections. The sections were then deparaffinized, and stained with hematoxylin and eosin (H&E). Finally, a light microscope was employed to observe retinal thickness.
Retinal trypsin digestion
Mouse retinas were trypsin-digested using a method set out in a previous study (12). Briefly, eyeballs were fixed in 10% formalin for 48 hours. The retinas were isolated and washed overnight, and then subjected to digestion in 3% trypsin (Amresco 1:250; PA, USA) for 120 minutes at 37 °C. After that, the vasculature was gently separated and stained with periodic acid-Schiff (PAS). Light microscope was used to observe acellular vessels and pericytes in 6 randomly selected fields for each retina.
TUNEL staining
A TUNEL [terminal deoxynucleotidyl transferase (TdT) dUTP nick-end labeling ] kit (biotin-labeled POD and KGA7072, KeyGEN Biotech, Nanjing, China) was used to detect apoptotic cells in adherence with the protocol supplied by the manufacturer. In brief, paraffin-embedded tissue sections were subjected to deparaffinization, permeabilization in proteinase K, and blocking in 3% hydrogen peroxide, after which they were linked with TdT enzyme and labeled with streptavidin-horseradish peroxidase (HRP). Finally, the sections were stained using a DAB (3,3'-diaminobenzidine) kit.
In vitro, pericytes were seeded and grown to 85% confluence on a 24-well plate, and treated with high glucose or PGF2a for 24 hours. For each section or slide, fluorescence microscopy was used to count the number of cells stained positively for TUNEL.
Western blot analysis
The extraction of proteins from retinal tissues or pericytes was performed using radioimmunoprecipitation lysis buffer containing phenylmethylsulfonyl fluoride, phosphatase inhibitors, and loading buffer. Protein was loaded onto SDS-PAGE gel in equal quantities before being transferred to nitrocellulose filter membranes. After 1 hour of blocking in milk, the membranes were subjected to overnight incubation at 4 °C in the presence of primary antibodies against Bax (1:500, CST, #2772 MA, USA), Bcl-2 (1:1,000, CST, #3498), cleaved caspase 3 (1:1,000, ABclonal, #A2156), t-Akt (1:2,000, CST, #9272S,), p-Akt (1:2,000, CST, #4060S,), t-GSK-3β (1:1,000, Bioworld, #BS4084,), p-GSK-3β (1:1,000, Bioworld, #BS4084), anti-active-β-catenin (1:1,000, CST, #8814) and GAPDH (1:5,000, Bioworld, #AP0063). Subsequently, HRP-conjugated secondary antibodies were added, after which the membranes were left to react. ECL reagents (Advansta, CA, USA) were used for chemiluminescence signal detection. The protein bands were densitometrically analyzed using ImageJ (NIH image software), with normalization to relevant controls.
Statistical analyses
Data obtained from our experiments were analyzed with GraphPad Prism 8.0 (La Jolla, CA, USA), and are presented as means ± standard deviation. The unpaired Student’s t-test was adopted for comparison of differences between 2 groups, while significant differences among multiple groups were determined by one-way analysis of variance. Statistical significance was determined at P<0.05.
Results
The numbers of capillary pericytes are reduced in diabetic mouse retinas
To explore the effects of hyperglycemia, we used 2 in vivo models: an HFD/STZ mouse model (with C57BL/6 mice as normal controls), and a spontaneous diabetic db/db mouse model (with wild-type litter made db/m mice as controls). First, tissues harvested from the mice were stained with H&E. With a view to improving comparability, each of the tissue samples was cut at the same distance from the optic nerve head.
The HE staining of retinal showed that compared with the normal groups, the retinal thickness of each layer became thinner in the diabetic groups (HFD/STZ and db/db groups) (Figure 1A,B). Next, we performed retinal trypsin digestion and observed the numbers of pericytes and acellular capillaries in the 2 animal models. Retinal capillary pericytes showed a marked decrease in number in diabetic mice (the HFD/STZ and db/db groups) compared with the controls, while the opposite result was observed with respect to retinal acellular capillaries (Figure 1C,D). Semiquantitative analysis verified these results (Figure 1E,F,G,H), suggesting that early-onset pericyte loss had occurred in the diabetic mouse models.
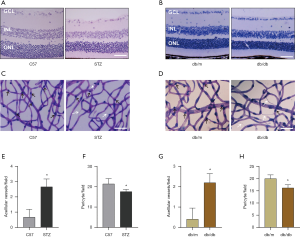
Hyperglycemia increases pericyte apoptosis in vivo and in vitro
Since apoptosis is the major pathological process in DR, we detected pericyte apoptosis in retinal tissues from diabetic mice by performing a TUNEL assay. Compared with those from the normal controls, the retinal tissues of the diabetic mice (the HFD/STZ and db/db groups) displayed marked increases in the proportions of apoptotic cells (Figure 2A,B). Western blotting further uncovered elevation of the protein expression of cleaved caspase 3 and the BAX/Bcl-2 ratio in the diabetic groups (Figure 2C,D).
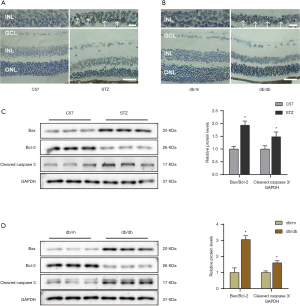
In vitro, TUNEL staining also showed that 48-hour exposure to HG treatment (33.3 mM) led to a dramatically increased apoptosis in pericytes (Figure 3A). Semiquantitative analysis verified this finding (Figure 3B). Further examination of apoptotic biomarkers of apoptosis revealed the protein expression of cleaved caspase 3 and the BAX/Bcl-2 ratio to also be increased after HG treatment (Figure 3C,D).
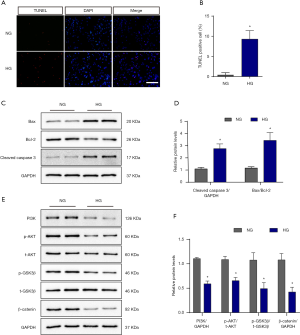
Hyperglycemia inhibits the PI3K/Akt/GSK3β/β-catenin pathway
To uncover the mechanism of pericyte apoptosis, we assessed the PI3K/Akt/GSK3β/β-catenin signaling pathway after HG treatment. Our results showed reductions in PI3K, p-Akt, p-GSK-3β, and active β-catenin protein expression (Figure 3E). This result was confirmed by semiquantitative analysis (Figure 3F).
PGF2α reverses apoptosis of pericytes
Since PGF2α has been reported to exert its physiological effects mainly through its specific G protein-coupled receptor-FP receptor (prostaglandin F receptor, FP receptor) (13), we investigated its protective effect on pericyte apoptosis using AL8810, a selective FP-receptor antagonist. Our results showed that PGF2α could reverse the increase in TUNEL-positive pericytes after 48 hours of HG treatment, while AL8810 blocked this protective effect (Figure 4A). Semiquantitative analysis verified these findings (Figure 4B). Western blot further showed that PGF2α could inhibit apoptotic protein expression, such as that of cleaved caspase 3 and BAX/Bcl-2. while ALL80 inhibited these PGF2α-induced anti-apoptotic effects (Figure 4C,D).
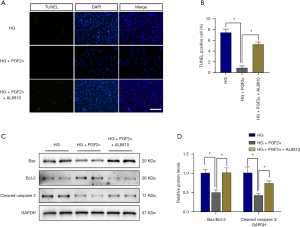
PGF2α reverses pericyte apoptosis via the PI3K/Akt/GSK3β/β-catenin pathway
For further mechanistic examination of PGF2α’s inhibitive effect on pericyte apoptosis, we detected the expression of PI3K/Akt/GSK3β/β-catenin pathway-related proteins. We found that PGF2α elevated the protein expression of p-Akt, p-GSK-3β, and active β-catenin, and AL880 blocked this effect (Figure 5A,B,C,D,E). All of these results implied that PGF2α reverses pericyte apoptosis through the PI3k/Akt/GSK3β/β-catenin pathway.
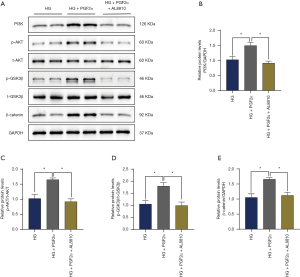
Discussion
Diabetes refers to a metabolic disorder resulting from deficiencies in insulin secretion or resistance. Patients with this disease characteristically exhibit elevated blood sugar levels. DR is a frequently occurring microvascular complication in diabetic patients. It is characterized by changes in the morphology of microvessels, basement membrane thickening, loss of endothelial tight junctions, and loss of pericytes, as well as vascular leakage and abnormal angiogenesis (14). In particular, pericyte loss has been identified as the earliest change in the diabetic retina. In both animal models in the present study, we observed pericyte loss and increased levels of apoptosis in diabetic mouse retinas.
Polyunsaturated fatty acids, especially arachidonic acid, eicosapentaenoic acid, and docosahexaenoic acid, have extremely high retinal expression levels. Research has shown that polyunsaturated fatty acids and their metabolites can regulate many biological processes, including diabetes and its complications (10,15). For instance, Sapieha et al. revealed that ω-3 polyunsaturated fatty acids can inhibit angiogenesis in oxygen-induced retinopathy (16).
PGF2α is a metabolite of arachidonic acid through the cyclooxygenase-2 pathway. Arachidonic acid has been reported to be found at low concentrations in the retinal blood vessels of DR patients (17). Douros et al. revealed that PGE2 and PGF2α levels in the vitreous of patients with proliferative diabetic retinopathy (PDR) are reduced (18). In patients with diabetes, the levels of these prostaglandins decrease with the concentration of arachidonic acid precursors in the retina. In addition, studies have found that the retinal content of PGE2 in rats with STZ-induced diabetes is significantly lower than that in non-diabetic rats (19). Our previous research also showed that a marked decrease in PGF2α expression occurred in mice with non-proliferative DR in comparison to mice without DR, and that PGF2α exerts a protective effect against DR by regulating the mobility of pericytes via the FP receptor/RhoA pathway (11). However, the apoptotic effects of PGF2α on pericytes had not been reported. In this study, we found that PGF2α can improve pericyte apoptosis and this effect can be reversed by its specific receptor antagonist AL8810.
Next, we explored the mechanism by which PGF2α reduces pericyte apoptosis. The PI3K-Akt pathway is related to many important physiological processes such as cell proliferation, differentiation, and apoptosis (20,21). The activation of AKT phosphorylation can phosphorylate GSK-3β, resulting in its inactivation. GSK-3β, a protein kinase, can induce the phosphorylation of downstream β-catenin and deactivate it (22). Studies have shown that activation of the PI3K/Akt signaling pathway can inhibit cardiomyocyte and fibroblasts apoptosis (23,24). PGF2α is related to the proliferation of cardiomyocytes and vascular smooth muscle cells (25). Moreover, PGF2α binds to the FP receptor to promote cell proliferation and participates in the angiogenesis of embryos during pregnancy (26). Our results show that PGF2α can activate the PI3K/Akt/GSK3β/β-catenin pathway and improve pericyte apoptosis and this effect can be reversed by AL8810 treatment.
In conclusion, our results demonstrate that HG treatment can increase the apoptosis of pericytes, while PGF2α treatment can protect against pericyte apoptosis by activating the PI3K/Akt/GSK3β/β-catenin pathway, and its effects can be reversed by its specific receptor AL8810.
Acknowledgments
Funding: This work was supported by grants from the Natural Science Foundation of Tianjin (grant No. 18JCZDJC35500), the National Natural Science Foundation of China (grant No. 81970697), the National Key Research and Development Program of China (grant Nos. 2018YFC1314001 and 2019YFA0802503), and Tianjin Research Innovation Project for Postgraduate Students (grant No. 2020YJSB168).
Footnote
Reporting Checklist: The authors have completed the ARRIVE reporting checklist. Available at https://dx.doi.org/10.21037/atm-21-2717
Data Sharing Statement: Available at https://dx.doi.org/10.21037/atm-21-2717
Conflicts of Interest: All authors have completed the ICMJE uniform disclosure form (available at https://dx.doi.org/10.21037/atm-21-2717). The authors have no conflicts of interest to declare.
Ethical Statement: The authors are accountable for all aspects of the work in ensuring that questions related to the accuracy or integrity of any part of the work are appropriately investigated and resolved. Animal experiments were performed under the approval of Tianjin Medical University Experimental Animal Ethics Committee (No.2020018), in compliance with institutional guidelines for the care and use of animals.
Open Access Statement: This is an Open Access article distributed in accordance with the Creative Commons Attribution-NonCommercial-NoDerivs 4.0 International License (CC BY-NC-ND 4.0), which permits the non-commercial replication and distribution of the article with the strict proviso that no changes or edits are made and the original work is properly cited (including links to both the formal publication through the relevant DOI and the license). See: https://creativecommons.org/licenses/by-nc-nd/4.0/.
References
- Fong DS, Aiello LP, Ferris FL 3rd, et al. Diabetic retinopathy. Diabetes Care 2004;27:2540-53. [Crossref] [PubMed]
- Cheung N, Mitchell P, Wong TY. Diabetic retinopathy. Lancet 2010;376:124-36. [Crossref] [PubMed]
- Saaddine JB, Honeycutt AA, Narayan KM, et al. Projection of diabetic retinopathy and other major eye diseases among people with diabetes mellitus: United States, 2005-2050. Arch Ophthalmol 2008;126:1740-7. [Crossref] [PubMed]
- Beltramo E, Porta M. Pericyte loss in diabetic retinopathy: mechanisms and consequences. Curr Med Chem 2013;20:3218-25. [Crossref] [PubMed]
- Sweeney MD, Ayyadurai S, Zlokovic BV. Pericytes of the neurovascular unit: key functions and signaling pathways. Nat Neurosci 2016;19:771-83. [Crossref] [PubMed]
- Armulik A, Genové G, Betsholtz C. Pericytes: developmental, physiological, and pathological perspectives, problems, and promises. Dev Cell 2011;21:193-215. [Crossref] [PubMed]
- Bergers G, Song S. The role of pericytes in blood-vessel formation and maintenance. Neuro Oncol 2005;7:452-64. [Crossref] [PubMed]
- Cogan DG, Toussaint D, Kuwabara T. Retinal vascular patterns. IV. Diabetic retinopathy. Arch Ophthalmol 1961;66:366-78. [Crossref] [PubMed]
- Li W, Yanoff M, Liu X, et al. Retinal capillary pericyte apoptosis in early human diabetic retinopathy. Chin Med J (Engl) 1997;110:659-63. [PubMed]
- Chen L, Cheng CY, Choi H, et al. Plasma Metabonomic Profiling of Diabetic Retinopathy. Diabetes 2016;65:1099-108. [Crossref] [PubMed]
- Peng L, Sun B, Liu M, et al. Plasma metabolic profile reveals PGF2α protecting against non-proliferative diabetic retinopathy in patients with type 2 diabetes. Biochem Biophys Res Commun 2018;496:1276-83. [Crossref] [PubMed]
- Chou JC, Rollins SD, Fawzi AA. Trypsin digest protocol to analyze the retinal vasculature of a mouse model. J Vis Exp 2013;e50489 [Crossref] [PubMed]
- Pierce KL, Fujino H, Srinivasan D, et al. Activation of FP prostanoid receptor isoforms leads to Rho-mediated changes in cell morphology and in the cell cytoskeleton. J Biol Chem 1999;274:35944-9. [Crossref] [PubMed]
- Lechner J, O'Leary OE, Stitt AW. The pathology associated with diabetic retinopathy. Vision Res 2017;139:7-14. [Crossref] [PubMed]
- Zhang X, Yang N, Ai D, et al. Systematic metabolomic analysis of eicosanoids after omega-3 polyunsaturated fatty acid supplementation by a highly specific liquid chromatography-tandem mass spectrometry-based method. J Proteome Res 2015;14:1843-53. [Crossref] [PubMed]
- Sapieha P, Stahl A, Chen J, et al. 5-Lipoxygenase metabolite 4-HDHA is a mediator of the antiangiogenic effect of ω-3 polyunsaturated fatty acids. Sci Transl Med 2011;3:69ra12 [Crossref] [PubMed]
- Futterman S, Kupfer C. The fatty acid composition of the retinal vasculature of normal and diabetic human eyes. Invest Ophthalmol 1968;7:105-8. [PubMed]
- Douros S, Phillips BA, Nadel A, et al. Human vitreal prostaglandin levels and proliferative diabetic retinopathy. Doc Ophthalmol 2001;103:27-34. [Crossref] [PubMed]
- Naveh-Floman N, Weissman C, Belkin M. Arachidonic acid metabolism by retinas of rats with streptozotocin-induced diabetes. Curr Eye Res 1984;3:1135-9. [Crossref] [PubMed]
- Zhang E, Gao B, Yang L, et al. Notoginsenoside Ft1 Promotes Fibroblast Proliferation via PI3K/Akt/mTOR Signaling Pathway and Benefits Wound Healing in Genetically Diabetic Mice. J Pharmacol Exp Ther 2016;356:324-32. [Crossref] [PubMed]
- Wang X, Pan J, Liu D, et al. Nicorandil alleviates apoptosis in diabetic cardiomyopathy through PI3K/Akt pathway. J Cell Mol Med 2019;23:5349-59. [Crossref] [PubMed]
- McCubrey JA, Steelman LS, Bertrand FE, et al. Multifaceted roles of GSK-3 and Wnt/β-catenin in hematopoiesis and leukemogenesis: opportunities for therapeutic intervention. Leukemia 2014;28:15-33. [Crossref] [PubMed]
- Dai J, Sun Y, Chen DY, et al. Negative regulation of PI3K/AKT/mTOR axis regulates fibroblast proliferation, apoptosis and autophagy play a vital role in triptolide-induced epidural fibrosis reduction. Eur J Pharmacol 2019;864:172724 [Crossref] [PubMed]
- Yao H, Han XY, Han XZ. The cardioprotection of the insulin-mediated PI3K/Akt/mTOR signaling pathway2. Am J Cardiovasc Drugs 2014;14:433-42. [Crossref] [PubMed]
- Goupil E, Fillion D, Clément S, et al. Angiotensin II type I and prostaglandin F2α receptors cooperatively modulate signaling in vascular smooth muscle cells. J Biol Chem 2015;290:3137-48. [Crossref] [PubMed]
- Kaczynski P, Kowalewski MP, Waclawik A. Prostaglandin F2α promotes angiogenesis and embryo-maternal interactions during implantation. Reproduction 2016;151:539-52. [Crossref] [PubMed]
(English Language Editor: J. Reynolds)